ABSTRACT
Just as nematic liquid crystals are widespread in display technology, a small number of building blocks find widespread use in the design and synthesis of liquid crystalline materials. This review explores the intricate relationship between the molecular structure of thermotropic liquid crystals (LCs) and their phase behaviour, emphasising the role of specific structural fragments in determining LC properties. We also detail into the impact of non-conventional building blocks on LC properties by comparing families of materials differentiated by only a single structural variation, allowing us to probe the extent to which deviations from traditional structures can maintain liquid crystallinity. This comprehensive overview not only underscores the importance of specific molecular fragments in LC design but also opens avenues for the innovative use of non-traditional building blocks in the development of new LC materials. Just as the ubiquity of 1,4-disubstituted benzene rings has its genesis (partly) in the extremely robust and predictable synthesis of such systems through cross-coupling chemistry; we consider that, the rapid progress in coupling sp3 fragments, coupled with the growing availability of suitable building blocks, makes the inclusion of said fragments ever more practical and attractive for use in liquid crystalline systems.
Introduction
Thermotropic liquid crystalline phases are intimately related to molecular structure [Citation1] Broadly speaking, the structure of thermotropic liquid crystals can be divided into four principal sub-groupings as shown in : terminal chain(s) (i), rigid core unit (ii), linking units (iii), lateral groups (iv) and terminal groups (v). We briefly consider some elementary variations to the nematogen 5CB; increasing the terminal chain leads to an increase in melting and clearing points, as well as a smectic A rather than nematic mesophase. In this system, extending the core unit by addition of a single 1,4-disubstituted benzene leads to dramatic increases in both melt and isotropisation temperatures (, compound 1b). Inclusion of conjugated linking groups such as esters (compound 1c) increases the clearing point, while non-conjugated links typically lead to depression (compound 1d); this is a consequence of both electronic and steric effects, with non-conjugated links (such as methyleneoxy in ) also endowing rather different conformational profiles than their conjugated equivalents. Replacement of 1,4-disubstituted benzene(s) with 1,4-disubstituted cyclohexane(s) gives increased clearing points along with higher melting points and reduced dielectric anisotropies. Although these behaviours for 5CB and its modifications can be applied broadly to thermotropic materials, any structural modification must be considered in the context of the entire molecular structure. While not shown in , typically lateral fluorination leads to a depression of 30–40 K in TNI [Citation9]; it is therefore unsurprising that fluorinated 5CB variants are non-mesogenic [Citation10–14]. The molecular structures of thermotropic liquid crystalline materials is typically constructed from a rather limited set of fragments, a point we will now illustrate. We constructed a database of materials which are reported as exhibiting one or more liquid-crystalline phases. Data were taken from two commercial databases (Reaxys, Scifinder). After removing duplicate entries, we obtained the most common core fragments (), lateral/terminal groups (; excluding alkyl/alkoxy groups which are ubiquitous, being found in >98% of materials) and linking units (). The well-known material 5CB is constructed from some of the most common fragments; two directly bonded 1,4-disubstituted benzene rings, a terminal nitrile, and an alkyl chain.
Figure 1. (Colour online) (a) subdivisions of liquid crystalline structure used in this work: (i) terminal chain; (ii) core-unit; (iii) linking unit; (iv) lateral group; (v) terminal group. Some more common structural variations of 5CB are given, along with their transition temperatures (°C): 1a [Citation2]; 1b [Citation3]; 1c [Citation4]; 1d [Citation5]; 1e [Citation6]; 1f [Citation7]; 1g [Citation8]. Common fragments used in the construction of thermotropic LCs: ring/cyclic fragments (b), lateral/terminal functionality (c) and linking units (d).
![Figure 1. (Colour online) (a) subdivisions of liquid crystalline structure used in this work: (i) terminal chain; (ii) core-unit; (iii) linking unit; (iv) lateral group; (v) terminal group. Some more common structural variations of 5CB are given, along with their transition temperatures (°C): 1a [Citation2]; 1b [Citation3]; 1c [Citation4]; 1d [Citation5]; 1e [Citation6]; 1f [Citation7]; 1g [Citation8]. Common fragments used in the construction of thermotropic LCs: ring/cyclic fragments (b), lateral/terminal functionality (c) and linking units (d).](/cms/asset/4de9adc7-8662-4b40-bfa8-31da2d7e85a2/tlct_a_2297236_f0001_oc.jpg)
Our primary aim with this database was to use the fragments for generative design of new liquid crystalline materials [Citation15], but we realised that we could also examine this for to find which fragments occur most often, and which could be considered unappreciated. As shown in , benzene rings are found in the vast majority of thermotropic liquid crystalline materials. The prevalence of substituted benzenes reflects their utility, and also the typically mild chemistry employed (and predictable reactivity) in the construction of complex molecular architectures. Other cyclic groups, such as those in , are equally adept at generating mesomorphic states and ever-advancing progress in sp2-sp3 and sp3-sp3 cross couplings makes the synthesis of such systems increasingly routine [Citation16–19]. We consider that the increasing ease of synthesis of such units, coupled with the rapidly growing commercial availability of suitable building blocks, makes these increasingly attractive for use in liquid crystalline materials. However, are these any good? What prior art exists for the use of non-conventional building blocks in liquid crystals? How far can we deviate from ‘conventional’ building blocks and still retain liquid crystallinity? These questions prompt us to review the use of non-conventional building blocks in thermotropic liquid crystals. Throughout this review, we introduce families of materials, typically grouped together from multiple sources; the grouping is based on materials being related by a single structural change (e.g. swapping 1,4-disubstituted benzene for a disubstituted acetylene) so as to enable us to make a meaningful comparison between materials.
Variations in terminal-chain length are commonplace and dictate, among other things, the melting point and tendency for nematic/smectic phase formation, as exemplified by the 4-alkyl-4′-cyanobiphenyl family shown in .
Figure 2. (Colour online) Transition temperatures (°C) of the first few members of the 4-alkyl-4’-cyanobiphenyl series. Hashed areas correspond to those below the melting point (monotropic regions).
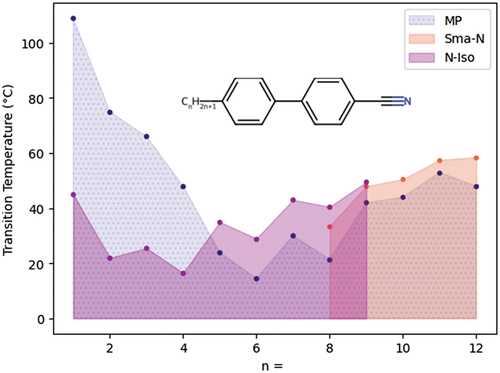
The role of terminal groups is also typically well explored, as illustrated by the family of 4-pentylbiphenyl materials with various polar termini given in . The ability of the parent of this series (5CB, 1) and other nitrile terminated materials to form transient antiparallel pairs is well established [Citation35,Citation36]; this affords a higher clearing point than would be anticipated based on molecular length alone, as the ‘effective’ molecular length is far larger – a point well illustrated by comparing other popular terminal groups (e.g. F, 3; NO2, 7). Polar groups which further extend the molecular length give large increases in clearing point (e.g. cyanoacetylene 8, cyanostilbene 9), whereas the corresponding non-polar equivalents do not (acetylene 4, stilbene 5). Isothiocyanates are generally competent at generating mesophases (e.g. 10), while the equivalent isocyanates (11) are not. Typically carboxylic acids spontaneously dimerise through hydrogen bonding, this effectively doubles the molecular length and hence compounds such as 12 can have seemingly extremely high melting/clearing points. Conversely, amides (such as 13) typically do not show liquid crystallinity in calamitic systems owing to extensive hydrogen bonding between N-H and C=O groups on adjacent molecules; curiously, N-methylation offers a means to circumvent this [Citation37]. Polar ring systems such as N-pyrrole (15) can also be utilised as a means to both extend the rigid core and introduce additional polarity, leading to impressive increases in clearing points. Compact fluorinated termini (16-20) do not lead to anti-parallel dimerisation in the same manner as nitriles and so they typically require a larger rigid core unit in order to exhibit liquid crystalline phases.
Table 1. Transition temperatures (T,°C) of 4-pentylbiphenyl derivatives with various polar groups in the 4’ position. #Compound is reported to be ‘photochemical very unstable’ [Citation25]. $virtual transition temperature extrapolated from mixtures. ##SmE.
This point is well illustrated by the extended core system in . Here, we see that the terminal fluoro substituent is competent at generating liquid crystalline phases and generates a modest positive dielectric anisotropy (22), in contrast to compound 3 encountered earlier. Increasing the polarity of the terminal group generally increases dielectric anisotropy, however as the physical dimensions of the unit increase and the molecular shape deviates from rod-like there is a tendency to depress clearing points significantly (e.g. 30). Attention must be paid to the geometry of the polar unit, for example the N(CH3)(CF3) group (31) has a relatively low dielectric anisotropy as the dipole associated with the N-CF3 group is far off axis. Further to this, the geometry of the gem difluorocyclopropylcarboxylate terminal group (32) is such that the resulting material yields a negative dielectric anisotropy [Citation49].
Table 2. Transition temperatures (°C) of trans trans 1-(4-propylcyclohexyl)cyclohexyl)benzenes with various polar groups in the 4-position. n/r not reported.
Next, we consider a set of materials which derive from 8CB in which the alkyl-bearing benzene ring is replaced with various other ring systems (). Replacement of one benzene ring with pyridine (34) or pyrimidine (35) yields marginally higher clearing points, along with modest increases in dielectric anisotropy [Citation50–52]. Conversely, replacement of the same benzene ring with thiophene (36) depresses the mesomorphic state(s) of 8CB due to its non-linear exit vectors. Similarly, fluorene (37) is somewhat non-linear, however the increased aspect ratio offsets the unfavourable geometric constraints of this unit and results in a large increase in the clearing point. The non-linearity of thiophene can be compensated for through use of 5,6-dihydro-4H-cyclopenta[b]thiophene [Citation64–66] or thieno[3,2-b]thiophene [Citation67].
Table 3. Transition temperatures (T,°C) of analogues of 4-octyl-4′-cyanobiphenyl incorporating various 1,4-disubstituted benzene isosteres.
The use of 1,3-dioxanes (39) is relatively commonplace in liquid crystals; the equivalent 1,2,3-dioxaborolanes (38), 1,3-thiopyrans (40), and 1,3-dithiane (41) are much less common and typically present with fairly significant depression of clearing points. The 1,2,3-dioxaborolanes are conveniently prepared from the corresponding boronic acid and diol and are generally competent at generating mesophases [Citation55]. Liquid crystalline materials containing trans 1,4-cyclohexane (42) are commonplace, and when this unit is introduced in place of a 1,4-benzene ring typically there is a modest increase in melting and clearing point. This effect is even more pronounced with bicyclo[2.2.2]octane (43), itself relatively commonplace in liquid crystals. Other polycyclic alkanes are essentially absent save for bicyclo[1.1.1]pentane (BCP, 45), with a few bicyclo[3.1.0]hexanes (44) [Citation62], bicyclo[3.3.0]octanes [Citation68], bicyclo[2.2.1]heptanes [Citation69], tricycle[4.4.0.03.8]decane [Citation70]. The bicyclo[1.1.1]pentane unit is so compact that the direct benzene-for-BCP swap generally gives non-mesogenic materials owing to the significant reduction in molecular length. However, BCP is useful for extending the rigid core unit resulting in a significant increase in transition temperatures, demonstrated by compound 46.
In fact, materials whose rigid units are entirely of BCP units – so-called [n]staffanes – can exhibit liquid crystalline phases () [Citation71]. This is due to compact nature of the BCP unit, a minimum of three rings is required to exhibit liquid crystallinity, with the resulting materials various exhibiting nematic and highly ordered smectic phases. Further examples of BCP containing liquid crystals are known (for example see [Citation63,Citation72,Citation73])
Table 4. Transition temperatures (°C) and phase assignments of [n]-staffanes [Citation71]. $SmG phase. £SmB phase. #decomposes.
