ABSTRACT
We report the synthesis and phase behaviour of a series of liquid crystalline trimers containing secondary amide groups. These materials form nematic, smectic and twist-bend nematic phases and their liquid crystal behaviour appears to be primarily driven by their molecular shape, with little evidence for the influence of hydrogen bonding between the amide groups. The results show that suitable molecular design can allow for amides to be included into mesogenic materials in an analogous manner to more commonly used linking groups, such as esters and imines, offering new synthetic routes to liquid crystalline materials.
1. Introduction
Liquid crystals (LCs) are fluid phases which retain some degree of order between molecules, and as such may be considered as an intermediate between ordered crystalline solids and conventional isotropic liquids. The anisotropic properties of liquid crystals have been harnessed for a plethora of applications, from LCD displays to stimuli-responsive polymers. The field of liquid crystals is a vibrant area of research covering a vast range of materials which may be categorised based on the type of phases they form, or by the nature of the material itself. Thermotropic LCs, which change their state in response to a change in temperature, can be classified based on the degree of molecular order [Citation1].
The simplest LC phase is the uniaxial nematic phase (), in which molecules display short-range orientational order but have no long-range positional order. As such, molecules in the nematic phase may be thought of as having their long axes pointing, on average, in the same direction, which is known as the director, n. Smectic phases have a greater degree of order than nematic phases and are characterised by their lamellar structure (). There are a variety of different smectic phases, and they differ based on the degree of ordering present within the layers. Alternately, liquid crystals can be divided into polymeric or low molar mass (LMM) liquid crystals. Oligomeric liquid crystals, such as dimers (consisting of two rigid mesogenic units linked by a flexible spacer) and trimers (three mesogenic units with two flexible spacers) were first studied as model systems to understand the behaviour of liquid crystalline polymers. However, they have recently attracted a great deal of interest in their own right, following the observation of spontaneous chiral symmetry breaking in achiral dimers with an overall bent shape [Citation2,Citation3]. Known as the twist-bend nematic (NTB) phase, frustration induced by the curved molecular shape causes the director to spontaneously bend and twist, forming degenerate left- and right-handed helices (). One of the intriguing features of the NTB phase is the very short helical pitch length, often less than 10 nm, regardless of the size of the molecules that form the phase [Citation4–6]. While the NTB phase has been most commonly studied in dimers, it has been shown that the NTB phase exhibited by trimers has a number of significant differences, including a much less temperature-dependent pitch length, and this may be important in potential applications [Citation7]. The behaviour of such mesogens was attributed largely to the shape of the trimers and how they pack. This introduces a key question in the development of liquid crystal structure–property relationships: to what degree is phase formation driven by the overall molecular shape, compared to the influence of individual chemical functional groups present? In this work, we aim to examine this question by preparing a series of liquid crystal trimers containing secondary amide groups and investigate their mesomorphic properties.
Figure 1. (Colour online) (a) A sketch of the molecular arrangement in the a) nematic phase, showing the director, n; (b) lamellar smectic A phase; (c) twist-bend nematic phase showing the formation of degenerate helices.
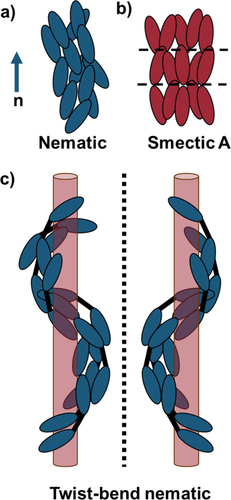
The amide group, while used extensively in polymeric systems, has been used sparingly in the design of low molar mass calamitic (rod-shaped) liquid crystals. The presence of hydrogen bonding between amide groups usually leads to very high melting points, but early work showed that materials containing both ester and amide linking groups, with long alkyl chains or lateral substituents did show mesogenic behaviour [Citation8–10]. The hydrogen bonding between amide groups has been exploited to promote the formation of layered phases in calamitic liquid crystals [Citation11]. More recently, we have shown that bent liquid crystal dimers containing a secondary benzanilide group show the twist-bend nematic phase [Citation12,Citation13].
