ABSTRACT
Liquid-crystalline dimers and bimesogens have attracted much attention due to their propensity to exhibit the spontaneously chiral twist-bend mesophase (NTB), most often by dimers with methylene spacers. Despite their relative ease of synthesis, the number of ether-linked twist-bend materials significantly lags behind those of methylene-linked compounds. In this work, we have prepared and studied a range of ether-linked bimesogens homologous in structure to the FFO9OCB; as with methylene-linked systems, it appears that it is molecular topology and the gross molecular shape that are the primary drivers for the formation of this phase of matter. Dimers and bimesogens are well studied within the context of the twist-bend phase; however, present understanding of this mesophase in oligomeric systems lags far behind. We report our recent efforts to prepare further examples of oligomeric twist-bend nematogens, including further examples of our ‘n+1’ methodology, which may allow the synthesis of high-purity, monodisperse materials of any given length to be prepared. We have observed that there is a tendency for these materials to exhibit highly ordered soft-crystalline mesophases as opposed to the twist-bend phase.
GRAPHICAL ABSTRACT
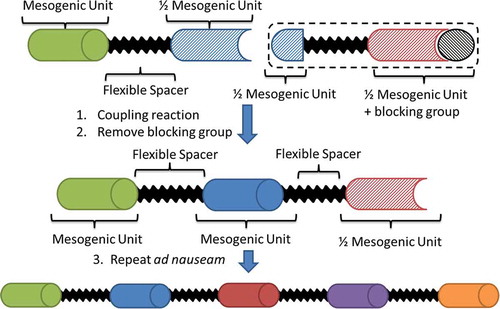
Introduction
Presently, the majority of known low-molar-mass liquid crystals have calamitic (rod-like) molecular structures. Joining two identical rod-like units together affords a dimer, three units afford a trimer and n units afford an oligomer [Citation1–Citation4]. However, if we join together n mesogenic units that are not identical, such as rod-disc systems [Citation5], then it is preferable to use the terms bimesogen (n = 2), trimesogen (n = 3), tetramesogen (n = 4) and oligomesogen. There has been something of resurgence in interest in liquid crystal dimers in recent years, due largely to the observation of thermodynamically stable twist-bend phases in these systems [Citation6–Citation38]. This has motivated the synthesis of a number of novel compounds, and present experimental results indicate that the twist-bend phase is primarily driven by topology [Citation39], with the thermal stability having a dependency on the intermesogen angle [Citation40,Citation41]. Several aspects of the NTB phase have been reviewed recently [Citation28,Citation32,Citation42,Citation43]. It has been demonstrated for materials with nonamethylene and heptamethylene central spacers that there is a linear relationship between TNTB−N and TN-Iso [Citation39]. It has been observed that many functional groups – including the SF5 group – can be incorporated into the structure of a suitable dimer without loss of the NTB phase. Last, the twist-bend nematic phase – in dimers and bimesogens at least – is found mostly for methylene-linked materials, although a number of other linking groups are known to yield materials that afford this mesophase [Citation7,Citation21,Citation22,Citation33,Citation44–Citation49]. In the first part of this paper, we present the latest part of our study into the structure–property relationships of bis ether-linked dimers and bimesogens that exhibit the twist-bend phase.
Following the identification of the NTB phase in dimers [Citation10], and bent-core materials [Citation50], Jansze et al. reported in 2014 on a novel hydrogen-bonded liquid-crystalline trimer that also exhibited the twist-bend nematic phase [Citation51]. This material, known as CB5OBA, has an odd-parity spacer unit – the homologous even-parity material does not exhibit the NTB phase, mirroring trends seen for dimers and bimesogens. Shortly after this Wang et al. reported a hybrid calamitic/bent-core trimesogen that exhibited the NTB phase [Citation52]. This material has two cyanobiphenyl mesogenic units appended to a central resorcinol-derived bent-core-type mesogenic unit. In 2015, a methylene-linked tetramesogen (T49, ) was reported by us group; this material exhibits enantiotropic nematic and twist-bend nematic mesophases [Citation53], as does the related linear trimesogen (T39, [Citation54]. Both T39 and T49 are prepared in the same manner; an intermediate with one complete mesogenic unit and one ‘half-complete’ mesogenic unit bearing a phenol is esterified with an appropriate dicarboxylic acid to yield a trimer/tetramer.
Figure 1. The molecular structures and transition temperatures (°C) of the trimer T39 (top) and the tetramer T49 (bottom) [Citation53,Citation54].
![Figure 1. The molecular structures and transition temperatures (°C) of the trimer T39 (top) and the tetramer T49 (bottom) [Citation53,Citation54].](/cms/asset/064582dd-d88a-4fa8-bec3-7512ede9fa3e/tlct_a_1343500_f0001_b.gif)
Figure 2. Truncated synthetic pathway to O47 (n = 2) and O67 (n = 4) via the use of a phenolic intermediate bearing a masked carboxylic acid [Citation55]. Transition temperatures are given in °C, and the related dimer ‘O27’ is given for comparison [Citation56].
![Figure 2. Truncated synthetic pathway to O47 (n = 2) and O67 (n = 4) via the use of a phenolic intermediate bearing a masked carboxylic acid [Citation55]. Transition temperatures are given in °C, and the related dimer ‘O27’ is given for comparison [Citation56].](/cms/asset/6b6602cb-b43c-4ff7-9774-0addac695bfd/tlct_a_1343500_f0002_b.gif)
Unfortunately, this method is ill suited to the preparation of higher oligomesogens due to the lack of available dicarboxylic acids that will yield pentamesogens, hexamesogens, etc. The aspiration to prepare higher oligomesogens than tetramesogens was later realised using chemistry inspired by a simple polyester condensation; a chemical intermediate (prepared in three steps from 4-carboxybenzaldehyde) was devised (), which bears a free phenol and a carboxylic acid masked with a benzyl group. The phenol is esterified with a suitable carboxylic acid; hydrogenolysis liberates the carboxylic acid group, which is then free to partake in another esterification reaction. Finally, esterification of the free acid with a suitable bis (4-hydroxyphenyl) alkane affords the symmetrical dimers. To date this has been used to prepare a tetramesogen (O47) and a hexamesogen (O67), with the synthesis of higher oligomers currently ongoing; both O47 and O67 exhibit nematic and twist-bend mesophases.
