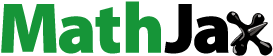
ABSTRACT
The splay, twist and bend elastic constants (K11, K22 and K33) have been measured as a function of temperature in bent-core/calamitic mixtures based on three different calamitic materials (5CB, 8CB and ZLI1132) and two bent-core dopants. The behaviour of the splay and bend constants are as expected; a reduction in K33 of ~20%, in line with predictions from mixing rules and other observations. Interestingly, no change is seen in the splay constant, K11 of the calamitic hosts. Surprisingly though, the twist elastic constant exhibits a reduction of 30 – 40% in all mixtures across the nematic range, an effect not previously reported and much larger than mixing rules can explain. The elastic behaviour is universal in our mixtures. We explain part of the reduction in the twist deformation by considering the influence of the chiral conformer fluctuations of the bent-core molecules on the twist elastic constants of the mixtures. However, the dramatic reduction can only be fully explained by also including contributions from chiral conformer fluctuations of the calamitic host, a form of chiral amplification.
Graphical abstract
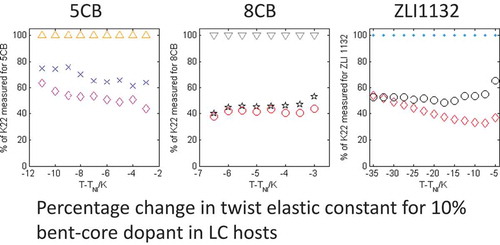
1. Introduction
Nematic liquid crystals (NLCs) are fluids with long-range orientational ordering that exhibit anisotropy in their optical, electrical and elastic properties. The anisotropy, together with their electro-optical responsiveness has led to NLCs being used widely in devices. Most NLCs that are used in devices tend to be rod-like (calamitic) in shape but more recently nematic phases formed from bent-core (BC) molecules have garnered interest, due to properties including increased flexoelectricity [Citation1,Citation2] as well as a reversal of the relationship between the splay (K11) and bend (K33) elastic constants, ie. K11 > K33 [Citation3–Citation9].
Elastic constants are important features of liquid crystals as they are fundamental physical properties that influence both the threshold voltage and the steepness of the electro-optical response in NLC devices. The twist (K22) constant in particular is significant as it is directly related to the Fréedericksz transition threshold voltage in twisted nematic (TN) [Citation10] and in-plane switching (IPS) devices [Citation11]. Whilst TN devices are used less nowadays, IPS devices are still widely employed in display devices such as TVs and lower K22 values will result in reduced threshold voltages in devices, with consequent potential for practical applications. However, perhaps more interestingly, significant reduction in the elastic constants in single-molecule nematic materials is associated with the observation of nematic variant phases, such as the twist-bend nematic (NTB) phase [Citation12–Citation15]. Thus, understanding how the elastic constants might be manipulated is also of fundamental interest in controlling the appearance of nematic variant phases in mixtures. In this paper, we report a reduction in the twist and bend elastic constants when NLCs are doped with 10% of bent-core mesogens, whilst the splay elastic constant remains unaffected. The reduction in the bend elastic constant in such mixtures is very well known and our results are in line with those of others, but a much more significant, particularly dramatic fall is seen in the twist constant.
We have selected two bent-core compounds with unusually low twist and bend elastic constants [Citation16] as dopants, choosing a single concentration (10% weight) in calamitic NLCs for this study. Two of the calamitic liquid crystals chosen are 5CB and 8CB; both have very well-known elastic behaviour and 8CB exhibits pretransitional divergence of the twist and bend elastic constants close to the transition to the smectic A phase. We have also studied mixtures of the BC materials with a broad temperature-range nematic mixture, ZLI 1132 (Merck).
Reductions in K33 in bent-core/calamitic mixtures are well known and have been described by a number of other groups. Kundu et al. [Citation17] doped 8OCB with a bent-core mesogen referred to as BC12 [Citation18] and reported that K33 reduced from ~6–50 pN in 8OCB to ~2.5–4 pN in a 11:89 BC12:8OCB mixture, along with a lower temperature dependence of K33 in the mixture. Sathyanarayana et al. [Citation19] doped 5CB with bent-core mesogens [Citation20] and measured K33 to decrease from ~3.5–9 pN in 5CB to ~1.5–3.5 pN when they added 12% of the bent-core mesogen to 5CB. Parthasarathi et al. [Citation21] made a number of binary mixtures of CB7CB, which exhibits a twist bend (NTB) phase, with 7OCB. When 7OCB was doped with 10% of CB7CB, K33 was found to reduce from ~3–3.5 pN in 7OCB to ~0.5–2 pN in the mixture. Whilst we also report reductions in K33 in our mixtures, we further provide the first detailed study describing how K22 is affected in such mixtures. We also find that K11 is unchanged by the inclusion of the bent-core mesogen, as reported by the aforementioned groups.