The structures of the trimers discussed in this work are given in . The key feature in these is the central benzanilide-based mesogenic unit. We have changed the terminal mesogenic groups from cyanobiphenyl to cyanoterphenyl in order to promote liquid crystalline behaviour and increased the length of the flexible spacers from 6 to 10 carbon atoms to enhance molecular flexibility. We stress that the spacers are all odd-membered in order to give rise to a bent molecular shape, widely believed to be a prerequisite for the observation of the NTB phase [Citation14,Citation15]. We have investigated the effect of changing the number of lateral methyl substituents, and also their positions around the central amide group.
2. Experimental
The syntheses of the trimers reported here were carried out according to literature methods, and full experimental details and structural characterisation data are given in the ESI.
The phase behaviour of the materials was studied using differential scanning calorimetry (DSC) with a Mettler Toledo DSC3 differential scanning calorimeter equipped with a TSO 801RO sample robot and calibrated with indium and zinc standards. The heating and cooling rates were 10 K min−1 and the transition temperatures and their associated enthalpy changes were extracted from heating traces unless otherwise noted. Optical textures were used to identify the liquid crystal phases and these were observed using an Olympus BH2 polarising optical microscope equipped with a Linkam TMS 92 heating stage.
Geometry optimisation was carried out using DFT calculations at the B3LYP 6-31 G(d) level of theory with Gaussian09 software [Citation16]. Visualisations of the electrostatic potential surface were generated from the optimised geometries using the Gaussview package. Space-filling models were generated from the optimised geometries using the QuteMol package [Citation17]. Optimisations were carried out with the alkyl chains in the all-trans conformation, and this is in line with previous studies [Citation14].
Optical birefringence was measured with a setup based on a photoelastic modulator (PEM-90, Hinds) working at a modulation frequency f = 50 kHz; a halogen lamp (Hamamatsu LC8) equipped with narrow bandpass filters was used as a light source. The signal from a photodiode (FLC Electronics PIN-20) was deconvoluted with a lock-in amplifier (EG&G 7265) into 1 f and 2 f components to yield a retardation induced by the sample. Knowing the sample thickness, the retardation was recalculated into optical birefringence. Samples were prepared in 3-micron-thick cells with planar anchoring. The alignment quality was checked prior to measurement by inspection under the polarised optical microscope.
AFM measurements were performed using a Bruker Dimension Icon Microscope working in tapping or scan assist mode and cantilevers with elastic constant of 0.4 N/m were applied. Image processing was carried out using SciAnalysis Copyright © 2022, Brookhaven Science Associates, LLC, Brookhaven National Laboratory [Citation18].
X-ray diffraction measurements were carried out using a Bruker D8 GADDS system with CuKα radiation, Goebel mirror monochromator, point beam collimator, and VANTEC2000 area detector. SAXS measurements were performed on a Bruker Nanostar system using CuKα radiation and patterns were collected with an area detector VANTEC2000.
Variable Temperature Infrared (VTIR) spectra were obtained using a Perkin Elmer spectrum 100 FTIR spectrometer equipped with a Linkam TMS 92 heating stage. Samples were prepared between KBr discs.
3. Results
The transition temperatures and the associated enthalpy and entropy changes for the trimers are given in . All seven compounds form the nematic phase, which could be identified based on the characteristic textures observed with a polarised light optical microscope. The nematic phases were enantiotropic in nature except for that shown by the cyanobiphenyl-based trimer 1. Additional smectic or twist-bend nematic phases were seen for all trimers except 6, which had two lateral methyl substituents at the ortho position to the amide nitrogen.
Table 1. Transition temperatures and the associated enthalpy and entropy changes for the trimers shown in . Monotropic transition temperatures are shown in ().
