ABSTRACT
As a follow-up to the 2008 state-of-the-art (SOTA) conference on traumatic brain injuries (TBIs), the 2015 event organized by the United States Department of Veterans Affairs (VA) Office of Research and Development (ORD) analysed the knowledge gained over the last 7 years as it relates to basic scientific methods, experimental findings, diagnosis, therapy, and rehabilitation of TBIs and blast-induced neurotraumas (BINTs). The current article summarizes the discussions and recommendations of the scientific panel attending the Preclinical Modeling and Therapeutic Development Workshop of the conference, with special emphasis on factors slowing research progress and recommendations for ways of addressing the most significant pitfalls.
Introduction
Need for TBI and blast-induced neurotrauma research
In the last several decades, traumatic brain injury (TBI) has frequently been called a ‘silent epidemic’, referring to the fact that it is the leading cause of death and disability in the most active population (<45 years of age) of industrialized countries [Citation1]. Mild TBI or concussion has been established as a considerable risk of military service as well as participation in contact sports [Citation2]. Indeed, sport-related concussions are among the leading health problems both in military and civilian young populations [Citation3]. The 2013 position paper prepared by the American Medical Society for Sports Medicine on concussion estimated that as many as 3.8 million concussions occur in the USA per year during competitive sports and recreational activities; however, as many as 50% of the concussions may go unreported [Citation4].
People involved in popular sports, including but not limited to hockey, football, wrestling, boxing, rugby, soccer, lacrosse, downhill skiing, martial arts, horse riding, and parachuting, risk exposure to brain injuries that range from asymptomatic sub-concussive blows to symptomatic concussion to moderate or severe TBI [Citation5]. Unfortunately, concussions are often repetitive. A 2003 study found that during 4251 player-seasons, 184 US college football players (6.3%) had a concussion, and 12 (6.5%) of these players had a repeat concussion within the same season [Citation6]. Accumulating evidence suggests the concussed brain is less responsive to usual neural activation, and premature cognitive or physical activity or stress before the brain completely recovers might cause prolonged dysfunction or even long-term deficits [Citation7]. A recent study [Citation8] aiming to determine if concussive events increase the incidence of depression in active semi-professional and professional North American football players showed that multiple concussive events (≥3) appear to increase the symptoms of depression.
TBI is of particular concern among military personnel due to increased risk of exposure to concussive injuries resulting from explosions or other military training- and combat-related factors such as motor vehicle crashes and falls [Citation9–Citation11]. It has been estimated that roughly 15–20% of deployed US military personnel have had a concussion/mild TBI [Citation12,Citation13]. This number could be much higher within combat units. Military-related concussions are also often repetitive. Out of 113 personnel experiencing more than one concussion between 2004 and 2008, 20% were found to have experienced the second concussion within 2 weeks of the first and 87% within 3 months after the first. A majority of these incidents were blast related [Citation14]. Indeed, blast-induced neurotrauma (BINT), i.e. brain injury caused by blast exposure(s), causes the overwhelming majority of battlefield TBIs [Citation15]. It also represents a progressively increasing threat to civilians due to a growing risk of terrorist attacks [Citation16] and a frequent use of explosives in industry (e.g. in mining and construction sites, among others). Regardless of the aetiology, while the brain might fully recover after a single insult, repeated mild-intensity insults and their accumulating effects increase the probability of post-traumatic neurological impairments [Citation17].
TBI presents across a range of clinical severities that are divided into mild, moderate, and severe. Clinically, severities are separated based primarily on the duration of loss or alteration of consciousness at the time of the injury. While injuries of all severities may lead to chronic and persistent symptoms, recently the effects of mild TBI (mTBI) have received increased attention in both civilian and military life. For example, although the recent conflicts in Iraq and Afghanistan produced the largest number of combat-related severe TBIs since the Vietnam era, most TBIs encountered in these settings were mTBIs [Citation13]. TBIs of all severities may be associated with a post-concussion syndrome that includes a combination of cognitive, somatic and affective symptoms including memory complaints, headaches and sleep disturbance. Mental health problems are common. Indeed, one striking feature of the mTBI cases being seen in the most recent veterans has been the frequent presence of post-traumatic stress disorder (PTSD) [Citation18].