As mentioned above, it has been shown that significant reductions in the elastic constants are a driving factor in forming more exotic phases, such as the NTB phase [Citation12–Citation15], the dark conglomerate (DC) phase [Citation22,Citation23], and more recently the splay nematic phase, described by Mertelj et al., which exhibits low splay and twist values (K11 and K33 ~ 2 pN) [Citation24]. Indeed, two of our mixtures (mixtures 4 and 5 in ) also exhibit unusual behaviour in the nematic phase; filaments form through self-assembly at temperatures below the isotropic-nematic transition. Mixture 4 has already been described by Gleeson et al. [Citation25] to demonstrate interesting rheological and structural properties when the filaments form. The X-ray scattering data in ref. 25 suggests that the filaments form a rectangular columnar structure, similar to that observed in the NTB material described by Chen et al. [Citation26]., which then evolves into a hexagonal columnar structure. By understanding the behaviour of the elastic constants in the nematic phase of these mixtures, we can also draw conclusions regarding whether the formation of this filament phase is analogous to any of the nematic variant phases.
Table 1. Compositions of the pure materials and mixtures with the phase temperatures determined on cooling, where Iso = isotropic phase, N = nematic phase, SmX = smectic phase of unknown type, Cr = crystal phase, N + F = nematic phase with filaments, SmA = smectic A phase and SmA+F = smectic A phase with filaments. The elastic constants of the pure materials and the mixtures are all given in the results section. Synthesis of compound 1 is available from the linked data repository https://doi.org/10.5518/482.
2. Experimental methods
2.1 Materials and devices
The molecular structures and phase transition temperatures of the pure bent-core and calamitic liquid crystals used in this work are shown in . The elastic constants K22 and K33 of bent-core materials, compound 1 and 2, are ~0.1 pN and ~0.7 pN (twist) and ~1 pN and 3 pN (bend), respectively [Citation16]. In addition to the pure calamitic materials, 5CB and 8CB a nematic mixture, ZLI 1132 was also used. ZLI 1132 is a Merck mixture that is nematic at room temperature, with a nematic to isotropic transition temperature of TNI = 71.2°C. The transition temperatures of the pure materials and the mixtures were determined by differential scanning calorimetry (DSC) and polarising optical microscopy (POM); the temperatures in were taken from the DSC measurements. Mixtures were made by dissolving the pure compounds in a vial using dichloromethane (DCM) before allowing the DCM to evaporate completely at a temperature of 50°C. shows a graphical representation of the transition temperatures for all mixtures from .
Figure 1. (Colour online) Histogram showing the phase transitions with the transition temperatures determined on cooling for mixtures 1–6. Everything above the bars indicates the isotropic phase, with the dark blue bars representing the nematic phase, the light blue bar represents the nematic phase with filaments, the orange bars represent the smectic A phase and the light orange bar represents the smectic A phase with filaments.
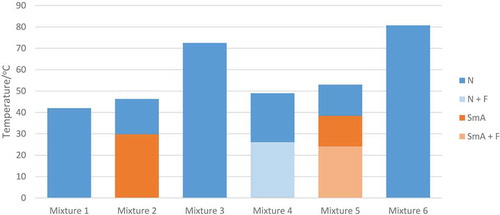
All electrical and electro-optic measurements were carried out using devices of nominal thickness 5 μm which were treated for planar alignment (nematic director parallel to substrates), obtained using a rubbed polyimide layer (SE130). Two types of devices were used to measure the elastic constants as described in detail previously [Citation16,Citation27,Citation28]; a planar homogeneous device with electrodes to apply a field perpendicular to the substrates was used to measure K11 and K33, and a planar in-plane switching (IPS) device (with a field in the plane of the substrates) was used to measure K22. The temperature of the devices was controlled with a relative accuracy of ±0.1°C using a Linkam LTS 350 hot stage and TMS 94 temperature controller. All electric fields were applied at a frequency of 10 kHz avoiding any relaxations in the systems. The devices were capillary filled in the isotropic phase. Gleeson et al. [Citation25] report that the filaments exhibited by mixtures 4 and 5 cause the system to become very viscous; the complex viscosity was measured to be ~3.12x107 mPas at 25°C, compared to pure 5CB which normally exhibits a complex viscosity of ~100 mPas at the same temperature. To avoid this regime of high viscosity, we made sure that measurements were taken in the nematic phases of the mixtures with the experiments completed before the filaments were observed to grow.
2.2 Measurement of the dielectric anisotropy and elastic constants
The dielectric anisotropy (), and splay and bend elastic constants were deduced from a fit to measurements of the dielectric permittivity (
) determined as a function of applied voltage across the Fréedericksz transition, a method employed successfully with many other NLC systems [Citation16,Citation27,Citation28]. The dielectric measurements of the Fréedericksz transition were carried out as a function of temperature in suitable device geometries across the nematic phase regime using an Agilent Precision LCR Metre E4980A. A detailed fit is made to the permittivity – voltage curve, from which the perpendicular (
) and parallel components (
), and hence
can be deduced (
). A typical data set and associated fit can be found in DOI: https://doi.org/10.5518/482 where all of the data for this paper has been deposited. An accuracy of ~5% or 0.2 (whichever is larger) in
is assumed. The fit also provides the splay (K11) and bend (K33) elastic constants, making use of the value of
deduced. The methodology to calculate
,
, K11 and K33 is described in detail in previous publications [Citation27–Citation29], and allows K11 and K33 to be determined with an accuracy of ~5% or 0.1 pN (whichever is larger). The twist constant was measured similarly using an IPS device geometry in which K22 related to the threshold voltage [Citation11];
where is the electrode separation in the IPS device (20 μm) and
is the device thickness (nominally 5 μm, but determined independently in each experiment). The intensity of light transmitted by the in-plane device held between crossed polarisers was monitored and extrapolation of the approximately linear portion of the transmission-voltage curve to the baseline was used to determine the threshold
. Typical data and details can be found in DOI: https://doi.org/10.5518/482. This method allows K22 to be measured with a relative accuracy of ~20% for each data set, although the absolute accuracy of this method is no better than ~33% because of the non-uniform electric fields in the geometry [Citation28,Citation29].