Trimer 1 consists of two cyanobiphenyl groups linked by hexyloxy spacers to the central methyl-substituted benzanilide fragment. This melts directly into the isotropic phase, and forms monotropic nematic and twist-bend nematic phases. On cooling the isotropic phase, a characteristic schlieren texture is formed containing both two- and four-point brush defects and which flashed when subject to mechanical stress (). The NTB phase showed a characteristic blocky texture on untreated glass, and a striped texture is seen in thin cells treated for planar alignment ( inset and Figure S.1). The optical birefringence increases on cooling in the N phase following the growing orientational order of the molecules, but starts to decrease at the transition from the nematic to NTB phase, which is consistent with the tilting of the molecules and the formation of heliconical structure () [Citation19].
Figure 3. (Colour online) The change in the optical birefringence of trimer 1 on cooling. (Inset left): the striped POM texture of the NTB phase shown by 1 in a cell treated for planar alignment at 98°C. (Inset right): the schlieren texture seen for 1 in the nematic phase at 127°C on untreated glass.
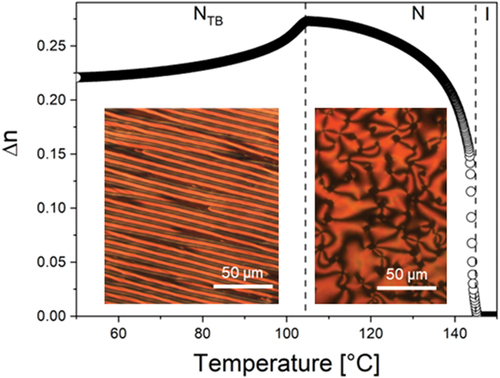
3.1. Increasing spacer length
As liquid crystalline behaviour for trimer 1 was only seen on cooling, the cyanobiphenyl outer groups were replaced with cyanoterphenyl units to increase the transition temperatures and promote the formation of enantiotropic LC phases. The cyanoterphenyl-based trimer with the shortest (hexyloxy) spacers, 2, forms an enantiotropic nematic phase and a monotropic smectic phase. The smectic phase gives a smooth fan-like texture on untreated glass and on shearing the sample a schlieren texture is seen containing two- and four-point brush defects (), which strongly suggests a tilted phase with anticlinic arrangement of mesogenic cores in neighbouring layers – an anticlinic smectic C (SmCA) phase (anticlinic refers here to tilting of the mesogenic cores, not the molecular axis, from the layer normal, see ). The X-ray diffraction pattern of the smectic phase contains sharp commensurate signals in the small-angle region arising from the layered structure, and a diffuse signal in the wide-angle region, indicating a liquid-like molecular order within the layers, consistent with the assignment of an SmCA phase (). Trimer 3 has octyloxy spacers, and forms an enantiotropic nematic phase, and two additional liquid crystal phases. The higher temperature phase shows a truncated fan-like texture on untreated glass which produces an optically extinct texture when sheared () Based on these observations, this phase is assigned as a smectic A phase, as the phase is optically uniaxial with the mesogenic cores aligned on average along the layer normal. On cooling from the SmA phase, on untreated glass, the fan texture develops a banded appearance, and from the homeotropic regions a schlieren texture forms, which features both two- and four-point brush defects (). These textures are consistent with the assignment of this phase as an anticlinic smectic C phase, as was seen for compound 2. Trimer 4 contains the longest (decyloxy) spacers and forms enantiotropic N phase and twist-bend nematic phases. The NTB phase shows rope-like POM textures on untreated glass (), and the X-ray diffraction pattern confirmed the phase assignment, as only diffuse signals are seen in both the wide and small angle regions ()
Figure 4. (Colour online) (a) POM texture of trimer 2 in the SmCA phase at 155°C on untreated glass with (inset) the schlieren texture seen upon shearing of the sample; (b) the X-ray diffraction pattern seen for 2 at 155°C; (c) truncated fan-like texture of the SmA phase of trimer 3 on untreated glass at 171°C and (inset) optically extinct texture on untreated glass; (d) the banded fan-like texture of the SmCA phase for trimer 3 on untreated glass at 136°C with (inset) the schlieren texture seen upon shearing; (e) the rope-like texture of the NTB phase of trimer 4 at 125°C on untreated glass; (f) the X-ray diffraction pattern of trimer 4 in the NTB phase at 120°C.