This approach is not without drawbacks; the use of bis phenyl terephthalate mesogenic units is especially problematic due to transesterification. We therefore sought to prepare materials with biphenyl mesogenic units which for obvious reasons do not undergo this unwanted side reaction, if possible avoiding the obvious and laborious lithiation/borylation/cross-coupling stratagem. In the second part of this paper, we present a novel synthesis of liquid-crystalline oligomers with mixed ether/methylene-linking groups using a derivative of the ‘n+1’ methodology used by us to prepare the hexamesogen O67 [Citation55].
Experimental
Materials were characterised by nuclear magnetic resonance (NMR) (1H, 13C{1H} and where appropriate 11B{1H}, 19F), mass spectrometry and Fourier-transform infrared (FT-IR), with purity of dimers assessed by either combustion analysis and reverse-phase high performance liquid chromatography (HPLC). Computational chemistry was performed in Gaussian G09 revision e.01 [Citation57]. The experimental set-up used for X-ray scattering experiments has been described previously [Citation58,Citation59]. Details of chemical synthesis and characterisation are given in the supplementary information to this article. Compounds were synthesised as depicted in -.
Results and discussion
The unsymmetrical bimesogens were prepared via one of the two methods, either the Williamson etherification of 4-(9-bromononyloxy)-4′-cyanobiphenyl with an appropriate phenol, or the Mitsunobu etherification of 4-(9-hydroxynonyloxy)-4′-cyanobiphenyl with an appropriate phenol as shown in . Both 4-(9-bromononyloxy)-4′-cyanobiphenyl and 4-(9-hydroxynonyloxy)-4′-cyanobiphenyl were available in house, having been prepared previously [Citation60]. Most phenols were available in house; however, 4-hydroxy-4′-nitrobiphenyl was prepared as described previously [Citation61], and 4-fluoro-4′-hydroxybiphenyl was purchased from TCI.
The liquid-crystalline properties of all materials were analysed by a combination of polarised optical microscopy (POM) and differential scanning calorimetry (DSC) (). Of the compounds presented in , both 1 (CBO9OCB) and 8 (FFO9OCB) are already known in the literature; the former exhibits a nematic phase whereas the latter exhibits an additional monotropic twist-bend (NTB) phase [Citation48]. Knowing that replacement of one of the 4-cyanobiphenyl mesogenic units of 1 with the 2′-4-difluorobiphenyl unit leads to the formation of the twist-bend phase serves as a starting point for our present study into structure–property relationships.
Table 1. Transition temperatures (°C) and associated enthalpies of transition (kJ mol−1) for 1–13 as determined using a Metter Toledo DSC822e at a heat/cool rate of 10°C min−1. Phase identification was made by POM as described in the text.
Compound 2, in which a fluorine atom has been positioned ortho to the nitrile group of one mesogenic unit, exhibits a monotropic twist-bend phase and, relative to the parent material, reduced melting and clearing points. Compound 3 is an isomer of 2 in which the position of the fluorine atom has been varied, and although this leads to a change in the melting point both the clearing point and N-NTB transition temperatures are virtually unchanged. For compound 4 a nitro group has replaced the cyano group of one mesogenic unit; this leads to a reduced melting point; however, both the N-I and NTB-N transition temperatures are comparable to those of 2 and 3. Replacement of a single nitrile with a fluoro-group affords compound 5; this leads to significantly lower melting and clearing points than 1, whereas the NTB-N transition temperature is around 25°C lower than for 2–4. Although the N-I phase transition was invariably found to be first order the NTB-N transition was only weakly first order, with the associated enthalpies of transition being significantly smaller for the latter than the former. Compound 8 was reported previously to exhibit both nematic and NTB mesophases; we opted to resynthesise it to use as a standard for comparative purposes. The transition temperatures we obtain are essentially identical, albeit with a marginally higher melting point, to those reported previously (Cr 72.5 NTB 49.9 N 112.4 Iso). When studied by DSC, the material was invariably found to recrystallise prior to the N-NTB transition (see thermogram in ). However, it was possible to observe by microscopy that the transition occurs around 50°C. Similarly, for compound 9 we observed the material readily crystallised during DSC study, but it was possible to obtain a reproducible temperature value of 45°C for the NTB-N transition by microscopy, although this often occurred simultaneously with crystallisation.
Figure 3. (Colour online) DSC thermogram for compound 8 (FFO9OCB) obtained at 20°C min−1 with an expansion showing the region between 20°C and 60°C during which the sample recrystallises; however, with rapid uncontrolled cooling we can observe a NTB-N transition occurring simultaneously with crystallisation at around 50°C.
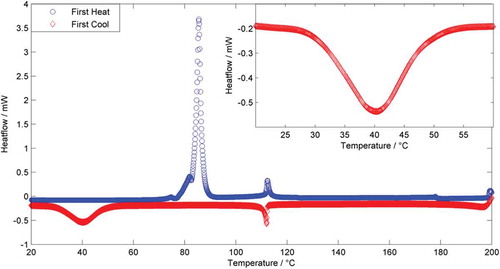
The incorporation of a third fluorine atom into the structure of 6 leads to compound 10; this material has a comparable melting point to the parent. However, the clearing point is dramatically reduced and the twist-bend phase is not observed because even with rapid cooling the sample crystallises directly from the nematic phase. Representative photomicrographs are given in .