These methods of determining the elastic constants do not take into account any contributions from flexoelectric effects. However, it has been found previously that in compound 2, the flexoelectric coefficients are only a factor of 2 greater than calamitic LCs [Citation1,Citation2] so this omission is justified. All measurements of the dielectric anisotropy and elastic constants were taken on cooling and are presented as a function of reduced temperature T-TNI.
3. Results
Due to the variety of mixtures we are reporting in this paper, we will discuss the results in three subsections; the first subsection will compare the experimental results in mixtures of 5CB, whilst the second and third will do so for mixtures of 8CB and ZLI 1132, respectively.
3.1 Dielectric anisotropy and elastic constants in mixtures 1 and 4; 5CB
The dielectric anisotropy, of mixtures 1 and 4 increases as the temperature decreases, as seen in . The mixtures exhibit reduced
values (by ~10%) with respect to 5CB [Citation30,Citation31], due to the negative dielectric anisotropy of the bent-core mesogens [Citation16,Citation27,Citation28], and varies between ~6–12 in mixtures 1 and 4 compared to ~8.5–12.5 in 5CB. shows that the datasets for each bent core-dopant can be considered to be the same (within error) of each other. respectively, show the values of the splay, twist and bend elastic constants measured for compound 1 [Citation16], 2 [Citation27,Citation28] and mixtures 1 and 4.
Figure 2. (Colour online) Dielectric anisotropy, Δε as a function of T-TNI for 5CB (orange upward triangles), mixture 1 (blue crossed) and mixture 4 (magenta diamonds).
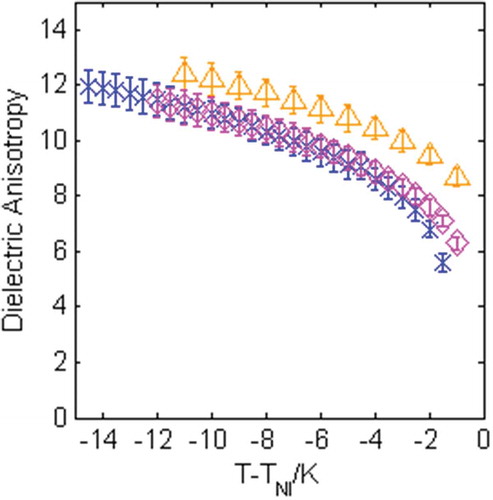
Figure 3. (Colour online) (a) The splay, K11 (a), twist, K22 (b) and bend, K33 (c) elastic constants as a function of T-TNI for compound 1 [Citation16] (maroon asterisks), compound 2 [Citation29] (green squares), 5CB (orange upward triangles), mixture 1 (blue crosses) and mixture 4 (magenta diamonds).
![Figure 3. (Colour online) (a) The splay, K11 (a), twist, K22 (b) and bend, K33 (c) elastic constants as a function of T-TNI for compound 1 [Citation16] (maroon asterisks), compound 2 [Citation29] (green squares), 5CB (orange upward triangles), mixture 1 (blue crosses) and mixture 4 (magenta diamonds).](/cms/asset/6ee719ed-2e5e-4d78-a7f7-b856446d9545/tlct_a_1687767_f0003_oc.jpg)
All three plots in show that the temperature dependence of the elastic constants is retained when 5CB is doped with the bent-core mesogens. Interestingly, ) shows that the mixtures exhibit no difference in their values of the splay constant with respect to 5CB; K11 ranges from ~1.5–6.5 pN. This set of results is somewhat surprising given that compound 1 exhibits much higher K11 values (~8–12 pN) than compound 2 (~3–4 pN), yet there is no difference in the values of K11 that mixtures 1 and 4 exhibit. In contrast and show that the mixtures exhibit a decrease in values of the twist and bend constants with respect to 5CB. The values measured vary between ~2–4 pN and ~4–10 pN for K22 and K33 in 5CB, to ~1–3.5 pN and ~2–9 pN for K22 and K33 in mixtures 1 and 4. It can also be seen that the K33 values for mixtures 1 and 3 are practically identical across the temperature range measured. However, there is insufficient evidence to suggest that mixtures 1 and 4 exhibit different values for K22 due to the uncertainties assigned to the data points. Thus, we can state that mixtures 1 and 4 exhibit K22 values that are similar within error.
In summary, we see that when 5CB is doped with 10% of either compound 1 or 2 only the K22 and K33 values are reduced with respect to 5CB by ~30% and 20%, respectively. There is no change in the temperature dependence of all three elastic constants, and both mixtures 1 and 4 exhibit elastic constant values that are practically indistinguishable from each other.
Next, we present the elastic behaviour exhibited by mixtures of 8CB.