The brain injury associated with TBI and BINT may be conceptualized as a combination of focal and diffuse injury [Citation19]. Focal injuries, mostly contusions and haemorrhages, are a more common feature of moderate-to-severe TBIs. By contrast, all severities of TBI are thought to involve some form of diffuse injury of which diffuse axonal injury (DAI) is the most recognized form. Although first recognized in human postmortem studies in the context of more severe injuries, modern imaging techniques suggest that some degree of DAI may be a feature of all severities of TBI.
TBI and BINT furthermore trigger complex pathological mechanisms that include elements of excitotoxicity, oxidative damage and cerebrovascular derangements [Citation20]. Cellular, sub-cellular and molecular pathological processes that become activated include decreased mitochondrial respiratory capacity (thus, energy failure), damage of liposomes, activated mechanisms of apoptotic and non-apoptotic delayed cell death, triggered cascades of inflammation, and protein degradation [Citation21–Citation23]. Additionally, both TBI and BINT induce complex metabolic changes including the metabolization of amino acids, carbohydrates and lipids that affect multiple organs, not only the brain [Citation24]. After a TBI, these systemic metabolic alterations accompanying inflammation may contribute to and/or aggravate the impaired energy production and neurotransmitter synthesis in the brain and significantly worsen the injury outcome [Citation25]. The prominence of specific cellular and molecular processes may vary with the severity of injury and evolve as the brain injury progresses from the acute into the subacute and chronic phases. The pathophysiological cascades activated by TBI and BINT may also differ [Citation16].
Neurological and behavioural abnormalities observed in patients with TBI or BINT may be transient or permanent, and are the visible consequences of the cellular, sub-cellular and molecular pathological processes and their evolution with time. Symptoms associated with moderate-to-severe TBI may improve spontaneously, but are often associated with long-lasting cognitive and motor deficits. While the post-concussion syndrome associated with mTBI may resolve spontaneously, in many veterans, symptoms become chronic and persistent [Citation26,Citation27]. More recently, it has also become recognized that TBI is a risk factor for the later development of neurodegenerative diseases including Alzheimer’s disease and chronic traumatic encephalopathy [Citation28].
Despite intensified research efforts and fortified support, methods that would provide early and reliable insights into the progress of TBI/BINT-induced brain damage, or a magic cocktail that would prevent delayed brain damage and/or replace or regenerate damaged cells after injury, have yet to be developed. Our knowledge of the pathobiology of TBI/BINT (including mechanisms of neuronal cell death, neurodegeneration and inflammation in the brain) is gradually increasing, but we are still far from having effective neuroprotective therapies that would help the large number of people who suffer from debilitating effects of TBI.
Progress in TBI research
Because of the personal and financial costs caused by TBIs/BINTs, the US Departments of Defense (DoD) and Veterans Affairs (VA) are allocating substantial financial and intellectual support to experimental and clinical research efforts aiming at better understanding of the essential mechanisms of brain injuries and the development of novel diagnostic and treatment approaches. This funding commitment has boosted the number of experimental studies focusing on TBI and BINT. shows the number of articles using rat () or mouse () TBI or BINT models published between 2008 and the first half of 2016. The productivity of this period is considerable: approximately 5200 articles used a rat model and around 3000 a mouse model to address some aspects of TBI, with 195 rat and 108 mouse studies attempting to replicate the features of human BINT. The progress is more obvious when we compare the productivity of the 2008–2016 period with the research outputs of the previous 7 years (1999–2007): roughly 3914 articles with a rat TBI model, 27 with a rat BINT model (), 1172 with a mouse TBI model, and only four with a mouse BINT model (). These statistics indicate around a 33% increase in rat and 155% increase in mouse TBI studies. The 2008–2016 period has seen a staggering rise in blast injury and BINT research. Between 1999 and 2007, rat and mouse BINT research projects represented only a fragment (0.69% or 0.34%, respectively) of the rodent studies focusing on some aspects of TBIs (including BINT), a number which increased by a factor of 10 over the next 7 years.
Figure 1. Number of articles using (A) rat or (B) mouse TBI or BINT models published between 2008 and the first half of 2016.
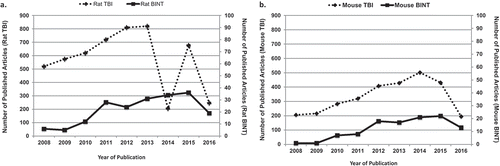
Figure 2. Number of articles using (A) rat or (B) mouse TBI or BINT models published between 1999 and 2007.