We now consider further ring variations in a series of 4’-cyanobiphenyl 4-pentylcarboxylates (53-66, ). Again, compared to the parent compound the swap of the 1,4-disubstituted benzene ring for trans 1,4-cyclohexane or bicyclo[2.2.2]octane yields the expected changes in mesomorphism. The trans 1,3-dioxane gives a slight reduction in melting and clearing point relative to the parent system; when the dioxane is augmented with either a 5-methyl (59) or 5-cyano (60) group significant depressions in the melting and clearing points are observed. The trans 1,3-cyclopentane (61) exhibits significantly lower transition temperatures than the equivalent cyclohexane due to its non-linearity and non-planarity. Contrast this with the aromatic thiophene derivative (62) which, although non-linear is planar, and while this has somewhat reduced transition temperatures than the parent. However, this change effect is less pronounced than seen earlier for compound 36 () owing to the increased molecular length of the present compound. Although radically different in structure to the present thiophene materials, other liquid crystalline materials with chalcogenophenes bearing a chalogen are known. For example, furans are ubiquitous and so are beyond the scope of this discourse [Citation86–88]. A handful of liquid crystalline 2,5-selenophenes are known [Citation89,Citation90], which is impressive as the olfactory challenges of working with low molecular weight organoselenium compounds are well appreciated [Citation91]. Despite the relentlessly foul nature of organotellurium chemistry [Citation92], the liquid-crystallinity of some 2,5-disubstituted tellurophene derivatives has been (very impressively) explored; they display modestly high birefringence which is comparable to the analogous selenophenes [Citation93]. Examples of benzochalcogenophenes are also known within this context [Citation94–100]. The spirobicyclo[5.5]undecane is one of the number of other materials which have been reported that include this functionality [Citation101], as have additional related spiro-systems such as dispirotetradecanes [Citation102], dispiro[5.1.5.2]pentadecan-7-ones [Citation103], dispiro[5.2.5.2]hexadecanes [Citation104], dispiro[3.2.5.2]tetradecanes [Citation105], and dispiro[2.0.2.1]heptanes [Citation106].
Table 5. Transition temperatures (T/°C) for 4′-cyano[1,1′-biphenyl]4-yl benzoates/carboxylates including various ring systems with a pentyl terminal chain; #chiral nematic phase.
We now focus on a family of 4’-cyanobiphenyl esters containing various ring structures with a propyl terminal chain (). 2-Alkylcyclobutane carboxylic acids are readily synthetically accessible in both cis and trans configurations via alkylation/decarboxylation of diethylmalonate with 2-alkyl-1,3-dibromopropane [Citation109], and these can be used as drop-in replacements for the benzene ring, albeit with reduced (significantly in the case of cis, 67) clearing points. Trans cyclopentane is a less effective isostere as it deviates significantly from linearity (70). Spiro[3.3]heptane (71) and dispiro[3.1.3.1]decane (72) preserve the linear exit vectors and are trivially prepared via the same alkylation/decarboxylation of malonate esters and make competent 1,4-benzene isosteres. Perhydroazulene has a more complex synthesis [Citation108], yet offers a higher clearing point (and lower melting point) than the corresponding 1,4-benzene (73).
Table 6. Transition temperatures (°C) for 4′-cyano[1,1′-biphenyl]4-yl benzoates/carboxylates including various ring systems with a propy terminal chain.
We shift focus now to a closely related set 4’-propylbiphenyl esters containing various ring structures with a second propyl terminal chain (). The behaviour of the trans 1,4-cyclohexane (75) and bicyclo[2.2.2]octane (76) derivatives mirrors that discussed elsewhere (e.g. compounds 55, 56). The cubane containing material 77 displays a markedly reduced melting point relative to the parent system. Whereas a modest number of cubane-containing liquid crystals are known [Citation110–113], derivatives of cubane such as the tetracyclo[4.2.0.02,5.03,8]octane system (78, obtained by partial hydrogenolysis of the parent cubane) are extremely uncommon and appear to be limited to ref [Citation107] only.
Table 7. Transition temperatures (°C) for 4′-propyl[1,1′-biphenyl]4-yl benzoates/carboxylates including various ring systems with a propy terminal chain.
Regrettably, the parent material (compound 79) of the series presented in has no reported clearing point but, given the high-reported melt, it may be non-mesogenic. The 2,6-disubstituted cunenane (pentacyclo[3.3.0.02,4.03,7.06,8]octane (80) is readily prepared via isomerisation of the parent cubane over palladium or silver salts [Citation118], and in this system it exhibits nematic and smectic A phase with significantly reduced melting points relative to the parent owing to its non-coplanar exit vectors. Recent improved synthetic routes promise to enable the synthesis of asymmetrically substituted 1,4-cunenanes which are of some relevance to liquid crystalline materials [Citation119,Citation120]. Further examples of liquid crystalline cubanes and cunanes are known within the context of liquid crystalline dimers [Citation113]. The analogous piperazine (81) exhibits a smectic B phase. The 1,n-diethenylcycloalkanones (83-85) variously exhibit nematic and SmA phases; as the cycloalkanone unit increases in size the system becomes less linear, and so the melting and clearing points decrease.
Table 8. Transition temperatures (°C) for rod-like liquid crystals with various central units flanked by 4-hexyloxybenzene. n/r = not reported.
In the next family of materials () we focus on heterocyclic rings within the same core structure introduced in . Incorporating pyrazole changes the mesomorphic behaviour of the materials now exhibiting a smectic A rather than nematic phase and a significantly higher clearing point (87). Complexation of this compound with RhCl(CO)2 yields a non-mesogenic complex (88). Compared to pyrazole, the isoxazole (89) leads to generally lower transition temperatures. The analogous 1,3,4-oxadiazole (90) has rather non-linear exit vectors (~130°) and so is non-mesogenic, whereas the more linear 1,3,4-thiaxiazole (91) exhibits the same phase sequence as the isoxazole. The thiazole 93 is non-mesogenic, and whilst the bis thiazole (94) behaves comparably to the bis oxadiazole (92), the bis thiadiazole (95) exhibits dramatically higher transition temperatures. While the majority of heterocyclic materials in exhibits nematic phases, the N-amino 1,2,4-triazole (96) and pyrimidine (97) materials do not exhibit a nematic phase but rather smectic C and A phases. The closely related pyrazine compound exhibits a rather different phase sequence (Cr 94 SmG 139 SmF 165 SmI 258 Iso) [Citation130] and is left out of for brevity.
Table 9. Transition temperatures (°C) of … . #identified as a B2 phase. $Identified as a SmB phase. *Unidentified smectic phase (SmX) on cooling to 164.7°C.
Finally, remaining with the same core system, we now consider some examples of fused and non-conventional ring systems (). The diamantane (98, elsewhere referred to as [Citation9]diamondoid and congressane) exhibits a dramatic increase in clearing point owing to the highly rigid structure of this elongated fused ring system. The αα’-substituted succinic acid bis-(enol-lactone) 99 is non mesogenic. The ‘trioxane’ (100, 1,3,5-trioxadecalin) is chiral, and the resulting material exhibits cholesteric and smectic A phases. Conversely, the ‘tetraoxane’ (101, 1,3,5,7-tetraoxadecalin) unit exhibits only a SmA phase with a modest increase in clearing point. The para substituted [2.2]paracyclophane (102) was prepared in its (R) form via chiral resolution techniques of the (S)-phenylethylamine diastereomeric salt [Citation135]; the material exhibits a wide temperature range chiral nematic phase; with other mesogenic units appended to the same planar chiral core variously exhibit chiral nematic and smectic A mesophases.
Table 10. Transition temperatures (°C) of some uncommon ring systems. #chiral nematic phase.
We now consider further non-conventional ring systems in a similar core system, this time with two ester linkages (). Compared to the parent system derived from hydroquinone (103), the 1,4-dioxane (104) and 1,4-dithiane (105) systems offer inferior clearing points and comparable melting points. The tropolone (106) displays an impressively high clearing point considering its non-linearity which is possibly related to [1,9]-sigmatropic rearrangement of the ester and the oxygen atom bound to the C-2 carbon (vide infra). The isorbides (107 and 108) are both non mesogenic yet are often employed as chiral additives [Citation146]. The biphenylene containing 109 exhibits an impressively high clearing point, with a modest increase in melt compared to the parent system, with other examples of this system being known [Citation147]. The 2,5-disubstituted-3,6-dichloroquinone (110) has a notably lower clearing point than the parent system. Lastly, the dimeric-type material 111 exhibits nematic, twist-bend nematic and smectic phases.
Table 11. Transition temperatures (°C) of some uncommon ring systems.
We conclude our focus on ring-systems by considering the same basic structure outlined in , but utilising alkynyl linking groups. Thiophene (113), thiazole (114) mirror behaviour seen elsewhere (e.g. compounds 36, 62, 93) and are generally inferior to the parent 1,4-disubstituted benzene (112). The quinoxaline (115) retains the nematic phase of the parent, despite its protrusion away from the molecular long axis. A similar effect is observed for the triptycenyl compound 116 (albeit monotropically).
Table 12. Transition temperatures (°C) of some uncommon ring systems.
Linking groups
We now focus on various linking groups and their effects on transition temperatures (see ). Arguably the most common linking unit is a direct bond, however esters and imines are commonplace due to their ease of synthesis and favourable interactions which support liquid crystalline order. Here, we define a linking group as a non-cyclic group which adjoins one or more ring systems. Mono-, di- and tri-acetylenes (117–119), extend the length of the rigid core unit and increase TNI and give very large birefringence values, albeit the chemo- and photo-stability of the materials is expected to be not that high. The cyanoethylene (123) exhibits nematic and smectic A phases, with the cyano moiety generating a large electric dipole moment. The analogous 1,2-substituted prop-1-ene linking unit (124) also sustains liquid crystalline order, whereas the 3,4-substituted hex-3-ene does not, on account of the large steric bulk of the two protruding – C2H5 groups. Azo-linkages are commonplace (e.g. 122); and the less common but the closely related azine (127) and ethane-1,2-diimine (129) groups are also shown to be able to generate liquid crystalline phases. Longer homologues of the propan-1,3-dione linked material (130) show various smectic mesophases [Citation162]. The analogous (Z)-aminopropenone material is also known and displays a number of unidentified mesophases [Citation170]. Oxime-esters (131, 132) and hydrazides (134, 135) are capable of generating liquid crystalline phases yet are not frequently encountered as linking groups. Homologues of the tetraketonate (138) [Citation169] with longer terminal-chain lengths exhibit columnar mesophases, however, the resultant complexes with boron trifluoride are non-mesogenic irrespective of terminal-chain length however. Other miscellaneous uncommon linking groups capable of supporting liquid crystalline order include selenoesters [Citation171], and trans tetrafluorosulfanyl [Citation172].
Table 13. Transition temperatures (T,°C) of various linking groups employed between the two 1,4-benzene rings of the 4,4′-hexyloxybiphenyl system. #SmB phase. $SmC phase. *not reported.
Terminal groups
We now focus on groups appended at the terminus of an alkyl chain in a 5-decyloxy-2-phenylpyrimidine liquid crystal (). Replacement of the terminal – C2H5 of the parent compound with tbutyl (140), trimethylsilyl (141) or trimethylgermyl (142) retains the smectic C phase with a slight reduction in clearing point accompanying a more pronounced drop in melting point. Using the bulkier triethylsilyl (143) or triethylgermyl (144) groups gives an even more pronounced depression. The heptamethyltrisiloxane terminated 145 experiences less of a depression in melting point and clearing point, while the cyclopentyl terminated 146 actually has a higher melt than the parent. Other cyclic units employed in pyrimidine containing liquid crystals, such as dispiro[2.0.2.1]heptane derivatives [Citation106], or various cyclic hydrocarbons in the 4-cyanobiphenyl system [Citation177].
Table 14. Transition temperatures (T,°C) of various groups appended at the terminus of one alkyl chain in a series of 5-decyloxy-2-phenylpyrimidine liquid crystals.
The nCB and nOCB liquid crystals remain arguably the most widely used core-type, and so unsurprisingly there are many known structures in which various terminal groups have been installed at the end of the alkoxy chain (). Generally, substituents which promote nanophase segregation (e.g. siloxanes, silanes etc., 154–160) retain the SmA phase of the parent system while other groups instead form a nematic phase (148-153). Generally, the larger the bulk of the terminal group the bigger the depression in clearing point (e.g. the halogen series, 150 – 152). Groups capable of hydrogen bonding typically display higher clearing points than the parent (148, 153, 163). The effect of hydrogen bonding is well demonstrated by comparing the aldehyde 162 to the equivalent carboxylic acid 163. Lastly, the 2,5-dimethylbenzene terminal group (164) can be considered to be ‘half’ of the [2.2]paracyclophanyl group (in 164); both exhibit comparable clearing points, but the steric bulk of the cyclophane prevents the formation of smectic phases in the latter.