Figure 4. (Colour online) Photomicrographs (100×, crossed polarisers) of (a) the schlieren texture of the nematic phase of 7 at 71°C, (b) the same region showing the blocky texture of the twist-bend phase of 7 at 56°C and (c) the nematic phase of 8 (FFO9OCB) at 56°C, cooled at 10°C min−1 showing crystallisation of the sample when not rapidly cooled.
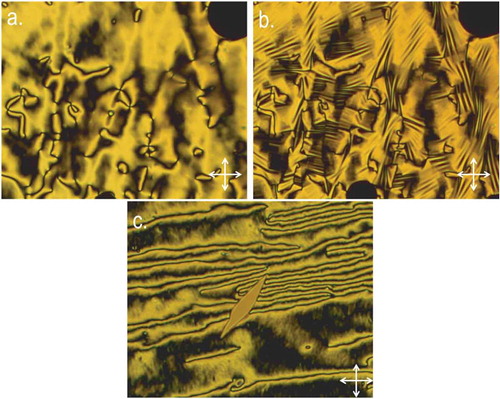
Thus, we opted to prepare a material incorporating the 2,3-difluorobiphenylcyclohexyl (DFBC) unit employed in the first ether-linked bimesogen to exhibit the NTB phase, FBO11ODFCB3 [Citation62]. We have found previously that dimers and bimesogens incorporating the DFBC unit have a strong tendency to exhibit the twist-bend nematic phase [Citation63]. Increasing the aspect ratio of the mesogenic units of a dimesogen/bimesogen has been demonstrated previously to confer large increases to both the clearing point and, if present, the NTB-N transition temperature [Citation31,Citation39,Citation64,Citation65], and with this in mind, we prepared the two mixed biphenyl/terphenyl bimesogens 12 and 13. Compounds 11, 12 and 13 were found to exhibit enantiotropic nematic and NTB mesophases. Representative photomicrographs are given in . In the case of the biphenyl/terphenyl bimesogens 12 and 13 the clearing points are significantly higher than the analogous biphenyl/biphenyl dimers 1 and 2. The thermal behaviour of compound 11, which has a nonamethylene spacer and a pentyl terminal chain, is remarkably close to that of the homologous dimer reported by us previously with an undecamethylene spacer and a propyl terminal chain (Cr 83.5 NTB 83.3 N 190.1 Iso) [Citation63].
Figure 5. (Colour online) Photomicrographs (100×, crossed polarisers) of (a) the schlieren texture of the nematic phase of 11 at 180°C, (b) various optical textures of the NTB phase at 75.8°C, (c) the same region with a ¼ waveplate inserted, (d) the schlieren texture of the nematic phase of 13 confined in a 9 μm cell with surfaces treated for homeotropic alignment and (e) the same region of 13 cooled into the NTB phase at 121°C.
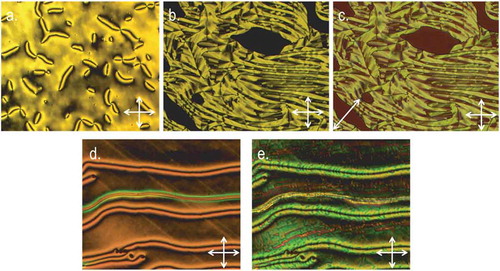
The ability of ether-linked dimers and bimesogens to exhibit the twist-bend nematic phase has once again been firmly demonstrated. We next turned our attention to incorporating ether linkages, as well as either alkyne or methylene linkers, into tetramesogenic materials with a view to obtaining further examples of oligomeric twist-bend materials. The two hydroxyl-terminated alkoxycyanobiphenyls (i1 and i2) used in were prepared as described previously [Citation58], and the preparation of 1,9-bis(4-bromophenyl)nona-1,8-diyne (i6) was reported in a previous publication [Citation40]. Palladium on carbon (5%) poisoned with diaminoethane (denoted as Pd/C{En} in the text) was prepared by us previously [Citation40,Citation66].
As shown in , the Mitsunobu etherification of i1 and i2 with the pinacol ester of 4-hydroxyphenyl boronic acid (i3) afforded i4 and i5. The Suzuki coupling of both i4 and i5 with i6 proceeded smoothly to afford the tetramesogens 14 and 15, respectively. Using biphenyl mesogenic units rather than the phenylbenzoate or bis teraphthaloyl benzoate effectively eliminates the problem of transesterification. The hydrogenation of 1 and 2 with palladium on carbon poisoned with diaminoethane afforded the tetramesogens 3 and 4, respectively. The reaction proceeded smoothly without hydrodefluorination or reduction of the nitrile to a benzylamine and was complete within 2 h in all cases. The products (16/17) and the starting materials (14/15) could be easily distinguished by 1H NMR spectroscopy due to the difference in chemical shift of a CH2 adjacent to an aromatic ring versus a CH2 adjacent to an alkyne, as well as differences in two of the aromatic proton environments as shown in . Furthermore, workup of the hydrogenation was trivial and chromatography free; the reaction mass was filtered through a packed bed of celite, dried in vacuo, redissolved in DCM onto which ethanol was layered, affording the tetramesogens 16 and 17 as microcrystalline solids.
Figure 6. (Colour online) Overlaid 1H NMR spectra (400 MHz, CDCl3) of compounds 14 (red) and 16 (blue) showing the change in multiplicity in the aromatic region ((a) 6.8–7.8 ppm) and in the chemical shift in the ‘aliphatic’ region ((b) 2.3–2.7 ppm).