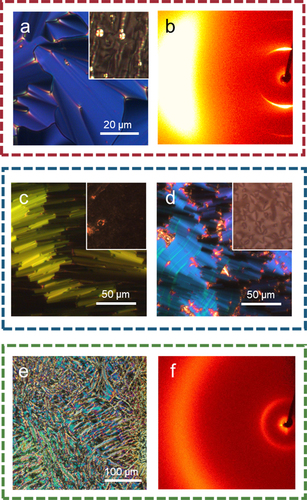
The birefringence of trimer 2 shows an increase at the isotropic to nematic transition, as well as a slight increase at the nematic to SmCA transition (), which may be attributed to the coupling between orientational and positional order. In contrast, the optical birefringence shown by trimer 4 increases at the isotropic–nematic transition, and then decreases at the transition to the NTB phase (), which resembles the behaviour seen for trimer 1.
3.2. The packing arrangement
The position of the small-angle XRD signal for the SmCA phase of trimer 2 () corresponds to a layer spacing (d) of 17 Å. DFT geometry optimisation gives a molecular length (l) of 53 Å for 2, which suggests a triply intercalated structure. The layer spacings in the two smectic phases shown by trimer 3 were measured by SAXS () as a function of temperature. In both the SmA and SmCA phases, the layer spacing corresponds to one-third of the molecular length (d ~ 19 Å), which is consistent with a triply intercalated lamellar structure, similar to that seen for 2. Interestingly, the layer spacing in the higher temperature SmA phase was slightly smaller than in the SmCA phase. This suggests that in the SmA phase, as in the SmCA phase, the individual mesogenic cores are inclined to the layer normal, although the rapid rotation of molecules around their long axes removes the correlations of tilt direction within the layers in SmA phase. For trimer 4, the diffuse signal seen in the small angle region in the NTB phase corresponds to a length of 20 Å, which is again about one-third of the molecular length and suggests a locally triply intercalated arrangement.
Figure 6. (Colour online) The layer spacing in the smectic phases and local periodicity detected in the nematic phase of trimer 3, with sketches showing the packing of trimers in the SmCA and SmA phases.
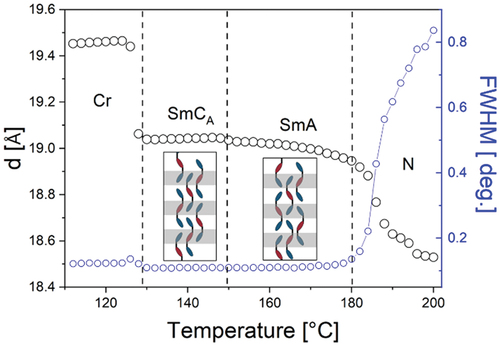
The observation of triply intercalated phases for these trimers is consistent with the reported behaviour of mesogenic trimers with central biphenyl units and outer cyanobiphenyl groups [Citation7]. This implies that hydrogen bonding between the amide groups has little effect on the phase structure. Hydrogen bonding requires the alignment of the benzanilide units of neighbouring molecules, presumably favouring their space segregation, whereas the suggested triply intercalated structure randomly mixes the cyanoterphenyl and benzanilide units. To investigate the extent of hydrogen bonding in trimers 2 and 3, IR spectra were recorded on cooling from the nematic phase to the crystal at 10 K intervals and the peak associated with the amide (I) band was carefully monitored. This band arises predominantly from the carbonyl stretching vibration and for trimer 2 at room temperature it appeared at 1641 cm−1. In the nematic phase at 190°C, the peak is shifted to 1674 cm−1. The shift to higher frequencies implies that hydrogen bonding is not as extensive in the nematic phase as in the crystal [Citation20]. As the sample is cooled into the SmCA phase, the peak shifts slightly to lower frequencies, before a relatively large jump is seen at 120°C, as the sample crystallises ( and Figure S.2a). In the variable temperature IR spectra for trimer 3 (Figure S.2b) the shift of the amide (I) band shows a similar trend, appearing at higher frequencies in the liquid crystal phases than in the crystal, as was seen for trimer 2. On cooling from the nematic phase into the SmA phase, there is a slight gradual shift of the peak maximum of the band, but no apparent step change. This would appear to suggest that the degree of hydrogen bonding, and the molecular packing is similar to that seen in the SmCA phase for 2.