Table 15. Transition temperatures (T,°C) of various groups appended at the terminus of the alkyl chain in 4-cyano-4′-undecyloxybiphenyl.
Fluorination
Fluorination of the rigid core aromatic unit is commonplace in liquid crystals with ~17% of materials bearing at least one fluoro substituent on an aromatic ring, and so falls outside our focus of ‘uncommon’ structural motifs and the reader is referred elsewhere [Citation189]. Likewise, a significant number of materials with perfluorinated (or semi-fluorinated) alkyl chains are relatively commonplace [Citation190]. Fluorination of the non-aromatic portions of the core is far less common, and this is our initial focus here ().
Table 16. Transition temperatures (°C) of various polar non-aromatic ring systems.
While the cyclohexan-2-one motif is non mesogenic (166), replacement with gem difluorocyclohexane (167) or 2-fluorocyclohex-2-ene (168) afforded relatively wide temperature nematic phases [Citation191]. The 5,5,6,6-tetrafluorocyclohexa-1,3-diene derived 169 has an impressively large negative dielectric anisotropy of −7.3 (extrapolated from mixtures with MLC-6608) [Citation192]. Further examples of liquid crystalline gem difluorocyclohexanes are known, both with the fluorines acting as a lateral group (as above) [Citation193,Citation194] or as a terminal group [Citation195]. In a similar vein, liquid crystalline gem difluorobicyclo[2.2.2]octanes are known [Citation196], while the monofluoro bicyclo[2.2.2]octanes are known outside of the context of liquid crystals [Citation197].
Axial fluorination on cyclohexanes is generally detrimental to the liquid crystalline phase, although it can be used to generate impressively large values of dielectric anisotropy (e.g. 171 and 173 in ). Facially fluorinated cyclohexane derivatives such as 174-177 were found to be non-mesogenic, with fairly small values of dielectric anisotropy (). Other axially fluorinated cyclohexanes, such as 178, while attractive in terms of wide nematic-phase range and low rotational viscosity [Citation198], have the unfortunate propensity to spontaneously and autocatalytically eliminate HF. The tri- and tetrafluoroindanes (178, 179) have impressive negative dielectric anisotropies, but also suffer the same issue of HF elimination [Citation198,Citation200]. The propensity for elimination is not seen in the analogous cyclohexanes bearing an axial cyano group [Citation8].
Table 17. Transition temperatures (°C) and extrapolated dielectric anisotropies of CG-3-N and CU-3-N, with and without axial fluorination.
Table 18. Melting points (°C) of facially fluorinated cyclohexane (174-177), axially monofluorinated cyclohexane (178), and difluoroindane (178-179) derivatives. * has the phase sequence cr 75 SmB 94 iso, Δn of 0.054.
Fluorinated links
Turning now to explore the role that the linking group has not only on transition temperatures but also dielectric anisotropy (). The parent material 181 has a direct link between the ‘central’ trans 1,4-cyclohexane and the 3,4,5-trifluorobenzene motif. A dimethylene space (CH2CH2, 182) reduces transition temperatures and also dielectric anisotropy relative to the parent owing to the increased conformational freedom. The carboxylate ester 183 has a comparable clearing point to the methyleneoxy 184, and a notably larger dielectric anisotropy. The monofluoromethyleneoxy bridged 185 suffers a modest reduction in clearing point, whereas the difluoromethyleneoxy bridge (186) has a beneficial effect on transition temperatures as well as making a significant contribution to dielectric anisotropy which is of a similar magnitude to that of the ester bridged 183.
Table 19. Transition temperatures (T,°C) of trans trans 4-(4-propylcyclohexyl)cyclohexyl liquid crystals with various (fluorinated) linking groups.
Unconventional chiral systems
Further to the chiral systems already discussed, we now focus on more ‘exocit’ chiral moieties which have been shown to promote, or at least tolerate liquid crystallinity (). The C3-symmetric (S,S,S) tricyclo[2.2.1.02,6]heptane-3,5,7-triol, functionalised with trans 4-(4-pentylcyclohexyl)benzoic acid (187), showed a helical twisting power of up to 25 um−1 [Citation206]. Chiral cyclohexylideneethanones (188) were prepared via asymmetric coupling via a chiral sulphoxide and are one of the earliest examples of axial chirality in an optically active liquid crystal [Citation207]. Axially chiral allenes, appended to a thiadiazole bent-core system (189, 190), were shown to generate SmC*, N* and blue phases [Citation208].
Figure 3. (Colour online) Transition temperatures (°C, where available) and helical twisting powers (HTP, where available) for a selection of point, axial, planar, and helically chiral liquid crystalline materials.
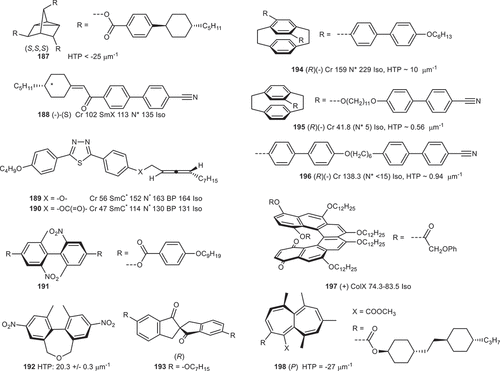
Biphenyls substituted with bulky nitro/methyl groups to block rotation about the central bond and are atropisomeric [Citation209,Citation210], such as 191 in . Related axially chiral biphenyls with a – CH2OCH2- bridge have been reported and exhibit a cholesteric phase [Citation211]; the helical twisting power of such compounds depends strongly upon substituents present, but values as high as 20.3 um−1 were reported for 192 shown in [Citation212]. A variety of alternate bridging groups have been reported, including amides, fused ring systems, 2,3-diaminobutyl [Citation213,Citation214]. Another notable demonstration of axially chirality is the 2,2’-spirobiindan-1,1’-dones [Citation215,Citation216]. These materials show large ferroelectric polarisations in the SmC* phase, for example 193 displays a PS of circa 1 uC cm2 in NCB76 [Citation216]. As mentioned briefly in the discussion around the paracyclophanyl compound in , systems with dissymetrically substituted rings which are non-coplanar can exhibit so-called planar chirality. One prominent example is [2.2]paracyclophane; however, other systems have been shown within the context of liquid crystals (e.g. ferrocenes [Citation217] and azobenzenophanes [Citation218]). When the paracyclophane unit is in the centre of the rigid portion of the mesogen then the HTP values are modest (194) [Citation135], however when relegated to the periphery via flexible chains or via appending to one side of a dimer the resulting HTP values are rather low (195, 196) [Citation219]. Helicenes are polycyclic aromatic compounds in which the regular annulation of aromatic rings gives rise to a helical structure. Beyond molecular aesthetics, the helicene derivative 197 has been shown to exhibit a columnar phase at ambient temperature [Citation220–222]. Heptalenes are dissymmetric molecules with highly twisted symmetric C2 structure and lack a stereogenic centre. It was shown that for a series of chiral heptalene derivatives the position of the substituent groups on the heptalene core dictates the chiral sense of the helical twist (e.g. 198) [Citation223].
One (or less) ring systems
The vast majority of thermotropic liquid crystalline materials have two or more rigid cyclic groups. Typically, multiple cyclic groups (either directly bonded, fused, or with linking groups) are required to retain the requisite molecular shape for liquid crystallinity. As such, it is very uncommon to find mesogenic material with one, or even no, rings. That is not to say that there are no reported examples () Perhaps the most well-known examples of ‘single ring’ mesogens are the alkylbenzoic acids [Citation224,Citation225]. Of course, these benzoic acid derivatives readily dimerise through hydrogen bonding can we consider them true single-ring systems? Typically multiple cyclic groups (either directly bonded, fused, or with linking groups) are required to retain the requisite molecular shape for liquid crystallinity.
Figure 4. (Colour online) Further examples of non-conventional structures exhibiting liquid crystalline behaviour.
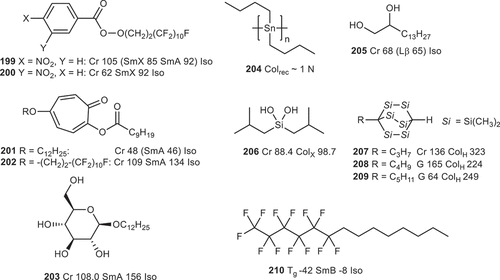
Despite having only a single ring, nitrobenzoates with fluorocarbon tails exhibit smectic phases (199, 200), as the steric clash between fluorine atoms doubles the energy difference between trans and gauche conformers relative to the parent hydrocarbon system [Citation226–229]. 2,5-Disubstituted tropones were shown to be able to generate smectic A mesophases (201, 202) [Citation230–232], a perfluorocarbon chain gives higher transition temperatures than the analogous hydrocarbon in this system. The liquid crystallinity of the 2,5-disubstituted tropone results from a [Citation1,Citation3]-sigmatropic rearrangement of the acyl group and the oxygen atom bound to the C-2 carbon gives a transient ring system which gives a time-averaged linear molecular structure. Synthesis of non- [Citation1,Citation3]sigmatropic systems supports this conclusion [Citation233]. Poly dialkylstannanes (e.g. 204) were shown to be liquid crystalline, variously exhibiting nematic, lamellar and rectangular columnar phases depending on the length of the pendant alkyl chains [Citation234]. Diisobutylsilane-diol [Citation235] forms a columnar structure through hydrogen bonding between adjacent silanol groups (206). A family of 1-alkyl-2,3,5,6,7,8-hexasilabicyclo[2.2.2]octanes were found to exhibit columnar mesophases despite having only a single rigid unit (207-209) [Citation236], with the steric bulk of the (many) dimethylsilyl motifs driving the formation of a hexagonal columnar structure via shape segregation. Fluorocarbons can also confer ‘rigidity’ and generate liquid crystalline mesophases in systems that lack any ring systems, for example 210 in [Citation40,Citation237–240].
Conclusions and outlook
In this review, we have explored the use of non-traditional building blocks in liquid crystals and although these units are rarely encountered (and sometimes entirely novel), in many cases these follow expected patterns of behaviour. However, the context of the entire molecule is important when considering the impact of such changes. In the most general terms, the more anisotropic (i.e. longer) the rigid core unit the more forgiving it tends to be in terms of generating liquid crystalline phases with ‘unfavourable’ groups. We suspect that any ring system (or even relatively rigid group of bonded atoms) that reasonably approximates the linear exit vectors of 1,4-disubstituted benzene will probably have some capacity for generating liquid crystalline order, and this is supported by examples herein.
When encountering bulky groups appended at the end of a terminal chain () it is tempting to conclude that it is simply the size of the group that dictates the mesogenic behaviour (e.g. compounds 147-159), however this overlooks subtle effects that can result from hydrogen bonding (e.g. 147, 160, 163), to give one example. Ultimately, these subtle interactions between many molecules are responsible for the genesis of liquid crystalline order; to consider only shape or polarity in isolation is not typically sufficient (e.g. ; various entries). Again, the context of the entire molecule is again relevant – several of the polar terminal units in that yield non-mesogenic materials are shown to be competent at generating nematic phases in (e.g. SCF3) when utilising a different core system.
Metal catalysed cross-coupling reactions are as essential to the synthetic chemist as a hammer and nails and a saw are to a carpenter. With the advent of new synthetic methodology that greatly simplifies sp3-sp3 couplings, these reactions can reliably be used in the design of liquid crystalline materials; specifically, complex ring systems can be installed as entire fragments rather than assembled step-by-step as in the past. For example, a large number of bicyclo[x.y.z]alkanes are available as the mono methyl esters of their dicarboxylic acids; through decarboxylative couplings these could be expanded into fully fledged rod-like liquid crystals. We performed basic geometric analysis on a selection of bicyclo[x.y.z]alkanes (). This demonstrates that some (e.g. bicyclo[3.3.2]nonane) are probably sufficiently close to linear that they would suffice as a drop-in replacement for 1,4-benzene.
Figure 5. (Colour online) Geometrical properties of some 1,4-benzene isosteres, computed for geometry optimised at the B3LYP/6-31G(d) level of DFT shown in order of decreasing linearity.