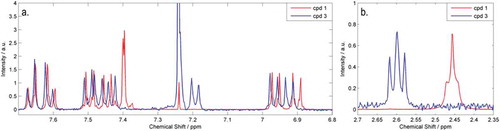
The liquid-crystalline behaviour of the tetramesogens 14–17 was determined using a combination of POM and DSC. Given the high viscosity of these materials, even in the isotropic liquid, we were unable to load the samples into the capillary tubes required for small angle X-ray scattering (SAXS) analysis, and so the phase identification was made solely on optical microscopy. Representative photomicrographs are presented in .
Figure 7. (Colour online) Photomicrographs (100×) of compound 16 on an untreated glass slide in (a) the nematic phase at 175°C, (b) the smectic C phase at 165 °C and (c) the unknown ‘X’ phase at 135°C. All photomicrographs are of approximately the same region of the sample.
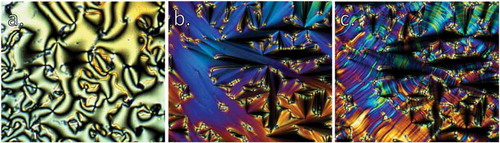
As shown in all three tetrameric materials exhibited smectic C and X phases, with 15, 16 and 17 exhibiting additional nematic phases. We were surprised that compounds 14–17 did not exhibit NTB phases, given that they satisfy the prerequisite bent shape and in the case of 16 and 17 possess methylene-linking groups. The identification of the nematic and smectic C phases was trivial from their defect textures, the former exhibiting a schlieren texture and the latter exhibiting both focal–conic defects and a schlieren texture. The magnitude of the enthalpy associated with the X-SmC transition suggests that the lower temperature phase is soft-crystalline in nature rather than liquid-crystalline. The optical textures of this unknown smectic are primarily comprised of broken banded focal conics (which are presumably paramorphotic from the smectic C phase); in this regard, the X phase is reminiscent of the crystal E phase. Photomicrographs are given in .
Table 2. Transition temperatures (°C) and associated enthalpies of transition (kJ mol−1) for compounds 14–17, as determined by DSC with a heat/cool rate of 10°C min−1.
We hypothesise that the lack of the NTB phase in 14–17 can be attributed to two distinct structural features. First, the length of the ether-linked spacer is rather long and may favour the formation of smectic phases in an analogous manner to how the length of terminal chain dictates the incidence of either the NTB or SmCA phase in apolar dimers [Citation67]. It is known that the bend-angle dictates the incidence of the twist-bend nematic phase; this is demonstrated by comparing CB9CB (which exhibits the NTB phase) to the bis ether-linked CBO7OCB (which does not). The material CB8OCB () is ‘halfway’ between these two materials, having one methylene- and one ether-linking group, and this also exhibits the NTB phase, having been reported by us previously [Citation40] and more recently by others [Citation21]. It has been observed that as the number of ether linkages increases, the scaled NTB transition temperature (TNTB-N/TN-Iso) decreases irrespective of the actual values of TNTB-N. We therefore opted to reduce the number of ether-linking groups and prepare materials that can be thought of, in crude terms, as oligomeric analogues of CB6OCB, reported by Paterson et al. [Citation33], with our synthetic approach outlined in . We hypothesised that the reduction in the number of ether linkages would increase the scaled NTB transition temperature and therefore afford further examples of oligomeric twist-bend nematogens.
Figure 8. (Colour online) (a) Unaligned 2D SAXS obtained for the SmX phase of compound 20 at 97°C, (b) plot of scattered intensity as a function of Q (Å−1) obtained from a radial integration of 2D SAXS data. For (b) a 3-term Gaussian fit to the data was used to obtain spacings (both Q/Å−1 and d/Å) and correlation lengths (ζ/Å) for each individual peak. (c) Photomicrograph (100×) of the SmX phase of 20 at 91.5°C on untreated glass slides, viewed under crossed polarisers. (d) The molecular structure and transition temperatures of the previously reported material that exhibits the SmX phase [Citation70].
![Figure 8. (Colour online) (a) Unaligned 2D SAXS obtained for the SmX phase of compound 20 at 97°C, (b) plot of scattered intensity as a function of Q (Å−1) obtained from a radial integration of 2D SAXS data. For (b) a 3-term Gaussian fit to the data was used to obtain spacings (both Q/Å−1 and d/Å) and correlation lengths (ζ/Å) for each individual peak. (c) Photomicrograph (100×) of the SmX phase of 20 at 91.5°C on untreated glass slides, viewed under crossed polarisers. (d) The molecular structure and transition temperatures of the previously reported material that exhibits the SmX phase [Citation70].](/cms/asset/33db54b3-6840-4e64-b3a5-ffacb6e97844/tlct_a_1343500_f0008_oc.jpg)
Figure 9. The molecular structure and transition temperatures of CBO7OCB, CB8OCB and CB9CB as taken from ref. [Citation40].
![Figure 9. The molecular structure and transition temperatures of CBO7OCB, CB8OCB and CB9CB as taken from ref. [Citation40].](/cms/asset/d78514a8-1631-4a0d-8ad0-ee6d6a7ccf57/tlct_a_1343500_f0009_b.gif)
The useful alcohol-terminated cyanobiphenyl i14 was prepared via a Sonogashira coupling followed by a Suzuki–Miyaura reaction in high yield. Benzyl protection of the hydroxyl group of i13 followed by a Suzuki–Miyaura coupling with 4-hydroxyphenyl boronic acid afforded i16, with a subsequent etherification via the Mitsunobu protocol affording i17. We envisioned performing a selective heterogeneous hydrogenation/hydrogenolysis using palladium on carbon poisoned with diaminoethane (Pd/C{En}) so as to avoid unwanted reduction of the nitrile, affording the alcohol-terminated dimer 19, which was then, in turn, etherified with 4-cyano-4′-hydroxybiphenyl to afford a trimer. By etherifying 19 with i16, followed by selective hydrogenation, we believed it would be possible to obtain monodisperse oligomeric materials of virtually any given length in a complimentary manner to the ‘n+1’ method reported by us recently [Citation55], affording the CB6O[B6O]nCB series of materials (). Although we could obtain quantitative reduction of the alkyne groups, we were unable to obtain the debenzylated material 19, instead obtaining quantitative conversion to the saturated, benzyl-protected material 18. Despite screening a range of conditions, we are yet to find a suitable combination of catalyst and poison that affects debenzylation without accompanying nitrile reduction.