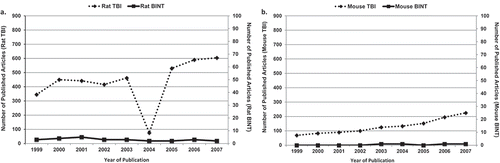
In addition to the publications as a measure of intensified research on TBI/BINT, the impressive financial support awarded by the National Institutes of Health for projects addressing any aspects of traumatic brain injury research during the 2008–2015 period further demonstrates the institutional commitment towards neurotrauma research. Indeed, over $509 M has been spent for 1,369 projects and 136 sub-project addressing some aspects of TBI/BINT.
Since rodents are the most frequently used animals in preclinical TBI modelling, these statistics are an index to the increasing interest in preclinical studies addressing the multifaceted nature of TBI and BINT. Unfortunately, the translation of promising leads suggested by experimental findings into clinical applications for better diagnosis or therapy [Citation29–Citation32] has failed. Thus, there is an obvious discrepancy between the intensified efforts invested in research generating a vast amount of information about TBI and BINT and the failure to develop clinically relevant neuroprotective strategies. The inevitable question is what (if anything) went wrong? Why has the increasing number of studies focusing on TBI and BINT failed to provide the critical mass of information sufficient to prompt the development of clinically and militarily relevant and applicable diagnostic, treatment and rehabilitation tools?
Problems and challenges
The Preclinical Modeling and Therapeutic Development Workshop attendees included professionals with multidisciplinary backgrounds: basic biomedical researchers, engineers, computer scientists, clinicians, clinical scientists, and stakeholders, among others. During the lively discussion, the panel outlined their views about the status quo and pitfalls of current TBI and BINT research. The text below provides a synthesis of the discussion.
Preclinical models provide useful information about TBI and BINT, but in their current forms, they do not adequately mimic the pathophysiology of human injury and often fail to replicate the clinical impairments seen in patients.
Animals used in research
We experimentally create TBIs or BINT in animals in aseptic environments using highly controlled protocols. The end result is that most animal models are highly reproducible and predictable, allowing for manageable group sizes from a statistical perspective. The great majority of preclinical studies use rodents. Indeed, many researchers have accepted rodent models as the most suitable choice for neurotrauma research: the relatively small size, short lifespan and low cost of rodents permit repetitive measurements of morphological, biochemical, cellular and behavioural parameters. Such models are certainly desirable from a logistical perspective: besides using a relatively small group size, they allow humane and economical use of animals and reproduce pathological processes caused by injury more consistently because of their same or similar genetic backgrounds. In comparison, studies with phylogenetically higher species with gyrencephalic brains are less approachable due to ethical, technical, and/or financial limitations. However, despite their many advantages, the use of animals with a lissencephalic cortex has limitations that are often not considered in the experimental design and data interpretation of the research. Because of the vast differences in geometry, metabolism, anatomy, and neurobiology between rodents and humans, it often happens that rodents, which survive even the most severe TBIs (such as those induced in the rat penetrating brain injury model) outwardly appear to perform well across a spectrum of behavioural, physiological and cognitive domains within days post injury. Such ‘recovery’ is virtually never seen in patients with a severe TBI. It is clearly arguable that, beyond the primary injury/insult, animal models fail to represent the real-life circumstances of a TBI or responses to blast exposure. It was the consensus of the panel that we need to make our models less ‘sterile’ and more clinically relevant. In addition, rarely is a moderate-to-severe TBI an isolated injury to the head – the incidence of clinical polytrauma and its influence on the progress and outcome of TBI is significant but not as well studied as it should be in preclinical experiments.
Approach to experimental modelling
Considering the complexity of the pathobiology of TBIs and BINT, existing animal models do not accurately represent the clinical entity of human head injuries, reproducing only a very limited set of pathological impairments. Different research groups have differing research targets: preclinical models tend to be reductionistic and reproduce only highly targeted pathological changes, while overlooking the broad spectrum of TBI/BINT outcomes seen in patients. Ultimately, such an approach results in a research field where inter-laboratory comparisons become difficult if not impossible.