3.2 Dielectric anisotropy and elastic constants in mixtures 2 and 5; 8CB
The values measured for for mixtures 2 and 5 (shown in ) are lower than those measured for pure 8CB, the latter of which exhibits
values varying between ~6 and 8.5. However, while mixtures 1 and 4 were indistinguishable in their values of
, here mixture 5 exhibits an apparently lower dielectric anisotropy with respect to mixture 2, varying between ~4–7 in mixture 5 and ~6–8.5 in mixture 2 across the temperature range measured. However, as with , the datasets can be considered to be within error of each other.
Figure 4. (Colour online) Dielectric anisotropy, Δε as a function of T-TNI for 8CB (grey downward triangles), mixture 2 (black pentagrams) and mixture 5 (red circles).
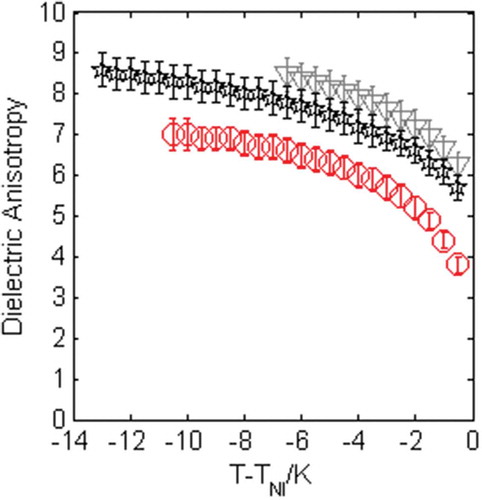
The values of K11, K22, and K33 as a function of T-TNI are shown in respectively, for 8CB, compounds 1 and 2, and mixtures 2 and 5. It can be seen from all three plots in that mixtures 2 and 4 not only exhibit elastic constant values lower than pure 8CB, but they also do not follow quite the same temperature dependence as 8CB. In 8CB we observe a divergence of the twist and bend elastic constants as we approach the smectic A (SmA) transition [Citation32]. This is clearly seen for K33 in 8CB, occurring over ~4 K above the N-SmA transition (), but is less obvious for K22 (), where the divergence occurs over ~1 K. Such data are in excellent agreement with those reported in ref. 32. The divergence of K33 is retained in mixture 2 but occurs at a lower reduced temperature as the nematic phase has been broadened in the mixture and the SmA phase occurs at a lower temperature. In , we note that filament growth occurs just before the N-SmA transition; the gel formed has a high viscosity [Citation25] and prevents measurements of K22 in the region that critical divergence of K22 would be expected.
Figure 5. (Colour online) (a) The splay, K11 (a), twist, K22 (b) and bend, K33 (c) elastic constants as a function of T-TNI for compound 1 [Citation16] (maroon asterisks), compound 2 [Citation29] (green squares), 8CB (grey downward triangles), mixture 2 (black pentagrams) and mixture 5 (red circles).
![Figure 5. (Colour online) (a) The splay, K11 (a), twist, K22 (b) and bend, K33 (c) elastic constants as a function of T-TNI for compound 1 [Citation16] (maroon asterisks), compound 2 [Citation29] (green squares), 8CB (grey downward triangles), mixture 2 (black pentagrams) and mixture 5 (red circles).](/cms/asset/8a4e8562-726a-4ef6-a4fc-8913cff4a533/tlct_a_1687767_f0005_oc.jpg)
All three plots in show that mixture 2 exhibits similar elastic constants (within error) to mixture 5. With respect to 8CB, ) shows that K11 for mixtures 2 and 5 are both effectively unchanged with respect to 8CB, ) shows ~40% reduction in K22, and ) shows ~15% reduction in K33. K33 in mixture 2 increases to ~20 pN as the SmA transition is approached, reflecting the critical divergence phenomenon. The large change in K22 (~40%) when 8CB is doped with the bent-core mesogens is especially dramatic when compared to the behaviour in mixtures 1 and 4 in 5CB. Such a dramatic reduction in K22 in bent-core/calamitic mixtures has not been previously reported and is discussed further later.
In summary, when 8CB is doped with 10% of compounds 1 and 2 we observe a slightly changed temperature dependence for all elastic constants with respect to those of 8CB. Whilst the divergence of K33 at lower temperatures (approaching the SmA phase) was maintained in mixture 2, we were unable to measure it in mixture 5 due to filament growth. The absolute values of the elastic constants are lower in the mixtures with respect to 8CB, with K22 being reduced the most, by ~40%.
Next, we present the elastic behaviour exhibited by mixtures of the bent-core compounds with the Merck mixture ZLI 1132.
3.3 Elastic constants in a mixture 3 and 6; ZLI 1132
The values of K11, K22, and K33 as a function of T-TNI are shown in respectively, for compound 1, compound 2, ZLI 1132, and mixtures 3 and 6. It can be seen from all three plots in that mixtures 3 and 6 exhibit lower K22 and K33 with respect to ZLI 1132. The K11 values exhibited by ZLI 1132 and mixtures 3 and 6 can be considered to be the same within error. The temperature dependence of all three elastic constants, compared with ZLI 1132, can be considered to be retained.
Figure 6. (Colour online) (a) The splay, K11 (a), twist, K22 (b) and bend, K33 (c) elastic constants as a function of T-TNI for compound 1 [Citation16] (maroon asterisks), compound 2 [Citation29] (green squares), ZLI 1132 (light blue dots), mixture 3 (black circles) and mixture 6 (red diamonds).