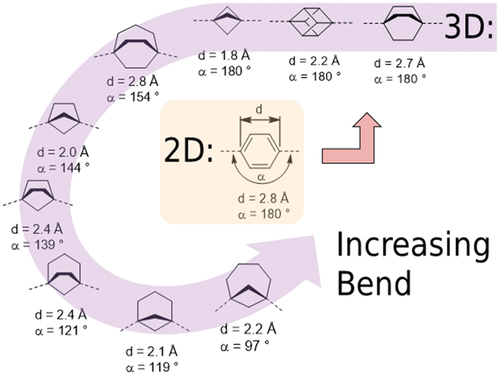
Just as sp2-sp3 and sp3-sp3 couplings are becoming routine, mild fluorination of sp3 carbon is a rapidly growing area. We suspect that direct and targeted fluorination of such 3D benzene isosteres is not only possible, but may be advantageous over ‘2D’ isosteres such as axially fluorinated cyclohexanes. Several examples of cubane-based liquid crystal have been discussed here, with more examples in the literature. Cubane is one of the platonic hydrocarbons, and of the other possible platonic hydrocarbons it seems probable dodecahedrane could be incorporated into a linear motif and coaxed into exhibiting liquid crystalline phases, although the 23-step synthesis presents a rather high barrier to this undertaking [Citation241]
Lastly, so far we have largely restricted ourselves to discussion of materials evaluated experimentally after synthesis. Separate to this, computer hardware and software offer ever increasing performance, which allows us the luxury of probing molecular interactions in atomistic detail through a computational lens. One especially attractive area for the future is in building molecules not by targeting properties by study of prior art, but through specific design of intermolecular molecular interactions. For example, four-centre heteroleptic dipole–dipole interactions between nitriles and sulphoxides [Citation242].
Acknowledgments
Computational work was undertaken on ARC3 and ARC4, both part of the high-performance computing facilities at the University of Leeds, UK. RJM gratefully acknowledges funding from UK Research and Innovation (UKRI) via a Future Leaders Fellowship (grant no. MR/W006391/1), from Merck, and from the University of Leeds via a University Academic Fellowship.
Disclosure statement
No potential conflict of interest was reported by the author(s).
Correction Statement
This article has been corrected with minor changes. These changes do not impact the academic content of the article.
Additional information
Funding
References
- Goodby JW, Davis EJ, Mandle RJ, et al. Nano-segregation and directed self-assembly in the formation of functional liquid crystals. Isr J Chem. 2012;52(10):863–880. doi: 10.1002/ijch.201200059
- Daniel MF, Lettington OC, Small SM. Langmuir-blodgett films of amphiphiles with cyano headgroups. Mol Cryst Liq Cryst. 1983;96(1):373–385. doi: 10.1080/00268948308074719
- Klämke W, Fan ZX, Haase W, et al. An experimental setup for x-ray investigations on higher ordered liquid crystals. Berichte der Bunsengesellschaft für Physikalische Chemie. 1989;93(4):478–482. doi: 10.1002/bbpc.19890930410
- Kelly SM. Some novel nematic 4-cyanophenyl esters incorporating a lateral substituent. J Chem Soc Chem Commun. 1983;1983(7):366–367. doi: 10.1039/c39830000366
- Carrt N, Gray GW. The preparation and liquid crystal properties of materials incorporating a mono-substituted ring system. Liq Cryst. 1989;6(4):467–480. doi: 10.1080/02678298908034191
- Bezborodov VS, Bubel ON, Konovalov VA, et al. Synthesis of trans-4-alkyl-1-phenylcyclohexanes and their derivatives. Zh Org Khim+. 1983;19:1669–1674. doi: 10.1002/chin.198351197
- Bradshaw MJ, Raynes EP. Electric permittivities and elastic constants of the cyano bi-cyclohexanes (cch). Mol Cryst Liq Cryst. 1981;72(2–3):35–42. doi: 10.1080/01406568108084034
- Eidenschink R, Haas G, Römer M, et al. Liquid-crystalline 4-bicyclohexylcarbonitriles with extraordinary physical properties. Angew Chem Int Ed Engl. 1984;23(2):147–147. doi: 10.1002/anie.198401471
- Kirsch P, Bremer M. Nematic liquid crystals for active matrix displays: molecular design and synthesis. Angew Chem Int Ed. 2000;39(23):4216–4235. doi:10.1002/1521-3773(20001201)39:23<4216::AID-ANIE4216>3.0.CO;2-K
- Fearon JE, Gray GW, Ifill AD, et al. The effect of lateral fluorosubstitution on the liquid crystalline properties of some 4-n-alkyl-, 4-n-alkoxy- and related 4-substituted-4’ -cyanobiphenyls. Mol Cryst Liq Cryst. 1985;124(1):89–103. doi: 10.1080/00268948508079467
- Szilvási T, Roling LT, Yu H, et al. Design of chemoresponsive liquid crystals through integration of computational chemistry and experimental studies. Chem Mater. 2017;29(8):3563–3571. doi: 10.1021/acs.chemmater.6b05430
- Hird M, Toyne KJ, Goodby JW, et al. Synthesis, mesomorphic behaviour and optical anisotropy of some novel materials for nematic mixtures of high birefringence. J Mater Chem. 2004;14:1731–1743. doi: 10.1039/b400630e
- Field LD, Hambley TW, Pierens GK. Synthesis and properties of partially fluorinated 4-alkyl-4’-cyanobiphenyls - part ii 4-alkyl-4’-cyano-2‘3’5‘6’-tetrafluorobiphenyls. Tetrahedron. 1990;46(20):7069–7080.
- Field LD, Pierens GK. Synthesis and properties of partially fluorinated 4-alkyl-4’-cyanobiphenyls - part 1. 4’-alkyl-4-cyano-2‘3’5‘6’-tetrafluorobiphenyls. Tetrahedron. 1990;46(20):7059–7068.
- Mandle RJ. Automated flow synthesis of algorithmically designed ferroelectric nematogens. Liq Cryst. 2023;50(3):534–542. doi: 10.1080/02678292.2023.2208550
- Prier CK, Rankic DA, MacMillan DWC. Visible light photoredox catalysis with transition metal complexes: applications in organic synthesis. Chem Rev. 2013;113:5322–5363. doi: 10.1021/cr300503r
- Yan M, Kawamata Y, Baran PS. Synthetic organic electrochemical methods since 2000: on the verge of a renaissance. Chem Rev. 2017;117(21):13230–13319. doi: 10.1021/acs.chemrev.7b00397
- Do J-L, Friščić T. Mechanochemistry: a force of synthesis. ACS Central Sci. 2017;3(1):13–19. doi: 10.1021/acscentsci.6b00277
- Plutschack MB, Pieber B, Gilmore K, et al. The hitchhiker’s guide to flow chemistry. Chem Rev. 2017;117(18):11796–11893. doi: 10.1021/acs.chemrev.7b00183
- Bailey AL, Bates GS. Synthesis of isocyano and (haloalkynyl)biphenyls: new thermotropic liquid crystals. Mol Cryst Liq Cryst. 1991;198(1):417–428. doi: 10.1080/00268949108033417
- Dabrowski R, Ytyńki E. Mesomorphic properties of 4-n-pentylbiphenyl derivatives. Mol Cryst Liq Cryst. 1982;87:109–135. doi: 10.1080/00268948208083777
- Ruolene YI, Adomenas PV, Sirutkaitis RA, et al. Synthesis of 4-alkyl-4’-cyanodiphenyls. Zh Org Khim+. 1984;20:1305–1310.
- Kobr L, Zhao K, Shen Y, et al. Inclusion compound based approach to arrays of artificial dipolar molecular rotors: bulk inclusions. J Org Chem. 2013;78:1768–1777. doi: 10.1021/jo3009897
- Petrzilka M. Polar acetylenic liquid crystals with broad mesomorphic ranges. The positional influence of different c, c-elements on the transition temperatures. Mol Cryst Liq Cryst. 1984;111(3–4):329–346. doi: 10.1080/00268948408072442
- Coates D, Gray GW. Effect of light on the liquid crystal transition temperatures of 4-(4-n-pentylphenyl)vinyl cyanide. J Chem Soc Chem Commun. 1975;1975(13):514–515. doi: 10.1039/c39750000514
- Czupryński K. Phase diagrams of mixtures consisting of polar compounds se and sad. Mol Cryst Liq Cryst Incorporating Nonlinear Opt. 1990;192(1):47–52. doi: 10.1080/00268949008035604
- Petrov VF, Shimizu Y. (Iso)thiocyanato substitution in calamitic liquid crystals. Mol Cryst Liq Cryst. 2001;363(1):107–121. doi: 10.1080/10587250108025262
- Kelly SM, Huynh-Ba T. Some novel nematic materials bearing two lateral nitrile functions. Helv Chim Acta. 1983;66(6):1850–1859. doi: 10.1002/hlca.19830660625
- Macmillan JH, Labes MM. Low transition temperature liquid crystalline amines incorporating the biphenyl ring system. Mol Cryst Liq Cryst. 1979;56(2):51–56. doi: 10.1080/01406567908071966
- Osman MA, Huynh-Ba T. Nematogens for matrix-addressed twisted nematic displays, ii. Z Naturforsch B. 1983;38(10):1221–1226. doi: 10.1515/znb-1983-1010
- Melamed D, Nuckols C, Anne Fox M. Synthesis and electrochemistry of a liquid crystalline pyrrole and a structurally related thiophene. Tetrahedron Lett. 1994;35(45):8329–8332.
- Kelly SM, Skelton G, Jones C, et al. Nematic liquid crystals with a trifluoromethyl group. Mol Cryst Liq Cryst. 2001;364(1):873–880. doi: 10.1080/10587250108025060
- Bartmann E, Dorsch D, Finkenzeller U. Liquid crystals with polar substituents containing fluorine: synthesis and physical properties. Mol Cryst Liq Cryst. 1991;204(1):77–89. doi: 10.1080/00268949108046596
- Januszko A, Glab KL, Kaszynski P, et al. The effect of carborane, bicyclo[2.2.2]octane and benzene on mesogenic and dielectric properties of laterally fluorinated three-ring mesogens. J Mater Chem. 2006;16(31):3183–3192. doi: 10.1039/b600068a
- Gray GW, Harrison KJ, Nash JA. New family of nematic liquid crystals for displays. Electron Lett. 1973;9(6):130–131. doi: 10.1049/el:19730096
- Leadbetter AJ, Richardson RM, Colling CN. The structure of a number of nematogens. J Phys Colloques. 1975;36(C1):C1–43. doi: 10.1051/jphyscol:1975105
- Strachan GJ, Zattarin A, Storey JMD, et al. Tailoring amide n-substitution to direct liquid crystallinity in benzanilide-based dimers. J Mol Liq. 2023;384:122160. doi: 10.1016/j.molliq.2023.122160
- Bartmann E. Flüssigkristalle mit fluorhaltigen alkylgruppen. Berichte der Bunsengesellschaft für Physikalische Chemie. 1993;97(10):1349–1354. doi: 10.1002/bbpc.19930971027
- Gotoh Y, Kubo T Compounds having a difluorocyclohexane ring, liquid crystal compositions and liquid crystal display devices. US 2017/0240809 A1. 2017 Feb 22.
- Kirsch P. Fluorine in liquid crystal design for display applications. J Fluorine Chem. 2015;177:29–36. doi: 10.1016/j.jfluchem.2015.01.007
- Haldar S, Barman S, Mandal PK, et al. Influence of molecular core structure and chain length on the physical properties of nematogenic fluorobenzene derivatives. Mol Cryst Liq Cryst. 2010;528(1):81–95. doi: 10.1080/15421406.2010.504617
- Pavluchenko AI, Smirnova NI, Petrov VF, et al. Synthesis and properties of liquid-crystals with fluorinated terminal substituents. Mol Cryst Liq Cryst. 1991;209:225–235. doi: 10.1080/00268949108036197
- Kanie K, Takehara S, Hiyama T. A facile synthesis of novel liquid crystalline materials having a trifluoromethoxy group and their electro-optical properties. Bull Chem Soc J. 2000;73(8):1875–1892. doi: 10.1246/bcsj.73.1875
- Pavluchenko AI, Smirnova NI, Petrov VF, et al. Synthesis and properties of new halogenated liquid crystals. Mol Cryst Liq Cryst. 1995;265(1):41–45. doi: 10.1080/10587259508041676
- Poetsch Eike DR, Hittich Reinhard DR, Bartmann Ekkehard DR, et al. New fluoro-benzene derivs. - useful as components of liq. Crystal media for lc and electro=optical display elements. DE 4142519 A1. 1991 Dec 21. 1992.