When studied by POM and DSC, we observed that compound 18 exhibits both nematic and twist-bend mesophases, although at greatly reduced temperatures to the ‘parent’ material CB6OCB. The related dialkyne i17 was found to be non-mesogenic, melting directly to the isotropic liquid and exhibiting no monotropic mesophases on supercooling. The contrast in thermal behaviour between 18 and i17 mirrors our previous observations of alkyne- and methylene-linking groups in dimers, namely that the former exhibits reduced transition temperatures relative to the latter [Citation40,Citation41]. We believe that it could be possible for a ‘calamitic’ material to exhibit the twist-bend phase via the formation of supramolecular oligomers formed via non-covalent interactions (hydrogen bonds, halogen bonds, etc.). For instance it is well known that alcohols form moderately strong hydrogen bonds to nitriles [Citation68], and for this reason we studied i14 and its saturated analogue (i18, obtained via Pd/C {En} catalysed selective hydrogenation) by POM and DSC. Neither material exhibits the NTB phase: with i14 exhibiting nematic and an unknown smectic (SmY) mesophases and i18 exhibiting only a nematic phase. In the case of i18 the material was supercooled to −60°C and remained in the nematic phase with no further phase transitions observed. With i14, identification of the smectic phase (SmY) was hindered by the fact that the phase transition occurred simultaneously with crystallisation. The transition temperatures of materials from are given in .
Table 3. Transition temperatures (°C) and associated enthalpies of transition (kJ mol−1) for compounds 20 and 21 as determined by DSC with a heat/cool rate of 10°C min−1.
Table 4. Transition temperatures (°C) and associated enthalpies of transition (kJ mol−1) for compounds i17 and 19 as determined by DSC with a heat/cool rate of 10°C min−1.
As a workaround to the problem of o-debenzylation in the presence of an aromatic nitrile, we opted to prepare oligomesogenic materials with one ‘apolar’ 4-alkoxybiphenyl mesogenic unit and one ‘polar’ cyanobiphenyl mesogenic unit, with multiple biphenyl groups interspersed between. As the aromatic nitrile group is introduced last the problems of selective hydrogenation were avoided. We elected to use a hexyloxy chain at one end of the molecule for two reasons: first, this is the same length and parity as the spacer unit; second, in our previous work on apolar bimesogens we observed this chain length to be the cross-over point from twist-bend nematic to smectic behaviour [Citation65].
A Mitsunobu etherification of i16 and i20 afforded the o-benzylated compound i21. Hydrogenation saturated the alkynes and cleaved the benzyl ether, affording an alcohol. The length of the oligomesogen can then be extended via a Mitsunobu coupling of the debenzylated material, i23, with i16 or the oligomer could be ‘capped’ with 4-hydroxy-4′-cyanobiphenyl (i25). A full account of these materials, their intermediates (many of which are liquid-crystalline in their own right) and higher oligomers will be given in a future paper. We note that the reactions used to prepare these materials occur with minimal side-products and are much higher in yield than the methods used to prepare oligomers by us previously (the hexamesogen O67 was prepared in a ~13% yield, compared to ~60–80% for the present oligomeric materials) [Citation55].
Although the related material CB6OCB exhibits nematic and twist-bend nematic mesophases, the homologue 20 (where n = 0) exhibits nematic and SmX mesophases. Extending from the dimer to the trimer, 21, the material no longer exhibits a nematic phase and instead has a direct Iso-SmX transition, as in . The SmX phase exhibited by 20 and 21 displayed similar optical textures (see )) to the SmX phase exhibited by compounds 14–17, shown in the photomicrographs in . Due to the reduced viscosity of 20 relative to the trimeric (21) and tetrameric (14–17) materials, it was possible to study the SmX phase by SAXS (Figure 10). Although the nematic phase of 20 was well aligned by the external field (B ≈ 0.6 T at sample) perpendicular to the incident X-ray beam, this alignment was lost on entering the SmX phase. The SmX mesophase is characterised by having two Bragg scattering peaks at small angles (Q = 0.19 Å−1 and Q = 0.38 Å−1) as well as two more diffuse peaks at wide angles (Q = 1.40 Å−1 and Q = 1.54 Å−1). The two Bragg scattering peaks correspond roughly to the molecular length (Q = 0.19 Å−1) and one half of the molecular length (Q = 0.38 Å−1), which was calculated to be 33 Å at the DFT(B3LYP/6-31G(d,p)) level in Gaussian G09 [Citation57]. The diffuse wide angle peaks correspond to lateral separation of the molecules within the smectic layers; our interpretation is that the differing mesogenic units (4-cyano and 4-hexyloxy-biphenyls) have differing side-to-side packing distances because of differences in polarity. Due to the lack of alignment attained for the SmX phase of 20, it is not yet possible to give definitive phase identification; however, the SAXS pattern appears consistent with an unaligned soft-crystalline E mesophase [Citation69]. The observed scattering pattern is nearly identical to that reported by us previously for what was then referred to as the ‘SmX’ mesophase [Citation70]. Given the similarity in molecular structure between this previous material ()) and compound 20, it is perhaps unsurprising that both apparently exhibit the same intercalated, soft-crystalline mesophase.