Many laboratory researchers prefer to use already existing sets of experimental models legitimized by publication and consensus as bone fide tools for TBI research, regardless of whether those models approximate the complexity seen in clinical TBI/BINT. Updated versions of these models are often just technologically improved forms of the older ones and rarely change the basic premises of the original model. Developing a novel model that fulfils all the criteria of scientific stringency while having clinical relevance is a long and painstaking process, which usually does not bring much reward in terms of publications or government grants. Researchers interested in TBI/BINT and related pathological consequences routinely choose existing models, which do not necessarily reproduce the main features of the clinical syndrome. Because of the highly targeted research approach fostered in a technological society, the models are mainly developed from an academic perspective to dissect the minutiae of a distinct pathological pathway, rather than to provide a global understanding of an ailment in patients. The current field of neuroscience research is dominated by highly reductionistic, molecular/biological paradigms, and many academic scientists consider translational research to be better left to the pharmaceutical and medical appliance industries.
Lack of understanding of the biomechanics of traumatic brain injury and blast-induced neurotrauma
Improved diagnosis, treatment and rehabilitation of TBI and BINT hinges on how well we grasp the interactions between the injurious forces and the human body/head. Gaining new knowledge about unknown biomechanical and biomedical processes requires development and utilization of experimental models capable of reproducing both the injurious factors and the related pathological consequences seen in humans. However, the physics of the injury scenarios that occur in humans are not well understood. Thus, biomechanical factors frequently remain insufficiently characterized, and their relationships to pathological changes are vague.
The panel argued that establishing a causal relationship between the mechanical factor inflicting injury and its clinical outcomes is crucial in developing appropriate translational models of brain damage. Regardless of the research questions to be addressed, clinically and militarily relevant TBI/BINT models should satisfy the following criteria: (1) the injurious factor should be clearly identified and reproduced in controlled, reproducible, and quantifiable manner; (2) the inflicted injury should be reproducible, quantifiable and mimic the pathophysiology or symptomatic components of human TBI/BINT; (3) the injury outcome established should be based on morphological, physiological, biochemical and/or behavioural parameters related to the chosen injurious component of the blast or the mechanical force; and (4) the mechanical properties (force, acceleration, deceleration, impact, velocity, duration, etc.) of the injurious factor should predict the outcome severity [Citation33]. Nevertheless, the majority of currently used experimental TBI/BINT laboratory models fail to fulfil these criteria, which makes inter-laboratory data comparison and collaboration very difficult.
Indeed, a comparison of shock tube BINT models reported in the existing literature is almost impossible: the overwhelming majority of the articles do not provide sufficient information about the shock tube device, shockwave characteristics and/or specimen size and positioning. Without these particulars, no scientifically sound conclusions can be made on essential blast-induced pathological changes and their spatial and temporal profiles. Moreover, comparing findings that have been generated by using widely differing shock tubes (hence, dissimilar shockwave patterns) and conflicting strategies in animal positioning could generate misleading research directions.
The criteria for proper allometric scaling and translation from animal models to humans are poorly defined.
The pharmacokinetics and pharmacodynamics of research drugs significantly differ in animal models due to their considerable biological and physiological differences compared to humans. The majority of research studies do not address this issue.
Our experimental ‘treatment’ protocols need a greater shift away from the classical neuroprotection paradigms of experimental research studies focusing on acute and short-term dosing. In clinical practice, we never stop treating patients before their ailment is healed, or before we are assured that a therapy has achieved its maximal benefit. Yet, virtually all animal studies employ a fixed treatment paradigm. If the study’s primary outcome is mortality, then the treatment should be given until all the animals in the vehicle-treated group die. If a research project uses a cognitive metric to assess injury outcome, then why do the majority of studies not extend the treatment right up to the point of cognitive testing?
Considering these and other discrepancies between the clinical problems caused by TBI/BINT and the experimental models that attempt to reproduce them, the failure of therapies that showed promise in experimental animals but lacked the desired effects in humans should not come as a surprise. Frankly, we cannot put all the blame on the preclinical models when our experimental and clinical protocols ignore the basic principles of pharmacokinetics/pharmacodynamics of a drug’s action. One recent example can be seen in the disappointing results of the clinical trials for progesterone. The dosing used in the clinical trials was not based on allometric scaling, so patients received a sixfold higher dose of the test drug than should have been used based on the differences between metabolic rate in rats and humans. This may have been one of the reasons that the clinical outcomes did not replicate those seen in preclinical rodent studies [Citation34].
Recommendations
1. Preclinical models should be developed based on clinical observations and should reproduce essential patient symptom complexes including multi-organ changes that occur simultaneously with neurological changes, whether due to blunt, impact TBI or BINT. To provide a preclinical-clinical research continuum, translational neurotrauma research must achieve closer collaboration between scientists and physicians.