- Miyazawa K, Goto Y, Matsui S, et al. Novel liquid crystalline compound having a 1,1,2,3,3,3,-hexafluoropropyl group and liquid crystal composition. EP 0640578 A1. 1994 Aug 30. 1995.
- Kirsch P, Bremer M, Heckmeier M, et al. Liquid crystals based on hypervalent sulfur fluorides: pentafluorosulfuranyl as polar terminal group. Angewandte Chemie. 1999;38(13–14):1989–1992. doi: 10.1002/(SICI)1521-3773
- Kanie K, Mizuno K, Kuroboshi M, et al. Syntheses and properties of novel liquid crystals containing a trifluoromethylamino group. Bull Chem Soc J. 1999;72(11):2523–2535. doi: 10.1246/bcsj.72.2523
- Miyazawa K, Meijere AD. Liquid crystalline compounds with 2,2-disubstituted cyclopropane end groups in their side chains. Mol Cryst Liq Cryst. 2001;364(1):529–546. doi: 10.1080/10587250108025023
- Grebyonkin MF, Petrov VF, Belyaev VV, et al. Synthesis and properties of 5-alkyl-2-(4-cyanophenyl)pyridines. Mol Cryst Liq Cryst. 1985;129(1–3):245–257. doi: 10.1080/15421408408084179
- Pavlyuchenko AI, Smirnova NI, Mikhailova TA, et al. Synthesis and liquid-crystal properties of 2-(4-alkylphenyl or 4-alkoxyphenyl)-5-cyanopyridines. Zh Org Khim+. 1986;22:1061–1065. doi: 10.1002/chin.198637204
- Boller A, Cereghetti M, Schadt M, et al. Synthesis and some physical properties of phenylpyrimidines. Mol Cryst Liq Cryst. 1977;42(1):215–231. doi: 10.1080/15421407708084509
- Brettle R, Dunmur DA, Marson CM, et al. New liquid crystalline compounds based on thiophene. Chem Lett. 1992;21(4):613–616. doi: 10.1246/cl.1992.613
- Boardman FH, Dunmur DA, Grossel MC, et al. Synthesis and liquid crystal phase behaviour of 2-(4-cyanophenyl)-7-n-alkylfluorenes: luminescent mesogens. Chem Lett. 2002;31(1):60–61. doi: 10.1246/cl.2002.60
- Matsubara H, Seto K, Tahara T, et al. 2,5-diaryl-1,3,2-dioxaborinanes: a new series of liquid crystals. Bull Chem Soc J. 1989;62(12):3896–3901. doi: 10.1246/bcsj.62.3896
- Haramoto Y, Nobe A, Kamogawa H. Liquid crystal materials with sulfur atoms incorporated in the principal structure 1. New liquid crystal compounds with 1,3-dithiane ring. Bull Chem Soc J. 1984;57(7):1966–1969. doi: 10.1246/bcsj.57.1966
- Vorbrodt HM, Deresch S, Kresse H, et al. Kristallin-flüssige heterocycloalkane. I. Synthese und eigenschaften substituierter 1,3-dioxane. J Prakt Chem. 1981;323(323):902–913. doi: 10.1002/prac.19813230607
- Haramoto Y, Kamogawa H. 2-(p-cyanophenyl)-5-alkyl-1,3-oxathianes in liquid crystal mixture. Mol Cryst Liq Cryst. 1985;131(3–4):201–207. doi: 10.1080/00268948508085042
- Haramoto Y, Kamogawa H. New liquid crystalline compounds. 2-(p-cyanophenyl)-5-alkyl-1,3-oxathianes. Chem Lett. 1985;14:79–82. doi: 10.1246/cl.1985.79
- Haase W, Loub J, Paulus H, et al. The crystal and molecular structures of a second crystalline modification of the mesogenic trans-4-n-octyl-(4′-cyanophenyl)- cyclohexane (pch8b) and of two crystalline modifications of the mesogenic trans-4-n-nonyl-(4′-cyanophenyl)- cyclohexanes (pch9a, pch9b). Mol Cryst Liq Cryst. 1991;197:57–66.
- Gray GW, Kelly SM. The synthesis of 1,4-disubstituted bicyclo[2.2.2]octanes exhibiting wide-range, enantiotropic nematic phases. J Chem Soc Perkin Trans. 1981;2:26–31. doi: 10.1039/p29810000026
- Kozhushkov SI, Langer R, Yufit DS, et al. Novel liquid crystalline compounds containing bicyclo[3.1.0]hexane core units. Eur J Org Chem. 2004;2004(2):289–303. doi: 10.1002/ejoc.200300448
- De Meijere A, Messner M, Vill V. Liquid crystalline bicyclo[1.1.1]pentane derivatives. Mol Cryst Liq Cryst. 1994;257(1):161–167. doi: 10.1080/10587259408033773
- Wan D, Yang X, Li J, et al. Synthesis and properties of 5,6-dihydro-4h-cyclopenta[b]thiophene-based nematic liquid crystals: a new access to mesogens with high birefringence and large dielectric anisotropy. J Mol Liq. 2021;327:114827. doi: 10.1016/j.molliq.2020.114827
- Wan D, Che Z, Mo L, et al. Synthesis and properties of fluorinated terphenyl liquid crystals utilizing 5,6-dihydro-4h-cyclopenta[b]thiophene as core unit. J Mol Struct. 2022;1267:133612. doi: 10.1016/j.molstruc.2022.133612
- Wan D, Shi F, Che Z, et al. Introduction of 5,6-dihydro-4h-cyclopenta[b]thiophene core unit into phenyl-tolane: expanding the mesophase range and increasing the birefringence and dielectric anisotropy of liquid crystal. Liq Cryst. 2021;48(12):1650–1659. doi: 10.1080/02678292.2021.1897892
- Yang X, Li J, Li J, et al. High birefringence nematic liquid crystals containing both thieno[3,2-b]thiophene core and acetylene bond. Liq Cryst. 2022;49(6):845–854. doi: 10.1080/02678292.2022.2027530
- Yuan G, Hua R, Lv W, et al. Liquid crystal compound containing bicyclooctane as well as preparation method and application thereof. CN 103086843 A. 2013 Jan 31.
- Tian H, Jiang T, Hang D, et al. Bicyclo[2,2,1]heptane-containing compounds and application thereof. CN 103553872 A. 2013 Oct 31. 2014.
- Geivandov RC, Goncharova IV, Titov VV. Mesomorphic twistane derivatives: a new class of chiral nematogens. Mol Cryst Liq Cryst Incorporating Nonlinear Opt. 1989;166(1):101–103. doi: 10.1080/00268948908037141
- Kaszynski P, Friedli AC, Micul J. Mesogenic properties of telomers of [1. 1. 1]propellane. Mol Cryst Liq Cryst Lett. 1988;6:27–33.
- De Meijere A, Messner M, Kazhushkov S, et al. Liquid-crystalline compounds having bicyclo[1.1.1]pentane structure, liquid-crystal composition, and liquid-crystal display element. WO 1998/035924 A1. 1998 Feb 13.
- Kaszynski P, Friedli AC, McMurdie ND, et al. Synthesis of liquid crystals based on bicyclo[1.1.1]pentane. Mol Cryst Liq Cryst Incorporating Nonlinear Opt. 1990;191(1):193–197. doi: 10.1080/00268949008038593
- Chan LKM, Gemmell PA, Gray GW, et al. Synthesis and liquid crystal properties of compounds incorporating cyclobutane, spiro[3.3]heptane and dispiro[3.1.3.1]decane rings. Mol Cryst Liq Cryst. 1987;147(1):113–139. doi: 10.1080/00268948708084629
- Gray GW, McDonnell DG. Liquid crystal compounds incorporating the trans-1,4-substituted cyclohexane ring system. Mol Cryst Liq Cryst. 1979;53(1–2):147–166. doi: 10.1080/00268947908083992
- Carr N, Gray GW, Kelly SM. A comparison of the properties of some liquid crystal materials containing benzene, cyclohexane, and bicyclo[2.2.2]octane rings. Mol Cryst Liq Cryst. 1981;66(1):267–282. doi: 10.1080/00268948108072679
- Druon C, Wacrenier JM. Study of sa phase structure using dielectric relaxation. Mol Cryst Liq Cryst. 1983;98(1):201–208. doi: 10.1080/00268948308073475
- Bezborodov VS, Petrovich AE, Grinkevich OA, et al. Synthesis and mesomorphous properties of trans-2-alkyl-5-carboxy-1,3-dioxanes and their derivatives. Zh Org Khim+. 1985;21:440–442. doi: 10.1002/chin.198525247
- Bezborodov VS. Synthesis and mesomorphous properties of cis-2-alkyl-5-methyl-5-carboxy-1,3-dioxanes and trans-2-alkyl-5-methyl-5-carboxy-1,3-dioxanes and their derivatives. Zh Org Khim+. 1989;25:377–379. doi: 10.1002/chin.198925177
- Tschierske C, Vorbrodt HM, Kresse H, et al. Synthesis and mesomorphic properties of axially cyano-substituted 1,3-dioxane-5-carboxylates. Mol Cryst Liq Cryst Incorporating Nonlinear Opt. 1989;177(1):113–121. doi: 10.1080/00268948908047775
- Karamysheva LA, Geyvandova TA, Agafonova IF, et al. Liquid crystalline 1,3-disubstituted cyclopentanes. Mol Cryst Liq Cryst Incorporating Nonlinear Opt. 1990;191(1):237–246. doi: 10.1080/00268949008038600
- Campbell NL, Duffy WL, Thomas GI, et al. Nematic 2,5-disubstituted thiophenes. J Mater Chem. 2002;12:2706–2721. doi: 10.1039/b202073b
- Bezborodov VS, Konovalov VA, Lapanik VI, et al. Synthesis and mesomorphous properties of aryl esters of 4-alkyl-1-cyclohexene-1-carboxylic and 4-alkyl-3-cyclohexene-1-carboxylic acids. Zh Org Khim+. 1988;24:615–619. doi: 10.1002/chin.198829126
- Wang Q, Yan Fan S, Wong HNC, et al. Enantioselective synthesis of chiral liquid crystalline compounds from monoterpenes. Tetrahedron. 1993;49:619–638.
- Karamysheva LA, Geivandova TA, Roitman KV, et al. Liquid crystalline esters of alicyclic and heterocyclic acids. Mol Cryst Liq Cryst. 1983;99(1):169–175. doi: 10.1080/00268948308072039
- Sakurai Y, Takenaka S, Sugiura H, et al. Molecular structure and smectic properties: thermal properties of some liquid crystals having a nitro group at the terminal position. Mol Cryst Liq Cryst. 1991;201(1):95–113. doi: 10.1080/00268949108038639
- Abdulnabi NM, Abdul Razzaq Al-Obaidy MM, Tomi IHR, et al. New calamitic mesogens derived from a furan ring: synthesis, characterization and study of their mesomorphic behavior. J Mol Liq. 2021;325:114562. doi: 10.1016/j.molliq.2020.114562
- Miyake S, Kusabayashi S, Takenaka S. The thermal properties of liquid crystalline materials incorporating cyanothiophene and cyanofuran. Bull Chem Soc Jpn. 1984;57(9):2404–2407. doi: 10.1246/bcsj.57.2404
- Hird M, Goodby JW, Toyne KJ, et al. The synthesis and mesomorphic properties of some novel antiferroelectric liquid crystals. Mol Cryst Liq Cryst. 2001;365(1):213–220. doi: 10.1080/10587250108025297
- Mandle RJ, Goodby JW. Does topology dictate the incidence of the twist-bend phase? Insights gained from novel unsymmetrical bimesogens. Chem Eur J. 2016;22(51):18456–18464. doi: 10.1002/chem.201604030
- Tavadyan LA, Manukyan ZH, Harutyunyan LH, et al. Antioxidant properties of selenophene, thiophene and their aminocarbonitrile derivatives. Antioxidants. 2017;6(2):22. doi: 10.3390/antiox6020022
- Berriault CJ, Lightfoot NE. Occupational tellurium exposure and garlic odour. Occup Med. 2011;61(2):132–135. doi: 10.1093/occmed/kqq178
- Jansen A, Pauluth D, Klasen-Memmer M 2, 5-selenophene derivatives and 2, 5-tellurophene derivatives. US 8304036 B2. 2008 Aug 07. 2012.