Conclusions and outlook
We have prepared and characterised several novel ether-linked dimers to exhibit the NTB phase, and within this homologous series the linear relationship between TNTB-N and TN-Iso – recently observed for methylene-linked dimers – appears to be obeyed. Extending the aspect ratio of one mesogenic unit from 2- to 3-rings confers a predictable increase in both TN-I and TNTB-N. Over the past few years, the number of dimeric materials known to exhibit the NTB phase has increased rapidly, and this work significantly increases the number of ether-linked dimers known to exhibit this intriguing state of matter.
The discovery of trimesogenic, tetramesogenic and hexamesogenic materials that exhibit the twist-bend nematic phase presents fresh impetus to prepare novel liquid-crystalline oligomeric compounds. It has been noted that the synthesis of higher linear oligomers (i.e. n ≥ 4) is often challenging due to low reaction yields due to side- and by-products during synthesis and difficult chromatographic separation. By excluding carboxylate esters and preparing oligomeric materials featuring biphenyl-derived mesogenic groups, we find that reactions proceed significantly ‘cleaner’ and the synthesis of these materials is greatly simplified. Compounds 14–17 were found not to exhibit the NTB phase, instead variously exhibiting nematic, smectic C and crystal E mesophases. With this in mind we have attempted to combine the advantages of both approaches, namely employing a short spacer with both one methylene- and one ether-linking unit to promote the formation of the NTB phase and using biphenyl mesogenic units rather than phenyl benzoates to improve yield and reduce side reactions (predominantly transesterification).
The onus is shifting to understanding how, if at all, changes to molecular structure in dimers impact on the properties of the NTB phase such as conical angle, pitch length, etc., whereas for oligomers the synthesis of further materials that exhibit the NTB phase is essential for advancing understanding of these systems to the same level as that of dimers. Ether-linked oligomers are synthetically accessible but do not appear to exhibit the NTB phase [Citation71], whereas methylene-linked oligomers present more of a challenge to synthesise but do exhibit this mesophase. Further developments to the stepwise ‘n+1’ synthetic methods presented by us here (and in previous work [Citation55]) should allow the synthesis of high-purity, monodisperse oligomeric materials of any length and help to increase understanding of these systems within the context of the NTB phase.
Acknowledgements
The authors thank the Department of Chemistry at the University of York for funding a summer placement for MPS. The EPSRC is thanked for funding the Bruker D8 small angle X-ray scattering instrument used in this work via grant EP/K039660/1, and for funding via grant EP/M020584/1
Disclosure statement
No potential conflict of interest was reported by the authors.
Supplemental data
Supplemental data for this article can be accessed here.
Additional information
Funding
References
- Imrie CT, Henderson PA, Yeap GY. Liquid crystal oligomers: going beyond dimers. Liq Cryst. 2009;36:755–777.
- Imrie CT, Henderson PA. Liquid crystal dimers and higher oligomers: between monomers and polymers. Chem Soc Rev. 2007;36:2096–2124.
- Henderson PA, Cook AG, Imrie CT. Oligomeric liquid crystals: from monomers to trimers. Liq Cryst. 2004;31:1427–1434.
- Imrie CT, Henderson PA. Liquid crystal dimers and oligomers. Curr Opin Colloid In. 2002;7:298–311.
- Imrie CT, Lu ZB, Picken SJ, et al. Oligomeric rod-disc nematic liquid crystals. Chem Commun. 2007;1245–1247.
- Dozov I. On the spontaneous symmetry breaking in the mesophases of achiral banana-shaped molecules. Europhys Lett. 2001;56:247–253.
- Sepelj M, Lesac A, Baumeister U, et al. Dimeric salicylaldimine-based mesogens with flexible spacers: parity-dependent mesomorphism. Chem Mater. 2006;18:2050–2058.
- Sepelj M, Lesac A, Baumeister U, et al. Intercalated liquid-crystalline phases formed by symmetric dimers with an alpha,omega-diiminoalkylene spacer. J Mater Chem. 2007;17:1154–1165.
- Panov VP, Nagaraj M, Vij JK, et al. Spontaneous periodic deformations in nonchiral planar-aligned bimesogens with a nematic-nematic transition and a negative elastic constant. Phys Rev Lett. 2010;105:167801.
- Cestari M, Diez-Berart S, Dunmur DA, et al. Phase behavior and properties of the liquid-crystal dimer 1″,7″-bis(4-cyanobiphenyl-4ʹ-yl) heptane: a twist-bend nematic liquid crystal. Phys Rev E Stat Nonlin Soft Matter Phys. 2011;84:031704.
- Henderson PA, Imrie CT. Methylene-linked liquid crystal dimers and the twist-bend nematic phase. Liq Cryst. 2011;38:1407–1414.
- Panov VP, Balachandran R, Nagaraj M, et al. Microsecond linear optical response in the unusual nematic phase of achiral bimesogens. Appl Phys Lett. 2011;99:261903.
- Adlem K, Copic M, Luckhurst GR, et al. Chemically induced twist-bend nematic liquid crystals, liquid crystal dimers, and negative elastic constants. Phys Rev E Stat Nonlin Soft Matter Phys. 2013;88:022503.
- Borshch V, Kim YK, Xiang J, et al. Nematic twist-bend phase with nanoscale modulation of molecular orientation. Nat Commun. 2013;4:2635.
- Chen D, Porada JH, Hooper JB, et al. Chiral heliconical ground state of nanoscale pitch in a nematic liquid crystal of achiral molecular dimers. Proc Natl Acad Sci U S A. 2013;110:15931–15936.
- Zhang ZP, Panov VP, Nagaraj M, et al. Raman scattering studies of order parameters in liquid crystalline dimers exhibiting the nematic and twist-bend nematic phases. J Mater Chem C. 2015;3:10007–10016.
- Mandle RJ. The dependency of twist-bend nematic liquid crystals on molecular structure: a progression from dimers to trimers, oligomers and polymers. Soft Matter. 2016;12:7883–7901.