1.1. To improve existing preclinical models and/or develop new ones, animal research should take into account validated, clinical observations on the natural history of outcomes in patients and attempt to reproduce them in preclinical models. The injury continuum from acute to chronic TBI/BINT should be observed in clinical studies and adequately reproduced in animal models, while taking into account differences in age, size and sex needed for proper scaling.
Clinically relevant biometrics including biomarkers, imaging and quantitative behavioural outcome measures should be synchronized between clinical observations and preclinical models. The biometrics would enable testing to determine whether the changes seen in preclinical animal models occur in humans with TBI or BINT. Functional assessments in standardized preclinical models should include a breadth of longitudinal quantitative biometric measures (e.g. cognitive assessment, spasticity measurement, balance, sleep architecture, and pupillometry, among others) that are sufficiently powered to detect incremental changes in the recovery trajectories of multiple disabilities. Further, these assessments should include measures that can be practically and rapidly translated for clinical use.
1.2. The design of a preclinical model should aim to reproduce the spectrum of severities seen in human TBI/BINT. While the term “mild” TBI (mTBI) is frequently used in the preclinical literature, it is typically applied without any critical sense of whether the injury under study represents an equivalent to human mTBI. Although some agreement exists about the clinical definition of mTBI in humans, only a few experimental studies have tried to mirror these conditions in animals. Indeed, there are still no clearly defined criteria for mild, moderate and severe TBI/BINT in animals and unfortunately, this is also true in humans. A disorder that is considered ‘mild’ but that leads to long-term morphological and functional impairments probably needs a better definition and consensus on what the term ‘mild’ actually means.
Preclinical model development should also mirror operational conditions in the military and civilian environments where the trauma was experienced. Attention should be given to developing models that exhibit the chronic and persistent effects of TBI/BINT that are so troublesome to veterans and apparently to many athletes engaged in contact sports from an early age. This should include the full range of cognitive as well as behavioural features associated with acute and chronic TBI/BINT. Traits related to mental health disorders such as PTSD are of particular importance. Preclinical models that can explore the relationship between psychological stressors and physical injury should also be developed. For example, preclinical models would seem ideal for exploring the relationship between BINT and PTSD. Studies using established animal models of Alzheimer’s pathology or human tauopathies might also shed light on the role of TBI as a factor contributing to chronic human neurodegenerative diseases. Preclinical models may also make pathophysiological predictions that can be translated to human studies. Animal models have, for example, contributed significantly to recent recognition of the role of inflammation in the effects of chronic physical impact TBI [Citation35,Citation36] and vascular effects in BINT [Citation31,Citation37].
1.3. Common data elements (CDEs) should be defined for clinical TBI/BINT and implemented as guiding principles for corresponding preclinical models. The inability to compare data across laboratories is recognized as a significant limitation of preclinical TBI/BINT research [Citation8]. This situation has resulted from many factors: most obviously, because of the differences in the species, injury mechanisms targeted and in the outcomes assessed. Laboratory studies using highly quantified measures such as gait, sensory perception and detailed cognitive testing may need to be replicated in clinical studies. Additionally, clinical studies should supplement (or replace) very blunt, highly subjective quality of life measures such as the Extended Glasgow Outcome Scale (GOS-E) currently in vogue, which do not detect the subtle changes in function that can be measured with more quantitative assessments [Citation38].
More subtle problems include the variability in the nature and extent of injury that can result even when the same injury model is used in different laboratories. The National Institute of Neurological Disorders and Stroke and several co-sponsoring federal agencies have lead the way in developing CDEs for human TBI research (https://commondataelements.ninds.nih.gov/tbi.aspx). The first set of human CDEs developed by a work group in 2010 focused on acute TBI in adults. A second version developed in 2012 expanded human CDEs to include data relevant to epidemiological research, rehabilitation of moderate/severe TBI and mTBI/concussion research [Citation39].
Recently, a workgroup was tasked with creating a set of CDEs for preclinical TBI research [Citation40]. The proposed preclinical CDEs were divided into core data elements that should be relevant to most if not all studies. The workgroup also proposed model-specific recommendations for nine of the more commonly used preclinical models including weight drop, fluid percussion, controlled cortical injury and BINT. The workgroup avoided making prescriptive judgements concerning parameters for individual models, but rather stressed the need for standardized documentation and adoption of a common language for preclinical TBI research. The VA and other federal agencies supporting research should continue to encourage the development and adoption of CDEs in preclinical TBI research in a non-prescriptive way.