![Figure 6. (Colour online) (a) The splay, K11 (a), twist, K22 (b) and bend, K33 (c) elastic constants as a function of T-TNI for compound 1 [Citation16] (maroon asterisks), compound 2 [Citation29] (green squares), ZLI 1132 (light blue dots), mixture 3 (black circles) and mixture 6 (red diamonds).](/cms/asset/6c89b0e5-8178-4e7b-ac80-edaedbd73102/tlct_a_1687767_f0006_oc.jpg)
shows that K11 increases from ~3–10 pN in mixtures 3 and 6, unchanged compared to ~3–10 pN in ZLI 1132. ) shows that K22 varies between ~1 and 6 pN in mixtures 3 and 6 compared to ~0.5–10.5 pN in ZLI 1132. ) shows that K33 takes values between ~4–15 pN in mixtures 3 and 6 compared to ~4.5–20 pN in ZLI 1132. In summary, the changes in the elastic constants with respect to ZLI 1132 are negligible for K11, approximately 40% for K22 and ~10% for K33. Again, the dramatic reduction in K22 is especially noteworthy.
4. Discussion and theoretical insight
There are marked similarities in the behaviour of all of the mixtures studied. The first, perhaps surprising result is that the splay elastic constant, K11 is essentially unaffected by doping up to 10% of either BC material into these calamitic liquid crystals. Indeed, in the systems chosen here, one BC material exhibited relatively low and the other relatively high values of K11 but no difference was observed in the splay elastic constants of any of the mixtures. On the other hand, both the twist and bend behaviour are significantly altered in the mixtures. Indeed, an especially dramatic reduction in the twist elastic constant occurs in all mixtures to some degree.
One is led to consider why this might be the case. We can explain the influence on the bend constant and the dramatic effect on the twist elastic constant as follows. Note first that some time ago it was observed that the cholesteric pitch in a calamitic compound became shorter when doped with a relatively small amount of achiral bent-core molecules [Citation33]. At first sight, such behaviour is counterintuitive and was explained by the twist deformation inducing some deracemisation in the system of bent-core conformers which then contributes to the total helical twisting power. However, it is now clear that the same chiral conformers also reduce the twist elastic constant. Thus, the increase of the inverse pitch, in ref. 33 is due to two factors; the increase of the helical twisting power (proportional to
) due to chiral conformer fluctuations of bent-core molecules, and an additional reduction of K22 also due to the conformer fluctuations.
We now consider the influence of the dopants on the elastic constants of a nematic material and begin with a discussion of the effect of concentration of the components of the binary mixture on the elastic constants of nematics. One notes that according to the existing molecular-statistical theory of nematic LCs the Frank elastic constants are proportional to the square of the molecular number density (see, for example [Citation34–Citation37], and references therein). A sufficiently general molecular theory of elasticity of the binary mixtures of nematic LCs has also been developed [Citation38] and it indicates that the elastic constants are the quadratic forms of the corresponding molar fractions and
of the components b and c, i.e. there are no terms linear in
and
. The quadratic dependence on the molar fractions is related to the fact that the elastic constants are determined by pair intermolecular in This result can be illustrated using a simple mean-field expression for the free energy of the binary mixture of the uniaxial molecules [Citation34,Citation36,Citation38]:
where is the average number density,
is the molar fraction of the component i,
is the one-particle distribution function,
is the unit vector along the primary axis of the uniaxial molecule and
is the intermolecular interaction potential.
The first sum in this general expression describes the orientational entropy in the nematic phase. This term is linear in molar fractions , but it does not contribute to the elastic constants because it is local, i.e. it is expressed as a volume integral of the entropy density which is defined at a single point. In contrast, the second sum is quadratic in
and it is nonlocal as it depends on the distribution functions
and
defined at different points
and
. This sum does contribute to the elastic constants and the corresponding contribution can be derived substituting the gradient expansion of the distribution function into the equation and collecting terms quadratic in the gradients of the director [Citation34–Citation38]. Thus, all Frank elastic constants appear to be quadratic in the molar fractions
. As a result the elastic constant of a binary mixture of bent-core and calamitic nematic can be approximately expressed as (neglecting the higher terms):
where and
are the molar fractions of bent-core and calamitic molecules, respectively. Here
and
are the elastic constants of pure bent-core and calamitic nematic materials, respectively, and
is determined by interactions between calamitic and bent-core molecules and is generally different from either
or
.
Note that in the case where 10% of bent-core molecules are added, as in our experiments, is of the order of 10−2 and can be neglected unless
is very large. In fact, for these systems, all
are smaller than the corresponding
(except for the splay elastic constant of compound 1). Thus, the last term in Equation (2) can be neglected for all twist and bend elastic constants. Taking into account that
the elastic constants of the mixtures can be expressed in terms of
only. Neglecting again the quadratic terms (in
) one obtains approximately;
Thus, it is the second term in Equation (2) which determines the deviation of the elastic constant of the mixtures from those of the pure calamitic host. Assuming that is of the order of
and
is of the order of 0.1, one concludes that
can be reduced by the order of 10 – 20%, offering a crude estimate of the expected influence of the dopant in our mixtures.