- Friedman MR, Toyne KJ, Goodby JW, et al. The synthesis and transition temperatures of 2-(4-alkyl- and 4-alkoxy-phenyl)-5-cyano-1-benzofurans and related diaryl-1-benzofurans—an assessment of how deviations from linearity and conformational effects in a core unit affect mesogenicity. J Mater Chem. 2001;11(11):2759–2772. doi: 10.1039/b102837p
- Yano T Benzothiophene-containing liquid crystalline compound, liquid crystal composition and liquid crystal display device. EP 3305774 A1. 2016 May 10. 2018.
- Yano T Liquid-crystalline compound having benzothiophene, liquid-crystal composition, and liquid-crystal display element. WO 2017/064892 A1. 2016 Jul 11. 2017.
- Liang Z, Ma S, Yu J, et al. Double annulations of dihydroxy- and diacetoxy-dialkynylbenzenes. An efficient construction of benzodifurans. Tetrahedron. 2007;63(52):12877–12882. doi: 10.1016/j.tet.2007.10.051
- Horiguchi M, Kadomoto Y, Kusumoto T Polymerizable compound and optically anisotropic object. WO 2016/056542 A1. 2015 Oct 06. 2016.
- Iino H, Hanna J, Okamura H, et al. Organic compound, production method of the same, organic liquid crystal, organic semiconductor and the like. JP 2020083782 A. 2018 Nov 16. 2020.
- Seki A, Yoshio M. Multi-color photoluminescence based on mechanically and thermally induced liquid-crystalline phase transitions of a hydrogen-bonded benzodithiophene derivative. ChemPhysChem. 2020;21(4):328–334. doi: 10.1002/cphc.201901004
- Schmidt W, Vögtle F, Poetsch E. Spiro units as building blocks in thermotropic liquid crystals: synthesis and physical properties of terminally substituted spiro[5.5]undecanes. Liebigs Annalen. 1995;1995:1319–1326. doi: 10.1002/jlac.1995199507175
- Fecková M, Vachtlová M, Podlesný J, et al. Structural tailoring of p-terphenyl scaffold: towards advanced plastic scintillator. Dyes Pigm. 2023;219:111593. doi: 10.1016/j.dyepig.2023.111593
- Boettcher J, Hartmann R, Vögtle F. Langgestreckte moleküle von dispiro[5.1.5.2]-typ. Chem Ber. 1992;125(8):1865–1871. doi: 10.1002/cber.19921250813
- Feuerbacher N, Vögtle F, Windscheidt J, et al. Synthesis of rodlike dispiro hydrocarbon skeletons for new liquid crystal compounds. Synthesis. 1999;1999(1):117–120.
- Poetsch Eike DR, Schmidt Wolfgang DR, Voegtle Fritz Prof DR, et al. Spiroverbindungen. DE 19755245 B4. 1997 Dec 12. 2012.
- Miyazawa K, Demus D, Meijere AD. Optically active mesogenic dispiro[2.0.2.1]heptane derivatives and their unusual physical properties. Mol Cryst Liq Cryst. 2001;364(1):253–270. doi: 10.1080/10587250108024994
- Gray GW, Langley NA, Toyne KJ. Novel liquid crystalline derivatives of cubanes and hydrogenolyzed cubanes. Mol Cryst Liq Cryst. 1983;98(1):425–431. doi: 10.1080/00268948308073492
- Hopf H, Hussain Z, Menon RS, et al. Perhydroazulenes - a new class of liquid crystalline materials. Synlett. 2011;2011(9):1273–1276.
- Chan LKM, Gemmell PA, Gray GW, et al. Synthesis and liquid crystal properties of dimethylene linked compounds incorporating the cyclobutane or spiro[3.3]heptane rings. Mol Cryst Liq Cryst Incorporating Nonlinear Opt. 1989;168(1):229–245. doi: 10.1080/00268948908045974
- Gray G, Langley N, Toyne K. Novel cubane mesogens. Mol Cryst Liq Cryst. 1981;64(7–8):239–245. doi: 10.1080/01406568108080171
- Gyula B, Istvan J, Katalin F-C. Structure property relationship study on new cubane-1,4-dicarboxylic acid derivatives. XV Conference on Liquid Crystals; Zakopane, Poland: Proc.SPIE; 2004. pp. 6–12. https://www.spiedigitallibrary.org/conference-proceedings-of-spie/5565/1/Structure-property-relationship-study-on-new-cubane-14-dicarboxylic-acid/10.1117/12.581058.short
- Chen SH, Mastrangelo JC, Blanton TN, et al. Novel glass-forming liquid crystals. IV. Effects of central core and pendant group on vitrification and morphological stability. Liq Cryst. 1996;21(5):683–694. doi: 10.1080/02678299608032880
- Al-Janabi A, Mandle RJ. Utilising saturated hydrocarbon isosteres of para benzene in the design of twist-bend nematic liquid crystals. ChemPhysChem. 2020;21(8):697–701. doi: 10.1002/cphc.202000130
- Bényei G, Jalsovszky I, Demus D, et al. First liquid crystalline cuneane‐caged derivatives: a structure–property relationship study. Liq Cryst. 2006;33(6):689–696. doi: 10.1080/02678290600722940
- Yamamoto E, Kubo K, Mori A, et al. Synthesis, crystal structures and mesomorphic properties of liquid crystals with a n,n′-bis(tropon-2-yl)piperazine skeleton. Chem Lett. 2002;31(1):100–101. doi: 10.1246/cl.2002.100
- Roger B, Dunmur DA, Farrand LD, et al. 3-arylcyclohex-2-en-1-ones and 3,6-diarylcyclohex-2-en-1-ones. New liquid crystalline compounds. 1993;22:1663–1666. doi: 10.1246/cl.1993.1663
- Matsunaga Y, Miyamoto S. Mesomorphic behavior of 2,4-bis-(4-alkoxybenzylidene)cyclopentanones and related compounds. Mol Cryst Liq Cryst. 1993;237(1):311–317. doi: 10.1080/10587259308030145
- Eaton PE, Cassar L, Halpern J. Silver(i)- and palladium(ii)-catalyzed isomerizations of cubane. Synthesis and characterization of cuneane. J Am Chem Soc. 1970;92(21):6366–6368. doi: 10.1021/ja00724a061
- Smith E, Jones KD, O’Brien L, et al. Silver(i)-catalyzed synthesis of cuneanes from cubanes and their investigation as isosteres. J Am Chem Soc. 2023;145(30):16365–16373. doi: 10.1021/jacs.3c03207
- Son J-Y, Aikonen S, Morgan N, et al. Exploring cuneanes as potential benzene isosteres and energetic materials: Scope and mechanistic investigations into regioselective rearrangements from cubanes. J Am Chem Soc. 2023;145:16355–16364. doi: 10.1021/jacs.3c03226
- Kubo K, Sutoh T, Mori A, et al. Synthesis, structure, and mesomorphic properties of liquid crystals with a bitropone core. Bull Chem Soc J. 2002;75(6):1353–1358. doi: 10.1246/bcsj.75.1353
- Torralba MC, Cano M, Campo JA, et al. Chemistry of rh(i) complexes based on mesogenic 3,5-disubstituted pyrazole ligands. X-ray crystal structures of 3,5-di(4-n-butoxyphenyl)pyrazole (hpzbp2) and [rh(μ-pzr2)(co)2]2 (r=c6h4ocnh2n+1, n=10, 12) compounds. Part ii. J Organomet Chem. 2002;654:150–161. doi: 10.1016/S0022-328X(02)01400-6
- Bartulin J, Martinez R, Müller HJ, et al. Synthesis and mesomorphic properties of the 3,5-bis-alkoxyphenyl-pyrazoles and-isoxazoles. Mol Cryst Liq Cryst. 1992;220(1):67–75. doi: 10.1080/10587259208033430
- Dimitrowa K, Hauschild J, Zaschke H, et al. Kristallin-flüssige 1,3,4-thiadiazole. I. Biphenyl- und terphenylanaloge 1,3,4-thiadiazole. J Prakt Chem. 1980;3(22):933–944. doi: 10.1002/prac.19803220610
- Qu S, Chen X, Shao X, et al. Self-assembly of highly luminescent bi-1,3,4-oxadiazole derivatives through electron donor–acceptor interactions in three-dimensional crystals, two-dimensional layers and mesophases. J Mater Chem. 2008;18(33):3954–3964. doi: 10.1039/b804189j
- Dölling K, Zaschke H, Schubert H. Kristallin-flüssige thiazole. J Prakt Chem. 1979;321(4):643–654. doi: 10.1002/prac.19793210417
- Yadav AK, Pradhan B, Ulla H, et al. Tuning the self-assembly and photophysical properties of bi-1,3,4-thiadiazole derivatives through electron donor–acceptor interactions and their application in oleds. J Mater Chem C. 2017;5(36):9345–9358. doi: 10.1039/C7TC01420A
- Tomi IHR, Al-Daraji AHR, Al-Qaisi ZHJ, et al. Novel bent-core mesogenic of 4-amino-triazole derivatives: Synthesis, characterization and liquid crystalline study. Tetrahedron. 2016;72:4390–4399. doi: 10.1016/j.tet.2016.06.013
- Schubert H, Zaschke H. Synthese von kristallin-flüssigen verbindungen. III. N-alkyl- und n-alkoxyderivate des 2,5-diphenyl-pyrimidins. J Prakt Chem. 1970;312(3):494–506. doi: 10.1002/prac.19703120314
- Slater JW, Lydon DP, Rourke JP. Doubly cyclopalladated pyridazines: chiral liquid crystals. J Organomet Chem. 2002;645(1–2):246–255. doi: 10.1016/S0022-328X(01)01330-4
- Gushiken T, Ujiie S, Ubukata T, et al. Alkoxyphenyl-substituted symmetric liquid crystalline diamantane derivatives. Bull Chem Soc J. 2011;84(3):269–282. doi: 10.1246/bcsj.20100259
- Czajkowski M, Duda Ł, Czarnocki SJ, et al. Novel highly luminescent diketofurofuran dye in liquid crystal matrices for thermal sensors and light amplification. J Mater Chem C. 2023;11(13):4426–4438. doi: 10.1039/D2TC04376A
- Vill V, Tunger H-W. Liquid crystals derived from carbohydrates: synthesis and properties of oxadecaline compounds. Liebigs Annalen. 1995;1995:1055–1059. doi: 10.1002/jlac.1995199506149
- Kohne B, Praefcke K, Omar RS, et al. Calamitische flüssigkristalle: Beziehung zwischen molekülstruktur und mesogenität, trans-und cis-1,3,5,7-tetraoxadecalin-derivate [1,2]/calamitic liquid crystals: Relationship between molecular structure and mesogenity, trans- and cis-1,3,5,7 -tetraoxadecalin derivatives [1, 2]. Z Naturforsch B. 1986;41:736–750.
- Popova EL, Rozenberg VI, Starikova ZA, et al. Thermotropic liquid crystals from planar chiral compounds: optically active mesogenic [2.2]paracyclophane derivatives. 2002;41:3411–3414.