For trimer 4, AFM measurements in the NTB phase revealed a periodic structure () that corresponds to a helical pitch length of 108 Å, slightly less than two molecular lengths. This is longer than the pitch length seen previously for the trimer CB6OBO6CB, of 66 Å [Citation7]. The remarkably short pitch length seen in CB6OBO6CB was attributed to the tight intercalation of the mesogenic units. It may be the case that the central benzanilide group and the lateral methyl substituent in trimer 4 are not as amenable to such a close packing as the central biphenyl unit in CB6OBO6CB.
3.3. The influence of methyl substituents
Trimer 5 has a comparable structure to 2 but without a lateral methyl substituent. On cooling from the nematic phase, smectic bâtonnets begin to develop at around 200°C () but this is very closely followed by rapid crystallisation. The growth of bâtonnets is strongly suggestive of a smectic phase, however it is not possible to determine whether this is a SmA or SmC phase due to the strong tendency of the sample to crystallise. The di-ortho substituted trimer 6 melts at 191°C into the nematic phase, before clearing at 254°C. The sample crystallises at 123°C and no additional liquid crystal phases are seen. Trimer 7 has a meta, rather than ortho, methyl substituent on the anilide ring, but otherwise has the same structure as trimer 2. It forms a nematic phase, and a second, lower temperature phase which forms a focal conic fan texture (). Shearing this phase produces a schlieren texture consistent with an anticlinic SmCA phase, as seen for 2. The layer spacing in the smectic phase is ~ 17.5 Å, very similar to that shown by 2, and again corresponding to one-third of the molecular length, implying a triply intercalated structure.
4. Discussion
4.1. A comparison of cyanobiphenyl and cyanoterphenyl-based trimers
Trimer 1 has two cyanobiphenyl groups, and replacing these by cyanoterphenyl units, giving trimer 2, increases TNI by 154 K. This increase is essentially identical to that seen between the corresponding dimers, 159 K [Citation12]. A key difference between trimers 1 and 2 is that 1 forms an NTB phase while 2 shows smectic behaviour. This is consistent with other studies showing that the cyanoterphenyl unit promotes smectic to a greater degree than NTB behaviour [Citation21].
The phase properties of trimer 1 may also be compared to those of the corresponding trimers with central benzylideneaniline (CB6O.O6CB) [Citation22], and biphenyl (CB6OBO6CB) [Citation7] units in order to better understand the influence of the 2° benzanilide group on liquid crystalline behaviour (). All three trimers form nematic and twist-bend nematic phases, suggesting that they adopt a similar ‘S’ shaped conformation. This implies that the benzanilide unit is in the Z conformer, as would be expected [Citation12]. This is shown for trimer 1 in However, both TNTBN and TNI are notably lower for 1 than for the other two trimers. This presumably reflects the disruption in the interactions between the mesogenic units arising from the lateral methyl substituent in 1, and it appears that this effect is not offset by hydrogen bonding between the amide groups. We also note that the amide link will modify the electron distribution in trimer 1, see , and hence change the net molecular dipole moment. The relative importance of these effects in determining its liquid crystal behaviour is unclear and now requires further investigation.