In the above argument, everything depends on which should be sensitive to the structure of both calamitic and bent-core molecules. One may assume that for our systems, the splay constant,
is close in value to
and then the change is small, as seen experimentally. In contrast, for bend, the corresponding difference between
and
may be significant and then one can explain the experimentally observed reduction in K33 of about 15% in all of the mixtures. However, it is hard to explain the 40% reduction of K22 using these arguments. Indeed, all elastic constants are positive and hence, according to Equation (3), the maximum reduction is achieved when
is much smaller than
. If we set
to zero for simplicity, then one obtains
, which results in a reduction of ~20%.
Clearly, some contribution is missing and so we return to the fluctuations in polar and chiral conformers which also cause very low elastic constants of the pure materials [Citation16]. Unfortunately, the corresponding corrections from such contributions are very small as they are proportional to . Further, the bend vector B and the splay vector S induce a local polarisation P and hence there are additional terms in the free energy to be considered [Citation16,Citation39]:
where the last positive term is the dielectric energy. Minimisation with respect to P yields the standard flexoelectric polarisation . Substituting this back one obtains the negative corrections to the elastic constants considered in previous papers, i.e.
.
However, previous papers referred to pure compounds and for this work we need to consider how and
depend on molar fractions.
Consider for simplicity the case of perfect orientational order. In this case, bent-core molecules, which possess only transverse electric and steric dipoles, contribute only to the bend polarisation, i.e. . Polar calamitic molecules contribute only to the splay polarisation and hence
. Thus, one obtains the following corrections to the splay and bend elastic constants;
We are only interested in the corrections which depend on the presence of bent-core molecules, i.e. on . Other corrections are already included in the elastic constants of the pure calamitic material. Note that the negative corrections to both bend and splay constants are quadratic in
and hence they cannot change anything dramatically. Interestingly, the correction to the splay constant, which is linear in
is positive, i.e. it leads to an increase in the elastic constant. Thus, these corrections lead to weaker reduction of the bend constant compared to the second term in Equation (3).
Calculations are similar in the case of the twist constant. A twist deformation induces a small dynamic enantiomeric excess () in bent core conformers which is coupled with the twist (
). Neglecting the interaction between bent-core molecules and the molecules of the host phase, the free energy of the mixture can be expressed as [Citation16];
where is the average number density of bent-core molecules,
and
are the molar fractions of the left and right conformers of the bent-core molecules, respectively,
and
is the helical twisting power. Here
,
is the effective interaction constant between conformers of equal and opposite handedness, respectively, and
is the chiral discrimination energy.
In contrast to our previous paper [Citation16] where a pure bent-core material has been considered, in the present mixture the molar fractions of bent-core conformers are of the order of which is of the order of 0.1. Thus, one may neglect the terms quadratic in
and
in the first approximation, i.e. the terms which describe the interaction between the conformers. Expressing the molar fractions
and
in terms of the dynamic enantiomeric excess (
), i.e.
,
and expanding in terms of
one obtains the following approximate expression for the contribution to the free energy which depends on the enantiomeric excess;
Minimisation of this free energy with respect to yields;
Substituting the expression for the dynamic enantiomeric excess back into the free energy, one obtains the negative correction to the twist constant, where
. It should be noted that the correction to the twist elastic constant is linear in
and hence at small
it is expected to be much larger than the corresponding negative corrections to the splay and bend constants which are quadratic in
. This qualitatively explains why the maximum reduction is observed for the twist constant.
Taking into account only linear terms in the twist constant of the mixture can now be estimated as;
and hence there are two negative corrections to the twist constant which are linear in . Note that in the pure bent-core nematic, the twist elastic constant,
and hence
. The correction
is then less than
. Thus, the last term in Equation (9) can account for at most a 10% reduction of the twist constant and the maximum value of the total negative correction is about 30%. This is already closer to experiment although is still a bit too low.
We suggest that the full explanation lies in an interesting influence of the system on chiral conformers of the host materials. Cyanobiphenyls are known to exist in chiral conformations (twisted about the direct bond between the aromatic rings). The dynamic enantiomeric excess of chiral bent-core conformations may in principle also induce some deracemisation in the biphenyl matrix which will lead to further reduction of the total twist constant. Let us consider this effect in more detail.
Now take into consideration interactions between chiral conformers of bent-core molecules and
and chiral conformers of cyanobiphenyls
and
. Neglecting the quadratic terms in
and
the free energy of the mixture in the presence of twist deformation can be written in the form;
where ,
,
, and
are the interaction constants between bent-core and cyanobiphenyl conformers and
,
, and
are the corresponding interaction constants between cyanobiphenyl conformers. Note that
,
, and
. Here
is the helical twisting power of a chiral cyanobiphenyl conformer.
Using the expressions ,
and
,
, where
is the dynamic enantiomeric excess of cyanobiphenyl conformers, and expanding the free energy in terms of
and
one obtains;
where is independent of
,
and the twist deformation
. Here
and
. Minimisation with respect to
and
yields the following system of simultaneous equations;
Taking into account only terms linear in the solution of Equation (12) can be expressed as;
where ,
and
,
. One notes that the dynamic enantiomeric excess
is given approximately by the same equation as Equation (8) where the coupling with the cyanobiphenyl molecules has not been taken into account. In contrast, the dynamic enantiomeric excess
is induced both by the twist deformation and by the interaction with chiral conformers of the bent-core molecules.