- Dewar MJS, Goldberg RS. Role of p-phenylene groups in nematic liquid crystals. J Am Chem Soc. 1970;92:1582–1586. doi: 10.1021/ja00709a026
- Schroeder JP. Liquid crystals: VII. Smectic-nematic transition temperature as a function of alkyl end group length in p-phenylene di-p-n-alkoxybenzoates. Mol Cryst Liq Cryst. 1980;61(3–4):229–240. doi: 10.1080/00268948008081343
- Them J, Vill V, Fischer F. Synthesis, properties and conformations of liquid crystalline 1,4-dioxanes and comparison with the corresponding 1,4-dithiane, cyclohexane, and benzene derivatives. Mol Cryst Liq Cryst Incorporating Nonlinear Opt. 1989;170:43–51. doi: 10.1080/00268948908047746
- Mori A, Taya H, Uchida M, et al. Mesogenic properties of tricyclic 2,5-di(4-alkoxybenzoyloxy)tropones. Increasing smectic thermal stabilities by the lateral polar carbonyl group. Chem Lett. 1996;25(8):699–700. doi: 10.1246/cl.1996.699
- Wang L, He W, Xiao X, et al. Wide blue phase range and electro-optical performances of liquid crystalline composites doped with thiophene-based mesogens. J Mater Chem. 2012;22(6):2383–2386. doi: 10.1039/C2JM15461G
- Shin S, Seo JW, Cho JK, et al. Bio-based chiral dopants having an isohexide skeleton for cholesteric liquid crystal materials. Green Chem. 2012;14(4):1163–1167. doi: 10.1039/c2gc16261j
- Chu-Tsin L. Molecular structure and phasef transition of thermotropic liquid crystals. Mol Cryst Liq Cryst. 1981;74(1):25–37. doi: 10.1080/00268948108073692
- Sevostyanov VP, Gnilomedova TI. Synthesis and study of liquid-crystalline benzeneazophenyl and para cyanobenzeneazophenyl esters of para alkoxybenzoic acids. Zh Org Khim+. 1976;12:2400–2403.
- Singh B, Pandey A. Liquid-crystalline properties of 2,5-bis(4-n-alkoxybenzoyloxy)-3,6-dichloro-1,4-benzoquinones and their copper (ii) complexes—synthesis and characterization. Mol Cryst Liq Cryst. 2010;524:3–16. doi: 10.1080/15421400903568104
- Mandle RJ, Davis EJ, Archbold CT, et al. Apolar bimesogens and the incidence of the twist–bend nematic phase. Chem Eur J. 2015;21:8158–8167. doi: 10.1002/chem.201500423
- Coles H, Pivnenko M, Hannington J Liquid crystal materials. EP 1713880 B1. 2005 Jan 26. 2009.
- Tanaka H. Liquid crystal compound having biphenylene, liquid crystal composition and liquid crystal display device. EP 3315583 A1. 2017 Sep 25. 2018.
- Arakawa Y, Kang S, Tsuji H, et al. Development of novel bistolane-based liquid crystalline molecules with an alkylsulfanyl group for highly birefringent materials. RSC Adv. 2016;6(20):16568–16574. doi: 10.1039/C5RA25122B
- Lee C-H, Yamamoto T. Synthesis of liquid crystals with bent-rod structure: mesogenic thiazole derivatives with long alkoxyl chains. Mol Cryst Liq Cryst. 2001;363(1):77–84. doi: 10.1080/10587250108025259
- Aguiar LDO, Junior ASL, Bechtold IH, et al. Molecular 5,8-π-extended quinoxaline derivatives as chromophores for photoluminescence applications. J Mol Liq. 2019;296:111763. doi: 10.1016/j.molliq.2019.111763
- Long TM, Swager TM. Triptycene-containing bis(phenylethynyl)benzene nematic liquid crystals. J Mater Chem. 2002;12(12):3407–3412. doi: 10.1039/B203160D
- Arakawa Y, Kang S, Nakajima S, et al. Diphenyltriacetylenes: novel nematic liquid crystal materials and analysis of their nematic phase-transition and birefringence behaviours. J Mater Chem C. 2013;1(48):8094–8102. doi: 10.1039/c3tc31658k
- Aliev DF, Bayramov GM, Mirbaghirova GM. On the physicochemical properties of new liquid-crystalline compositions exhibiting the smectic a phase. Mol Cryst Liq Cryst Incorporating Nonlinear Opt. 1987;151(1):335–344.
- Buey J, Espinet P. Monomeric and dimeric ortho-metallated palladium(ii)-imine systems as liquid crystals. J Organomet Chem. 1996;507(1–2):137–145. doi: 10.1016/0022-328X(95)05771-G
- Ruslim C, Ichimura K. Z-isomers of 3,3′-disubstituted azobenzenes highly compatible with liquid crystals. J Mater Chem. 1999;9(3):673–681. doi: 10.1039/a808083f
- Daugvila Y, Tubyalite A, Denis G, et al. Liquid-crystals of alpha-cyanostilbene type. Zh Obshch Khim+. 1976;46:2125–2130. doi: 10.1002/chin.197701228
- Tanguy L, Leclercq M, Billard J, et al. Studies on mesomorphic compounds .4. Derivatives of trans-stilbene and trans hexahydrochrysene. BULLETIN DE La SOCIETE CHIMIQUE DE FRANCE PARTIE II-CHIMIE MOLECULAIRE ORGANIQUE ET BIOLOGIQUE. 1974;(3-4):640–640.
- Brettle R, Dunmur DA, Hindley NJ, et al. Synthesis of new liquid crystalline compounds based on 1,4-diarylbuta-1,3-dienes. J Chem Soc Perkin Trans. 1993;1:775–781. doi: 10.1039/p19930000775
- Chudgar NK, Shah SN, Vora RA. Mesogenic properties as an analytical tool for the pyrolytic transformation of substituted aryl aldehyde semicarbazones to corresponding benzalazines. Mol Cryst Liq Cryst. 1991;209(1):237–241. doi: 10.1080/00268949108036198
- Marcos M, Melendez E, Serrano JL. Synthesis and mesomorphic properties of three homologous series of 4,4′-dialkoxy-α,α′-dimethylbenzalazines a comparative study (i. Mol Cryst Liq Cryst. 1983;91(1–2):157–172. doi: 10.1080/00268948308083083
- Zhao K-Q, Hu P, Xu H-B. Synthesis and structure of 1,4-diazabutadiene liquid crystals. Mol Cryst Liq Cryst. 2001;364(1):801–808. doi: 10.1080/10587250108025051
- Ohta K, Muroki H, Hatada K-I, et al. First examples exhibiting both double melting behavior and mesomorphism in a series of long chain substituted compounds: 1,3-di(p-n-alkoxyphenyl)propane-1,3-dione. Mol Cryst Liq Cryst. 1986;140:163–177. doi: 10.1080/00268948608080150
- Weissflog W, Schubert H. Kristallin-flüssige aldoximester. J Obstet Gynaecol. 1976;318:785–794. doi: 10.1002/prac.19763180510
- Weissflog W, Schubert H, König S, et al. Kristallin-flüssige ketoximester. J Prakt Chem. 1977;319(3):507–515. doi: 10.1002/prac.19773190321
- Torgova SI, Karamysheva LA, Agafonova IF. Phenacyl esters as the new class of liquid-crystal compounds. Zh Org Khim+. 1992;28:1472–1478.
- Schubert H, Hauschild J, Demus D, et al. Diacylhydrazin-mesogene;) symmetrische 1,2-dibenzoylhydrazinderivate. Z Chem. 1978;18:256–256. doi: 10.1002/zfch.19780180706
- Iliş M, Bucos M, Dumitraşcu F, et al. Mesomorphic behaviour of n-benzoyl-n′-aryl thioureas liquid crystalline compounds. J Mol Struct. 2011;987(1–3):1–6. doi: 10.1016/j.molstruc.2010.11.037
- Hope-Ross KA, Heiney PA, Kadla JF. A new family of bent-core c2-symmetric liquid crystals. Can J Chem. 2010;88(7):639–645. doi: 10.1139/V10-056
- Chen Y-W, Lin Y-C, Kuo H-M, et al. Fluorescent columnar bis(boron difluoride) complexes derived from tetraketonates. J Mater Chem C. 2017;5(22):5465–5477. doi: 10.1039/C7TC01425B
- Hoffmann S, Hanh NT, Mandl K, et al. Vinamid-mesogene – [4-n-alkoxy-phenyl]-[β-(4-n-alkoxy-phenyl)amino-vinyl]-ketone. Z Chem. 1986;26:103–104. doi: 10.1002/zfch.19860260312
- Rampon DS, Rodembusch FS, Schneider JMFM, et al. Novel selenoesters fluorescent liquid crystalline exhibiting a rich phase polymorphism. J Mater Chem. 2010;20:715–722. doi: 10.1039/B917366H
- Kirsch P, Bremer M. Hypervalent sulfur fluorides and the design of liquid crystals. Chimia. 2014;68(6):363. doi: 10.2533/chimia.2014.363
- Hartley CS, Kapernaum N, Roberts JC, et al. Electroclinic effect in chiral sma* liquid crystals induced by atropisomeric biphenyl dopants: amplification of the electroclinic coefficient using achiral additives. J Mater Chem. 2006;16(24):2329–2337. doi: 10.1039/b515313a
- Thurmes WN, More KM, Meadows MR, et al. Germanium liquid crystals. Liq Cryst. 2009;36(5):461–477. doi: 10.1080/02678290903006223
- Li L, Jones CD, Magolan J, et al. Siloxane-terminated phenylpyrimidine liquid crystal hosts. J Mater Chem. 2007;17(22):2313–2318. doi: 10.1039/b700972k
- Fuenfschilling J, Villiger A Cyclopentyl derivatives. US 5759443 A. 1997 June 26. 1998.
- Mandle RJ, Abbott LC, Fritsch L, et al. Engineering mesophase stability and structure via incorporation of cyclic terminal groups. J Mater Chem C. 2022;10(15):5934–5943. doi: 10.1039/D1TC05954H
- Cook A, Badriya S, Greenfield S, et al. Styrene-containing mesogens. Part 1: photopolymerisable nematic liquid crystals. J Mater Chem. 2002;12(9):2675–2683. doi: 10.1039/b203940k
- Griffin AC, Vaidya SR. Effect of molecular structure on mesomorphism 22.1 liquid crystalline1-alkanol derivatives. Mol Cryst Liq Cryst Incorporating Nonlinear Opt. 1989;173(1):85–88. doi: 10.1080/00268948908033369
- Mandle RJ, Davis EJ, Voll C-CA, et al. Self-organisation through size-exclusion in soft materials. J Mater Chem C. 2015;3:2380–2388. doi: 10.1039/C4TC02991G
- Kostromin SG, Cuong ND, Garina ES, et al. Liquid crystalline comb-shaped poly (vinyl ether)s with cyanbiphenyl mesogenic groups. Mol Cryst Liq Cryst Incorporating Nonlinear Opt. 1990;193(1):177–184. doi: 10.1080/00268949008031823
- Davis EJ, Mandle RJ, Russell BK, et al. Liquid-crystalline structure–property relationships in halogen-terminated derivatives of cyanobiphenyl. Liq Cryst. 2014;41(11):1635–1646. doi: 10.1080/02678292.2014.940505
- Gibb CJ, Storey JMD, Imrie CT. A convenient one-pot synthesis, and characterisation of the ω-bromo-1-(4-cyanobiphenyl-4’-yl) alkanes (cbnbr). Liq Cryst. 2022;49(12):1706–1716. doi: 10.1080/02678292.2022.2084568
- Wang D, Liu J, Zhao W, et al. Facile synthesis of liquid crystal dimers bridged with a phosphonic group. Chem Eur J. 2022;28(70):e202202146. doi: 10.1002/chem.202202146
- Mehl GH, Goodby JW. Substituted siloxysilanes and the structure of oligomeric liquid crystals. Chem Commun. 1999;1999(1):13–14. doi: 10.1039/a807549b
- Pensec S, Tournilhac F-G. An ω-functionalized perfluoroalkyl chain: synthesis and use in liquid crystal design. Chem Commun. 1997;1997(5):441–442. doi: 10.1039/a700085e
- Terazzi E, Rogez G, Gallani J-L, et al. Supramolecular organization and magnetic properties of mesogen-hybridized mixed-valent manganese single molecule magnets [mniii8mniv4o12(lx,y,z-cb)16(h2o)4]. J Am Chem Soc. 2013;135(7):2708–2722. doi: 10.1021/ja311190a
- Kim D-J, Oh N-K, Lee M, et al. Liquid crystalline assembly of calamatic mesogens and rod-coil molecule by pd and ru complexation. Mol Cryst Liq Cryst. 1996;280(1):129–134. doi: 10.1080/10587259608040321
- Hird M. Fluorinated liquid crystals – properties and applications. Chem Soc Rev. 2007;36(12):2070–2095. doi: 10.1039/b610738a
- Guittard F, Taffin de Givenchy E, Geribaldi S, et al. Highly fluorinated thermotropic liquid crystals: an update. J Fluorine Chem. 1999;100(1–2):85–96.