Table 2. Transition temperatures of trimers with benzanilide, benzylideneaniline, and biphenyl-based central mesogenic groups.
4.2. The influence of spacer length
The length of the flexible spacers in trimers 2, 3, and 4, containing cyanoterphenyl groups has the most dramatic effect on phase behaviour observed. Increasing the spacer length reduces TNI, and increases the associated entropy changes, and this is consistent with the behaviour of other trimer series that have been reported [Citation23–25].
Trimer 2 has hexyloxy spacers and forms SmCA and N phases, trimer 3 has octyloxy spacers and forms SmCA, SmA and N phases, and finally, trimer 4 with decyloxy spacers forms NTB and N phases. All these trimers pack into a triply intercalated arrangement in their smectic phases, and the periodicity measured along the director in the nematic phases also corresponds to approximately one-third of the molecular length. Increasing the length of the spacer between trimers 2 and 3 sees an increase in the stability of the smectic phase, which presumably reflects that the increased flexibility facilitates more efficient packing of molecules into layers. The formation of a SmA phase by 3 suggests that the interactions between the mesogenic units have been disrupted, at least to some extent, such that the correlated tilt within a layer is lost. Further increasing the spacer length in trimer 4 sees smectic behaviour extinguished in favour of the NTB phase, due to further dilution of the interactions between mesogenic groups that reduces the stability of the smectic phases and reveals the NTB phase.
4.3. The influence of methyl substituents
If we now consider the influence of the methyl substituents at the central mesogenic core on phase behaviour, the first factor is the effect of symmetry. The singly ortho substituted trimer 2, and the meta substituted trimer 7, show very similar phase behaviour, whereas the unsubstituted trimer 5, and the di-methyl substituted trimer 6 do not. Removing the methyl substituent in 2 to give trimer 5 leads to an increase of 31 K in TSmN. This suggests that the removal of the methyl group improves the ability of the molecules to pack into layers. By comparison, TNI is not changed by the removal of the methyl group, suggesting that a single lateral methyl group has little effect on the shape profile of these large molecules and hence does not strongly affect the interactions between the mesogenic units that govern the nematic–isotropic transition temperature.
The inclusion of two ortho methyl groups in trimer 6 leads to an increase in the melting temperature and the tendency to crystallise compared to 2, while TNI decreases by 44 K, as a result only nematic behaviour is observed. This is very similar to the behaviour seen for the corresponding mono- and di-substituted dimers reported previously [Citation12], for which the difference in TNI was 41 K. At first glance, it may appear unexpected that the inclusion of a second methyl group should have a much larger effect on TNI for 6 than the inclusion of the first methyl group did for 2. This may be accounted for, however, in terms of the effect the second methyl group has on the overall molecular shape. Due to the increased steric bulk, the addition of a second methyl ortho to the amide nitrogen leads to a twisting of the anilide ring relative to the plane of the amide bond. In 6, this twist may be amplified in the trimer structure, such that the two cyanoterphenyl units are now almost perpendicular to each other. This change in the overall shape of the trimer may account for the observed decrease in TNI on the addition of the second methyl group.
The difference in behaviour between the ortho and meta substituted compounds, 2 and 7, is much more subtle. TNI of the meta substituted compound 7 was slightly lower than that of 2, TSmN, however, was 31 K higher for 7, which suggests that moving the methyl group to the meta position relative to the amide nitrogen allows the mesogenic units to pack more efficiently in the anticlinic lamellar structure of the SmCA phase.