Substituting Equation (13) back into the free energy in Equation (11) and keeping only terms linear in , one obtains the following correction to the twist elastic constant;
where as generally
The first negative correction in Equation (14) is exactly the same as the one obtained earlier taking into account the partial dynamic deracemisation of the system of bent-core molecules in the presence of twist deformation. The second correction in Equation (14) has already been included in the twist elastic constant of the cyanobiphenyl host phase. Finally, the third term describes the additional negative correction (taking into account that generally ) which is determined by a partial dynamic deracemisation in the cyanobiphenyl host induced by chiral conformers of the bent-core molecules. Thus, there are two additional negative corrections to the twist elastic constant of the mixture which are linear in
and which may account for a reduction of the order of 10% each. These corrections are absent in the expressions for the splay and bend elastic constants of the mixture which enables one at least qualitatively explain why the twist constant experiences the largest reduction among all elasticity coefficients.
5. Conclusions
Firstly, we note that our results suggest that there is no relationship between the elastic constant behaviour in the nematic phase and the formation of the filament phase observed in mixtures 4 and 5, reported by Gleeson et al. [Citation25], confirming that the filament phase observed is not a nematic variant, despite the filaments exhibiting nematic-like order. Reductions in the twist and bend elastic constants were measured in all the mixtures, not just those that exhibited the filament phase.
We have shown that the influence of a bent-core dopant on the three elastic constants, K11, K22 and K33 is quite distinct. In the case of the splay constant, in all three mixture sets, the influence of the bent-core dopant is negligible. By considering the expected influence of a dopant on the elastic constants of the mixture, we can draw two conclusions. Firstly, the splay interaction constant, , determined by interactions between calamitic and bent-core molecules, must be negligible. Further, there can be no additional contribution that might emerge from fluctuations in the conformers. Splay is most affected by a wedge-shaped structure; splay instabilities have recently been reported in the nematic phase of wedge-shaped molecules where there are issues with space-filling [Citation24]. There is apparently little scope for the BC molecules included in the mixtures here to show any wedge-shaped conformers that might have a significant impact on the splay elastic constant.
The influence of the bent-core dopants on the bend elastic constant leads to a reduction of 10–20% in K33, in line with the simple mixing rule described by Equation (3) and with existing literature. However, the surprising result of this study was the remarkable reduction of 30–40% observed in the twist elastic constant, K22 of all of the mixtures. We have provided an explanation of this that suggests that not only do the fluctuations resulting in chiral conformers of the bent-core system have a significant effect, but that they must also enhance the fluctuations in chiral conformers of the host calamitic liquid crystal. This surprising result indicates an amplification of fluctuations in chirality of the system which is important both from the technological point of view and from understanding how chirality can ‘cascade’ in a fluid system.
Both the measurements and the explanation of the phenomena suggest how bent-core/calamitic mixtures could be employed to tune mixtures for devices. The effect on all three elastic constants, including the dramatic effect on the twist constant in particular has not previously been reported. As the elastic constants are related to the threshold voltages in devices, the measurements we detail in this paper indicate the potential of these mixtures to lower threshold voltages in devices. Further, the dramatic reduction in the twist elastic constant offers a possible route for designing mixtures that exhibit nematic variant phases such as the twist-bend phase.
Acknowledgments
This work was supported by the Engineering and Physical Sciences Research Council (EP/N509243 and EP/M020584). SS and HL thanks the EPSRC and Merck for funding a studentship.
Disclosure statement
No potential conflict of interest was reported by the authors.
Additional information
Funding
References
- Kaur S, Panov VP, Greco C, et al. Flexoelectricity in an oxadiazole bent-core nematic liquid crystal. Appl Phys Lett. 2014;105:223505.
- Addis J, Kaur S, Binks DJ, et al. Second-harmonic generation and the influence of flexoelectricity in the nematic phases of bent-core oxadiazoles. Liq Cryst. 2016;43:1315–1332.
- Sathyanarayana P, Mathew M, Li Q, et al. Splay bend elasticity of a bent-core nematic liquid crystal. Phys Rev E. 2010;81:010702.
- Majumdar M, Salamon P, Jákli A, et al. Elastic constants and orientational viscosities of a bent-core nematic liquid crystal. Phys Rev E. 2011;83:031701.
- Tadapatri P, Hiremath US, Yelamaggad CV, et al. Permittivity, conductivity, elasticity, and viscosity measurements in the nematic phase of a bent-core liquid crystal. J Phys Chem B. 2010;114:1745–1750.
- Salter PS, Tschierske C, Elston SJ, et al. Flexoelectric measurements of a bent-core nematic liquid crystal. Phys Rev E. 2011;84:031708.
- Balachandran R, Panov VP, Vij JK, et al. Effect of cybotactic clusters on the elastic and flexoelectric properties of bent-core liquid crystals belonging to the same homologous series. Phys Rev E. 2013;88:032503.
- Chakraborty A, Das MK, Das B, et al. Rotational viscosity measurements of bent-core nematogens. Soft Matter. 2013;9:4273–4283.
- Avci N, Borshch V, Sarkar DD, et al. Viscoelasticity, dielectric anisotropy, and birefringence in the nematic phase of three four-ring bent-core liquid crystals with an L-shaped molecular frame. Soft Matter. 2013;9:1066–1075.
- Yang KH. Fréedericksz transition of twisted nematic cells. Appl Phys Lett. 1983;43:171.