- Lu ZB, Henderson PA, Paterson BJA, et al. Liquid crystal dimers and the twist-bend nematic phase. The preparation and characterisation of the alpha,omega-bis(4-cyanobiphenyl-4′-yl) alkanedioates. Liq Cryst. 2014;41:471–483.
- Paterson DA, Xiang J, Singh G, et al. Reversible isothermal twist-bend nematic-nematic phase transition driven by the photoisomerization of an azobenzene-based nonsymmetric liquid crystal dinner. J Am Chem Soc. 2016;138:5283–5289.
- Challa PK, Borshch V, Parri O, et al. Twist-bend nematic liquid crystals in high magnetic fields. Phys Rev E Stat Nonlin Soft Matter Phys. 2014;89:060501.
- Paterson DA, Abberley JP, Harrison WTA, et al. Cyanobiphenyl-based liquid crystal dimers and the twist-bend nematic phase. Liq Cryst. 2017;44:127–146.
- Abberley JP, Jansze SM, Walker R, et al. Structure–property relationships in twist-bend nematogens: the influence of terminal groups. Liq Cryst. 2017;44:68–83.
- Vanakaras AG, Photinos DJ. A molecular theory of nematic-nematic phase transitions in mesogenic dimers. Soft Matter. 2016;12:2208–2220.
- Stevenson WD, Ahmed Z, Zeng XB, et al. Molecular organization in the twist-bend nematic phase by resonant X-ray scattering at the Se K-edge and by SAXS, WAXS and GIXRD. Arxiv E-Prints. 2016;1612.01180.
- Sebastian N, Tamba MG, Stannarius R, et al. Mesophase structure and behaviour in bulk and restricted geometry of a dimeric compound exhibiting a nematic-nematic transition. Phys Chem Chem Phys. 2016;18:19299–19308.
- Ramou E, Ahmed Z, Welch C, et al. The stabilisation of the Nx phase in mixtures. Soft Matter. 2016;12:888–899.
- Pardaev SA, Shamid SM, Tamba MG, et al. Second harmonic light scattering induced by defects in the twist-bend nematic phase of liquid crystal dimers. Soft Matter. 2016;12:4472–4482.
- Panov VP, Vij JK, Mehl GH. Twist-bend nematic phase in cyanobiphenyls and difluoroterphenyls bimosogens. Liq Cryst. 2017;44:147–159.
- Emsley JW, Lelli M, Joy H, et al. Similarities and differences between molecular order in the nematic and twist-bend nematic phases of a symmetric liquid crystal dimer. Phys Chem Chem Phys. 2016;18:9419–9430.
- Tamba MG, Salili SM, Zhang C, et al. A fibre forming smectic twist-bent liquid crystalline phase. Rsc Adv. 2015;5:11207–11211.
- Ahmed Z, Welch C, Mehl GH. The design and investigation of the self-assembly of dimers with two nematic phases. Rsc Adv. 2015;5:93513–93521.
- Sebastián N, Robles-Hernández B, Diez-Berart S, et al. Distinctive dielectric properties of nematic liquid crystal dimers. Liq Cryst. 2017;44:177–190.
- Paterson DA, Gao M, Kim YK, et al. Understanding the twist-bend nematic phase: the characterisation of 1-(4-cyanobiphenyl-4′-yloxy)-6-(4-cyanobiphenyl-4′-yl)hexane (CB6OCB) and comparison with CB7CB. Soft Matter. 2016;12:6827–6840.
- Dawood AA, Grossel MC, Luckhurst GR, et al. On the twist-bend nematic phase formed directly from the isotropic phase. Liq Cryst. 2016;43:2–12.
- Robles-Hernandez B, Sebastian N, de la Fuente MR, et al. Twist, tilt, and orientational order at the nematic to twist-bend nematic phase transition of 1″,9″-bis(4-cyanobiphenyl-4′-yl) nonane: a dielectric, H-2 NMR, and calorimetric study. Phys Rev E. 2015;92(6):062505.
- Meyer C, Luckhurst GR, Dozov I. The temperature dependence of the heliconical tilt angle in the twist-bend nematic phase of the odd dimer CB7CB. J Mater Chem C. 2015;3:318–328.
- Xiang J, Li YN, Li Q, et al. Electrically tunable selective reflection of light from ultraviolet to visible and infrared by heliconical cholesterics. Adv Mater. 2015;27:3014–3018.
- Xiang J, Varanytsia A, Minkowski F, et al. Electrically tunable laser based on oblique heliconical cholesteric liquid crystal. P Natl Acad Sci USA. 2016;113:12925–12928.
- Mandle RJ, Goodby JW. Does topology dictate the incidence of the twist-bend phase? Insights gained from novel unsymmetrical bimesogens. Chem-Eur J. 2016;22:18456–18464.
- Mandle RJ, Archbold CT, Sarju JP, et al. The dependency of nematic and twist-bend mesophase formation on bend angle. Sci Rep-UK. 2016;6:36682.
- Archbold CT, Mandle RJ, Andrews JL, et al. Liq Cryst. 2017. Submitted for publication.
- Sreenilayam SP, Panov VP, Vij JK, et al. The NTB phase in an achiral asymmetrical bent-core liquid crystal terminated with symmetric alkyl chains. Liq Cryst. 2017;44:244–253.
- Cukrov G, Mosaddeghian Golestani Y, Xiang J, et al. Comparative analysis of anisotropic material properties of uniaxial nematics formed by flexible dimers and rod-like monomers. Liq Cryst. 2017;44:219–231.
- Mandle RJ, Goodby JW. Dependence of mesomorphic behaviour of methylene-linked dimers and the stability of the NTB/NX phase upon choice of mesogenic units and terminal chain length. Chemistry. 2016;22:9366–9374.
- Ivsic T, Vinkovic M, Baumeister U, et al. Towards understanding the N-TB phase: a combined experimental, computational and spectroscopic study. Rsc Adv. 2016;6:5000–5007.