5. Conclusion
The liquid crystalline properties of trimers containing secondary benzanilide group appear to be primarily driven by the overall molecular shape. All seven studied trimers formed the nematic phase, with additional smectic or twist-bend nematic behaviour seen depending on their molecular structure. X-ray diffraction measurements revealed that these trimers exhibit a triply-intercalated arrangement in the smectic and nematic phases, which is consistent with the behaviour of similar trimers [Citation7,Citation26]. Variable temperature IR spectroscopic studies indicate that there is not a high degree of intermolecular hydrogen bonding present in the LC phases, in contrast to the amide-containing rod-like liquid crystals; rather the liquid crystalline properties of the materials studied here are governed by the structural features of the trimeric molecules, such as the overall shape, the size of the mesogenic units and the length of the spacers. These results demonstrate that the amide group may provide an additional tool in the design and synthesis of oligomeric liquid crystals.
SI LC trimers containing secondary amide groups.pdf
Download PDF (798.6 KB)Disclosure statement
No potential conflict of interest was reported by the author(s).
Supplementary material
Supplemental data for this article can be accessed online at https://doi.org/10.1080/02678292.2024.2382301.
Additional information
Funding
References
- Collings PJ, Hird M. Introduction to liquid crystals: chemistry and physics. London: CRC Press; 1997.
- Cestari M, Diez-Berart S, Dunmur DA, et al. Phase behavior and properties of the liquid-crystal dimer 1′′,7′′-bis(4-cyanobiphenyl-4′-yl) heptane: a twist-bend nematic liquid crystal. Phys Rev E. 2011;84(3):031704. doi: 10.1103/PhysRevE.84.031704
- Borshch V, Kim Y-K, Xiang J, et al. Nematic twist-bend phase with nanoscale modulation of molecular orientation. Nat Commun. 2013;4(1):2365. doi: 10.1038/ncomms3635
- Mandle RJ, Davis EJ, Voll C-C, et al. The relationship between molecular structure and the incidence of the N TB phase. Liq Cryst. 2014:1–16. doi: 10.1080/02678292.2014.974698
- Paterson DA, Gao M, Kim Y-K, et al. Understanding the twist-bend nematic phase: the characterisation of 1-(4-cyanobiphenyl-4′-yloxy)-6-(4-cyanobiphenyl-4′-yl)hexane (CB6OCB) and comparison with CB7CB. Soft Matter. 2016;12(32):6827–6840. doi: 10.1039/C6SM00537C
- Scarbrough AN, Tuchband MR, Korblova ED, et al. The heliconical nematic twist-bend phase from “classic” bent-core benzylideneanilines with oligomethylene cores. Mol Cryst Liq Cryst. 2017;647(1):430–438. doi: 10.1080/15421406.2017.1290396
- Tuchband MR, Paterson DA, Salamonczyk M, et al. Distinct differences in the nanoscale behaviors of the twist–bend liquid crystal phase of a flexible linear trimer and homologous dimer. Proc Natl Acad Sci USA. 2019;166(22):10698–10704. doi: 10.1073/pnas.1821372116
- Vora RA, Gupta R. A homologous series containing Ester and amide groups as central linkages: 4(4’- n -alkoxybenzoyloxy)-4”- n -Heptyloxy Benzanilides. Mol Cryst Liq Cryst. 1981;67:215–220. doi: 10.1080/00268948108070890
- Kalyvas V, Mcintyre JE. Thermotropic liquid crystal behavior in some aromatic esteramides. Mol Cryst Liq Cryst. 1982;80(1):105–118. doi: 10.1080/00268948208071024
- Zhang D, Zhou QF, Ma YG, et al. Synthesis of novel liquid crystal compounds with aromatic amide mesogenic cores. Liq Cryst. 1997;23(3):357–363. doi: 10.1080/026782997208280