- Sasnouski G, Bezborodov V, Dabrowski R, et al. Cyclopropanoles as the versatile intermediates in the synthesis of polyring liquid crystalline compounds. Mol Cryst Liq Cryst. 2001;365(1):63–74. doi: 10.1080/10587250108025282
- Yamada S, Hashishita S, Asai T, et al. Design, synthesis and evaluation of new fluorinated liquid crystals bearing a cf2cf2 fragment with negative dielectric anisotropy. Org Biomol Chem. 2017;15(6):1495–1509. doi: 10.1039/C6OB02431A
- Hird M, Toyne KJ, Slaney AJ, et al. The synthesis and transition temperatures of some difluoro-substituted cyclohexanes. J Chem Soc Perkin Trans. 1993;2:2337–2349. doi: 10.1039/p29930002337
- Bremer M, Pauluth D, Krause J, et al. Fluorierte cyclohexan-derivate und flüssigkristallines medium. DE 19918009 B4. 1999 Apr 21. 2010.
- Kirsch P, Bremer M, Kirsch A, et al. Materials for liquid crystal displays with reduced power consumption. Mol Cryst Liq Cryst. 2000;346(1):193–199. doi: 10.1080/10587250008023878
- Kamata T, Suzuki T Difluorobicyclo[2.2.2]octane compound, method for producing the same, and use thereof, and method for producing hydroxybicyclooctanone compound. JP 2010202542 A. 2009 Mar 02. 2010.
- Sidrauski C, Pliushchev M, Frost Jennifer M, et al. Modulators of the integrated stress pathway. WO 2017/193034 A1. 2017 May 05.
- Kirsch P, Tarumi K. A novel type of liquid crystals based on axially fluorinated cyclohexane units. Angew Chem Int Ed. 1998;37(4):484–489.
- Al-Maharik N, Kirsch P, Slawin AMZ, et al. Fluorinated liquid crystals: evaluation of selectively fluorinated facially polarised cyclohexyl motifs for liquid crystal applications. Org Biomol Chem. 2016;14:9974–9980. doi: 10.1039/C6OB01986B
- Bremer M, Lietzau L. 1,1,6,7-tetrafluoroindanes: Improved liquid crystals for lcd-tv application. New J Chem. 2005;29:72–74. doi: 10.1039/b414312d
- Demus D, Goto Y, Sawada S, et al. Trifluorinated liquid crystals for tft displays. Mol Cryst Liq Cryst. 1995;260(1):1–21. doi: 10.1080/10587259508038680
- Yamamoto H, Takeshita F, Terashima K, et al. Liquid crystalline compounds having 1,2,3-trifluorophenyl substituent for am-lcds with low-voltage ic driver. Mol Cryst Liq Cryst. 1995;264(1):57–65. doi: 10.1080/10587259508037302
- Andou T, Shibata K, Matsui S Polyhaloalkyl ether derivatives as well as liquid crystal compositions and liquid crystal display elements containing them. CN 1188134 A. 1997 Nov 22. 1998.
- Chen X, Wu C, Liu G. Preparation method of cyclohexyl methoxyl bridge type positive dielectric liquid crystal compound. CN 108658813 A. 2018 June 05.
- Kirsch P, Huber F, Lenges M, et al. Liquid crystals with multiple fluorinated bridges in the mesogenic core structure. J Fluorine Chem. 2001;112(1):69–72.
- Kozel V, Daniliuc C-G, Kirsch P, et al. C3-symmetric tricyclo[2.2.1.02,6]heptane-3,5,7-triol. Angew Chem Int Ed. 2017;5(6):15456–15460. doi: 10.1002/anie.201709279
- Solladie G, Zimmermann R. A new class of chiral smectic liquid crystals: substituted biphenylylcyclohexylidene ethanols having an axial chirality. J Org Chem. 1985;50:4062–4068. doi: 10.1021/jo00221a020
- Lunkwitz R, Zab K, Tschierske C. Axial chiral liquid crystals–synthesis of trisubstituted allenyl ethers. Journal für Praktische Chemie/Chemiker-Zeitung. 1998;340:662–668. doi: 10.1002/prac.19983400711
- Lemieux RP. Chirality transfer in ferroelectric liquid crystals. Acc Chem Res. 2001;34:845–853. doi: 10.1021/ar9901164
- Ayub K, Moran M, Lazar C, et al. Liquid crystals with axially chiral 3,3′-dinitro-2,2′,6,6′-tetramethylbiphenyl cores: the lateral shielding effect of bicyclo[2.2.2]octane-1-carboxylate terminal chains. J Mater Chem. 2010;20:6655–6661. doi: 10.1039/c0jm01005g
- Solladié G, Hugelé P, Bartsch R, et al. New family of enantiomerically pure liquid crystals containing a chiral biphenyl core. Angew Chem Int Ed Engl. 1996;35(13–14):1533–1535. doi: 10.1002/anie.199615331
- Williams VE, Lemieux RP. Substituent effects in the induction of a cholesteric liquid crystal phase by atropisomeric dibenzoxepins: a study of arene–arene interactions. Chem Commun. 1996;1996(19):2259–2260. doi: 10.1039/CC9960002259
- di Matteo A, Todd SM, Gottarelli G, et al. Correlation between molecular structure and helicity of induced chiral nematics in terms of short-range and electrostatic−induction interactions. The case of chiral biphenyls. J Am Chem Soc. 2001;123(32):7842–7851. doi: 10.1021/ja010406r
- Thompson MP, Lemieux RP. Chiral induction in nematic and smectic c liquid crystal phases by dopants with axially chiral 1,11-dimethyl-5,7-dihydrodibenz[c,e]thiepin cores. J Mater Chem. 2007;17(48):5068–5076. doi: 10.1039/b712253e
- Finden JG, Yuh E, Huntley C, et al. Ferroelectric liquid crystals induced by dopants with axially chiral 2,2′‐spirobiindan‐1,1′‐dione cores: diether vs. Diester side‐chains. Liq Cryst. 2007;34(9):1095–1106. doi: 10.1080/02678290701528899
- Boulton CJ, Finden JG, Yuh E, et al. Ferroelectric liquid crystals induced by dopants with axially chiral 2,2‘-spirobiindan-1,1‘-dione cores. J Am Chem Soc. 2005;127(39):13656–13665. doi: 10.1021/ja054322k
- Brettar J, Bürgi T, Donnio B, et al. Ferrocene-containing optically active liquid-crystalline side-chain polysiloxanes with planar chirality. Adv Funct Mater. 2006;16(2):260–267. doi: 10.1002/adfm.200500223
- Mathews M, Tamaoki N. Planar chiral azobenzenophanes as chiroptic switches for photon mode reversible reflection color control in induced chiral nematic liquid crystals. J Am Chem Soc. 2008;130(34):11409–11416. doi: 10.1021/ja802472t
- Mandle RJ, Goodby JW. An experimental and computational study of calamitic and bimesogenic liquid crystals incorporating an optically active [2,2]-paracyclophane. Liq Cryst. 2018;45(11):1567–1573. doi: 10.1080/02678292.2018.1453559
- Nuckolls C, Shao R, Jang W-G, et al. Electro-optic switching by helicene liquid crystals. Chem Mater. 2002;14(2):773–776. doi: 10.1021/cm010628o
- Nuckolls C, Katz TJ. Synthesis, structure, and properties of a helical columnar liquid crystal. J Am Chem Soc. 1998;120(37):9541–9544. doi: 10.1021/ja982025s
- Nuckolls C, Katz TJ, Castellanos L. Aggregation of conjugated helical molecules. J Am Chem Soc. 1996;118(15):3767–3768. doi: 10.1021/ja960145z
- Gottarelli G, Hansen H-J, Spada GP, et al. A liquid-crystal study of heptalene. Helv Chim Acta. 1987;70(2):430–435. doi: 10.1002/hlca.19870700222
- Arakawa Y, Sasaki Y, Igawa K, et al. Hydrogen bonding liquid crystalline benzoic acids with alkylthio groups: phase transition behavior and insights into the cybotactic nematic phase. New J Chem. 2017;41(14):6514–6522. doi: 10.1039/C7NJ00282C
- Fouzai M, Hamdi R, Ghrab S, et al. Properties of binary mixtures derived from hydrogen bonded liquid crystals. J Mol Liq. 2018;249:1279–1286. doi: 10.1016/j.molliq.2017.11.128
- Takenaka S. Thermal properties of novel liquid crystals having a single benzene unit. J Chem Soc Chem Commun. 1992;1992(23):1748–1749. doi: 10.1039/c39920001748
- Bao X, Dix LR. Smectic phases observed in 1, 1,2,2,-tetrahydroperfluoroalkyl-4-nitrobenzoates. Mol Cryst Liq Cryst. 1996;281(1):291–294. doi: 10.1080/10587259608042252
- Johansson G, Percec V, Ungar G, et al. Fluorophobic effect generates a systematic approach to the synthesis of the simplest class of rodlike liquid crystals containing a single benzene unit. Chem Mater. 1997;9(1):164–175. doi: 10.1021/cm960267q
- Sakurai Y, Takenaka S, Miyake H, et al. Molecular structure and smectic properties. Part 1. The effect of linkages on smectic a thermal stability in three aromatic ring compounds linked by ester groups. J Chem Soc Perkin Trans. 1989;2:1199–1204. doi: 10.1039/p29890001199
- Mori A, Taya H, Takeshita H, et al. Preparation of troponoid liquid crystals with a flexible oxymethylene linking unit. J Mater Chem. 1998;8(3):595–597. doi: 10.1039/a704536k
- Mori A, Takeshita H, Kida K, et al. Synthesis of 2-(alkanoyloxy)-5-alkoxytropones. Novel monocyclic rod-type liquid crystals. J Am Chem Soc. 1990;112(23):8635–8636. doi: 10.1021/ja00179a086
- Mori A, Uno K, Takeshita H, et al. Mesomorphic properties of monocyclic troponoids with a semi‐fluorinated side group. Liq Cryst. 2005;32(1):107–113. doi: 10.1080/02678290512331324020
- Mori A, Takeshita H, Mori R, et al. Monocyclic rod-type liquid crystals; 2,5-dialkanoyloxytropones and 5-alkanoyloxy-2-alkoxytropones. Liq Cryst. 2001;28(2):171–178. doi: 10.1080/026782901462616
- Choffat F, Käser S, Wolfer P, et al. Synthesis and characterization of linear poly(dialkylstannane)s. Macromolecules. 2007;40(22):7878–7889. doi: 10.1021/ma071463t
- Eaborn C, Hartshorne NH. The mesomorphism of diisobutylsilanediol. J Chem Soc (Resumed). 1955;1955:549–555. doi:10.1039/jr9550000549
- Shimizu M, Nata M, Mochida K, et al. 1-alkyl 2,3,5,6,7,8-hexasilabicyclo[2.2.2]octanes: unconventional class of mesomorphic columnar compounds. Angew Chem Int Ed. 2007;46(17):3055–3058. doi: 10.1002/anie.200604170
- Tschierske C. Non-conventional liquid crystals—the importance of micro-segregation for self-organisation. J Mater Chem. 1998;8(7):1485–1508. doi: 10.1039/a800946e
- Mahler W, Guillon D, Skoulios A. Smectic liquid crystal from (perfluorodecyl)decane. Mol Cryst Liq Cryst Lett. 1985;2:111–119.
- Viney C, Russell TP, Depero LE, et al. Transitions to liquid crystalline phases in a semifluorinated alkane. Mol Cryst Liq Cryst Incorporating Nonlinear Opt. 1989;168(1):63–82. doi: 10.1080/00268948908045960
- Rabolt JF, Russell TP, Twieg RJ. Structural studies of semifluorinated n-alkanes. 1. Synthesis and characterization of f(cf2)n(ch2)mh in the solid state. Macromolecules. 1984;17(12):2786–2794. doi: 10.1021/ma00142a060
- Ternansky RJ, Balogh DW, Paquette LAD. Dodecahedrane. J Am Chem Soc. 1982;104(16):4503–4504. doi: 10.1021/ja00380a040
- Ivanov DM, Baykov SV, Novikov AS, et al. Noncovalent sulfoxide–nitrile coupling involving four-center heteroleptic dipole–dipole interactions between the sulfinyl and nitrile groups. Cryst Growth Des. 2020;20(5):3417–3428. doi: 10.1021/acs.cgd.0c00196