- Ivsic T, Vinkovic M, Baumeister U, et al. Retraction. Milestone in the NTB phase investigation and beyond: direct insight into molecular self-assembly. Soft Matter. 2015;11:6716.
- Gorecka E, Vaupotic N, Zep A, et al. A twist-bend nematic (N-TB) phase of chiral materials. Angew Chem Int Edit. 2015;54:10155–10159.
- Sebastian N, Lopez DO, Robles-Hernandez B, et al. Dielectric, calorimetric and mesophase properties of 1″-(2ʹ,4-difluorobiphenyl-4ʹ-yloxy)-9″-(4-cyanobiphenyl-4ʹ-yloxy) nonane: an odd liquid crystal dimer with a monotropic mesophase having the characteristics of a twist-bend nematic phase. Phys Chem Chem Phys. 2014;16:21391–21406.
- Mandle RJ, Davis EJ, Lobato SA, et al. W. Synthesis and characterisation of an unsymmetrical, ether-linked, fluorinated bimesogen exhibiting a new polymorphism containing the N(TB) or ‘twist-bend’ phase. Phys Chem Chem Phys. 2014;16:6907–6915.
- Chen D, Nakata M, Shao R, et al. Twist-bend heliconical chiral nematic liquid crystal phase of an achiral rigid bent-core mesogen. Phys Rev E Stat Nonlin Soft Matter Phys. 2014;89:022506.
- Jansze SM, Martinez-Felipe A, Storey JM, et al. A twist-bend nematic phase driven by hydrogen bonding. Angew Chem Int Ed Engl. 2015;54:643–646.
- Wang Y, Singh G, Agra-Kooijman DM, et al. Room temperature heliconical twist-bend nematic liquid crystal. Crystengcomm. 2015;17:2778–2782.
- Mandle RJ, Goodby JW. A liquid crystalline oligomer exhibiting nematic and twist-bend nematic mesophases. Chemphyschem. 2016;17:967–970.
- Mandle RJ, Goodby JW. Progression from nano to macro science in soft matter systems: dimers to trimers and oligomers in twist-bend liquid crystals. Rsc Adv. 2016;6:34885–34893.
- Simpson FP, Mandle RJ, Goodby JW, et al. J Mater Chem C. 2017;5:5102–5110.
- Archbold CT, Andrews JL, Mandle RJ, et al. Effect of the linking unit on the twist-bend nematic phase in liquid crystal dimers: a comparative study of two homologous series of methylene- and ether-linked dimers. Liq Cryst. 2017;44:84–92.
- Frisch MJ, Trucks GW, Schlegel HB, et al. Gaussian 09. Wallingford (CT): Gaussian, Inc; 2009.
- Mandle RJ, Davis EJ, Voll CCA, et al. Self-organisation through size-exclusion in soft materials. J Mater Chem C. 2015;3:2380–2388.
- Mandle RJ, Davis EJ, Sarju JP, et al. Control of free volume through size exclusion in the formation of smectic C phases for display applications. J Mater Chem C. 2015;3:4333–4344.
- Davis EJ, Mandle RJ, Russell BK, et al. Liquid-crystalline structure-property relationships in halogen-terminated derivatives of cyanobiphenyl. Liq Cryst. 2014;41:1635–1646.
- Mandle RJ, Cowling SJ, Sage I, et al. Relationship between molecular association and re-entrant phenomena in polar calamitic liquid crystals. J Phys Chem B. 2015;119:3273–3280.
- Mandle RJ, Davis EJ, Lobato SA, et al. Synthesis and characterisation of an unsymmetrical, ether-linked, fluorinated bimesogen exhibiting a new polymorphism containing the N-TB or ‘twist-bend’ phase. Phys Chem Chem Phys. 2014;16:6907–6915.
- Mandle RJ, Voll CCA, Lewis DJ, et al. Etheric bimesogens and the twist-bend nematic phase. Liq Cryst. 2016;43:13–21.
- Mandle RJ, Davis EJ, Voll CCA, et al. The relationship between molecular structure and the incidence of the N-TB phase. Liq Cryst. 2015;42:688–703.
- Mandle RJ, Davis EJ, Archbold CT, et al. Apolar bimesogens and the incidence of the twist-bend nematic phase. Chem-Eur J. 2015;21:8158–8167.
- Sajiki H, Hattori K, Hirota K. The formation of a novel Pd/C-ethylenediamine complex catalyst: chemoselective hydrogenation without deprotection of the O-benzyl and N-Cbz groups. J Org Chem. 1998;63:7990–7992.
- Mandle RJ, Goodby JW. Dependence of mesomorphic behaviour of methylene-linked dimers and the stability of the N-TB/N-X phase upon choice of mesogenic units and terminal chain length. Chem-Eur J. 2016;22:9366–9374.
- Le Questel JY, Berthelot M, Laurence C. Hydrogen-bond acceptor properties of nitriles: a combined crystallographic and ab initio theoretical investigation. J Phys Org Chem. 2000;13:347–358.
- Leadbetter AJ, Mazid MA, Malik KMA. The crystal and molecular-structure of isobutyl 4-(4ʹ-phenylbenzylidene-amino)cinnamate (ibpbac) - and the crystal smectic-E transition. Mol Cryst Liq Cryst. 1980;61:39–59.
- Mandle RJ, Goodby JW. Intercalated soft-crystalline mesophase exhibited by an unsymmetrical twist-bend nematogen. Crystengcomm. 2016;18:8794–8802.
- Imrie CT, Luckhurst GR. Liquid crystal trimers. The synthesis and characterisation of the 4,4′-bis [omega-(4-cyanobiphenyl-4′-yloxy)alkoxy]biphenyls. J Mater Chem. 1998;8:1339–1343.