- Oh-e M, Kondo K. Electro‐optical characteristics and switching behaviour of the in‐plane switching mode. Appl Phys Lett. 1995;67:3895.
- Dozov I. On the spontaneous symmetry breaking in the mesophases of achiral banana-shaped molecules. Europhys Lett. 2001;56(2):247–253.
- Adlem K, Čopič M, Luckhurst GR, et al. Chemically induced twist-bend nematic liquid crystals, liquid crystal dimers, and negative elastic constants. Phys Rev E. 2013;88:022503.
- Borshch V, Kim Y-K, Xiang J, et al. Nematic twist-bend phase with nanoscale modulation of molecular orientation. Nat Commun. 2013;4:2635.
- Yun C-J, Vengatesan MR, Vij JK, et al. Hierarchical elasticity of bimesogenic liquid crystals with twist-bend nematic phase. Appl Phys Lett. 2015;106:173102.
- Srigengan S, Nagaraj M, Ferrarini A, et al. Anomalously low twist and bend elastic constants in an oxadiazole-based bent-core nematic liquid crystal and its mixtures; contributions of spontaneous chirality and polarity. J Mater Chem C. 2018;6:980–988.
- Kundu B, Pratibha R, Madhusudana NV. Anomalous temperature dependence of elastic constants in the nematic phase of binary mixtures made of rodlike and bent-core molecules. Phys Rev Lett. 2007;99:247802.
- Pratibha R, Madhusudana NV, Sadashiva BK. An orientational transition of bent-core molecules in an anisotropic matrix. Science. 2000;288(5474):2184–2187.
- Sathyanarayana P, Jampani VSR, Skarabot M, et al. Viscoelasticity of ambient-temperature nematic binary mixtures of bent-core and rodlike molecules. Phys Rev E. 2012;85:011702.
- Matraszek J, Mieczkowski J, Szydowska J, et al. Nematic phase formed by banana-shaped molecules. Liq Cryst. 2000;27(3):429–436.
- Parthasarathi S, Rao DSS, Palakurthy NB, et al. Binary system exhibiting the nematic to twist-bend nematic transition: behavior of permittivity and elastic constants. J Phys Chem B. 2016;120(22):5056–5062.
- Chen D, Shen Y, Zhu C, et al. Interface structure of the dark conglomerate liquid crystal phase. Soft Matter. 2011;7:1879–1883.
- Ericksen JL. Inequalities in liquid crystal theory. Phys Fluids. 1966;9:1205.
- Mertelj A, Cmok L, Sebastián N, et al. Splay nematic phase. Phys Rev X. 2018;8:041025.
- Gleeson HF, Liu H, Kaur S, et al. Self-assembling, macroscopically oriented, polymer filaments; a doubly nematic organogel. Soft Matter. 2018;14:9159–9167.
- Chen D, Porada JH, Hooper JB, et al. Chiral heliconical ground state of nanoscale pitch in a nematic liquid crystal of achiral molecular dimers. Proc Natl Acad Sci USA. 2013;110(40):15931–15936.
- Kaur S, Addis J, Greco C, et al. Understanding the distinctive elastic constants in an oxadiazole bent-core nematic liquid crystal. Phys Rev E. 2012;86:041703.
- Kaur S, Liu H, Addis J, et al. The influence of structure on the elastic, optical and dielectric properties of nematic phases formed from bent-core molecules. J Mater Chem C. 2013;1:6667–6676.
- Kaur S, Tian L, Liu H, et al. The elastic and optical properties of a bent-core thiadiazole nematic liquid crystal: the role of the bend angle. J Mater Chem C. 2013;1:2416–2425.
- Bogi A, Faetti S. Elastic, dielectric and optical constants of 4ʹ-pentyl-4-cyanobiphenyl. Liq Cryst. 2001;28(5):729–739.
- Bradshaw MJ, Raynes EP, Bunning JD, et al. The Frank constants of some nematic liquid crystals. J Phys (Fr). 1985;46:1513–1520.
- Cestari M, Ferrarini A. Curvature elasticity of nematic liquid crystals: simply a matter of molecular shape? Insights from atomistic modelling. Soft Matter. 2009;5:3879–3887.
- Earl DJ, Osipov MA, Takezoe H, et al. Induced and spontaneous deracemisation in bent-core liquid crystal phases and in other phases doped with bent-core molecules. Phys Rev E. 2005;71:021706.
- Osipov MA, Pajak G. Effect of polar intermolecular interactions on the elastic constants of bent-core nematics and the origin of the twist-bend phase. Eur Phys J E. 2016;39:45.
- Gelbart WM, Ben-Shaul A. Molecular theory of curvature elasticity in nematic liquids. J Chem Phys. 1982;77:916.
- Somosa AM, Tarazona P. Density functional theory of the elastic constants of a nematic liquid crystal. Mol Phys. 1991;72:911.
- Poniewierski A, Stecki J. Statistical theory of the elastic constants of nematic liquid crystals. Mol Phys. 1979;38:1931.
- Kapanowski A, Sokalski K. Elastic constants of binary liquid crystalline mixtures. Z Naturforsch. 1998;53 a:963–976.
- Shamid SM, Dhakal S, Selinger JV. Statistical mechanics of bend flexoelectricity and the twist-bend phase in bent-core liquid crystals. Phys Rev E. 2013;87:052503.