- Kajitani T, Kohmoto S, Yamamoto M, et al. Generation of stable calamitic liquid-crystal phases with lateral intermolecular hydrogen bonding. Chem Mater. 2004;16(12):2329–2331. doi: 10.1021/cm049574j
- Strachan GJ, Harrison WTA, Storey JMD, et al. Understanding the remarkable difference in liquid crystal behaviour between secondary and tertiary amides: the synthesis and characterisation of new benzanilide-based liquid crystal dimers. Phys Chem Chem Phys. 2021;23(22):12600–12611. doi: 10.1039/D1CP01125A
- Strachan GJ, Majewska MM, Pociecha D, et al. Using lateral substitution to control conformational preference and phase behaviour of benzanilide-based liquid crystal dimers. Chemphyschem. 2023;24(7):e202200758. doi: 10.1002/cphc.202200758
- Walker R, Pociecha D, Strachan GJ, et al. Molecular curvature, specific intermolecular interactions and the twist-bend nematic phase: the synthesis and characterisation of the 1-(4-cyanobiphenyl-4′-yl)-6-(4-alkylanilinebenzylidene-4′-oxy)hexanes (CB6O.M). Soft Matter. 2019;15(15):3188–3197. doi: 10.1039/C9SM00026G
- Mandle RJ, Goodby JW. Does topology dictate the incidence of the twist-Bend phase? Insights gained from novel unsymmetrical bimesogens. Chem Eur J. 2016;22(51):18456–18464. doi: 10.1002/chem.201604030
- Frisch MJ, Trucks GW, Schlegel HB, et al. Gaussian 09. Wallingford (CT): Gaussian Inc.; 2016.
- Tarini M, Cignoni P, Montani C. Ambient occlusion and edge cueing to enhance real time molecular visualization. In: IEEE Trans Vis Comput Graph; 2006. p. 12. https://gaussian.com/g09citation/
- Yager K. Sci Analysis [Internet]. Brookhaven Science Associates, LLC, Brookhaven National Laboratory; 2022. Available from: https://github.com/CFN-softbio/SciAnalysis/tree/master
- Pociecha D, Crawford CA, Paterson DA, et al. Critical behavior of the optical birefringence at the nematic to twist-bend nematic phase transition. Phys Rev E. 2018;98(5):052706. doi: 10.1103/PhysRevE.98.052706
- Wanga H, Bai B, Bai FQ, et al. Direct evidence for the effect of lateral hydrogen bonding on the smectic phase. Liq Cryst. 2011;38(6):767–774. doi: 10.1080/02678292.2011.573586
- Walker R, Pociecha D, Storey JMD, et al. Remarkable smectic phase behaviour in odd-membered liquid crystal dimers: the CT6O. m series. J Mater Chem C. 2021;9(15):5167–5173. doi: 10.1039/D1TC00904D
- Majewska MM, Forsyth E, Pociecha D, et al. Controlling spontaneous chirality in achiral materials: liquid crystal oligomers and the heliconical twist-bend nematic phase. Chem Commun. 2022;58(34):5285–5288. doi: 10.1039/D1CC07012F
- Imrie CT, Henderson PA, Yeap GY. Liquid crystal oligomers: going beyond dimers. Liq Cryst. 2009;36(6–7):755–777. doi: 10.1080/02678290903157455
- Arakawa Y, Komatsu K, Shiba T, et al. Phase behaviors of classic liquid crystal dimers and trimers: alternate induction of smectic and twist-bend nematic phases depending on spacer parity for liquid crystal trimers. J Mol Liq. 2021;326:115319. doi: 10.1016/j.molliq.2021.115319
- Imrie CT, Luckhurst GR. Liquid crystal trimers. The synthesis and characterisation of the4,4′-bis[ω-(4-cyanobiphenyl-4′-yloxy)alkoxy]biphenyls. J Mater Chem. 1998;8(6):1339–1343. doi: 10.1039/a801128a
- Imrie CT, Henderson PA, Seddon JM. Non-symmetric liquid crystal trimers. The first example of a triply-intercalated alternating smectic C phase. J Mater Chem. 2004;14(16):2486–2488. doi: 10.1039/b404319g