1.4. The preclinical model should provide acceptable alignment between the mechanical properties of the injurious factor and the biological consequences of the force-body/head interaction as seen in real-life scenarios involving humans.
A critical lack of information exists regarding the relationship between biomechanical injury thresholds and pathogenic cascades that predict long-term consequences of TBI/BINT [Citation33]. Despite recent advances, no biomechanical injury thresholds have been developed that are capable of accurately predicting chronic deficits caused by TBI/BINT, and evaluating influences of pre-existing biological conditions. A combined biomechanics/physiology approach is paramount to revolutionize our understanding of the pathophysiological mechanisms underlying neurological deficits following TBI and the development of novel therapeutic strategies for those suffering from debilitating effects of TBI. Hence, it is recommended to use multiple, both non-biological/surrogate and biological (in vitro, ex vivo, in vivo) experimental models of rotational, impact, penetrating or blast-related TBI in a highly orchestrated manner. The steps of such a synchronized approach would include the following:
Using biofidelic human and animal surrogates with the latest generation of sensors implanted in the organs to characterize the injury factors such as the kinetic energy of the impact, acceleration/deceleration, rotational forces and pressure, among others.
Then, reproducing the defined conditions in biological models (in vitro and/or in vivo, tissue and/or whole body), and characterizing, standardizing and validating biological outcome measures that show, for example, the extent of cell death and cellular damage, the intensity of triggered inflammation or degeneration, or degree of functional deficits;
Additionally, in established animal models of TBI/BINT with clearly outlined biological outcome measures, analysing potential synergistic effects between the injury-modifying factors (such as gender, age, emotional and environmental stressors, and length of observed post-traumatic period) and biomechanical factors of injury; and
Finally, performing a critical comparison between prevalent human injury scenarios and experimental set-ups, and—if necessary—fine-tuning the experimental models to match the clinically relevant situations.
The extrapolation from surrogate and animal experimental data to clinical TBI/BINT will enable us to test the translational value of research studies to predict long-term neurobehavioral dysfunction due to TBI/BINT, and decisively guide the development of therapeutic strategies.
1.5. TBI and BINT dosimetry-criteria capable of determining injury thresholds for both acute and chronic complications should be established for both clinical settings and preclinical models.
For BINT, while the development and fielding of a reliable dosimeter with a valid concussion threshold is the ultimate, long-term goal, what is needed in the near term is a simple and reliable means for measuring and recording blast-related exposures, including information about overpressure, pressure profile, impact, acceleration and rotation. Far too many studies are based purely on assumptions about the type and the magnitude of blast-related insults that service members are exposed to in training and in combat. While the DoD has undertaken several initiatives in developing field sensors, including helmet sensors and blast gauges, these initiatives have had very limited success. Greater emphasis needs to be placed on the development and fielding of a technology for characterizing actual exposures that is reliable, accurate and transparent to service members. There should also be more of an emphasis on the effects of repeated, low-level primary blast exposures, such as those related to weapons firing on brain functions. This is a research topic that has not been adequately addressed despite the fact that many service members are routinely exposed to these ‘occupational’ levels of blast in training and combat. The research should focus on determining safe exposure limits to ensure that we are not injuring service members with their own weapons. Also, emphasis is needed on the importance of an appropriate use of shock tubes and other laboratory devices to ensure that test subjects are receiving relevant blast exposures. Investigators should team with blast physicists and engineers with expertise in blast physics and design of blast generators.
For blunt TBI, vital biomechanics should be clarified and information about the circumstances of injury (in clinics) and impact/delivered energy, acceleration, and rotation (in preclinical models) should be provided.
The panel recommends that, like the CDEs, a consensus document on the dosimetry-criteria for all types of TBI should be established, and its utilization in research grants and scientific papers reinforced.
1.6. There was an agreement that the existing clinical observations and experimental findings should prompt more studies that would address:
Mechanisms of plasticity, recovery and decompensation;
The role of inflammation in the chronic effects of TBI/BINT;
The neurobiological basis of TBI/BINT-related behavioural changes, TBI/BINT-stress interactions, and the similarities/differences between TBI/BINT and stress; and
The full spectrum of clinical manifestations that cross traditional clinical domains (e.g. PTSD and psychiatry) to better understand the physiological processes that stem from TBI sustained while on active duty.
2. Clinical trials testing drugs that have been established as successful in preclinical studies should use more stringent stratification principles to ensure that a drug is tested for its ability to attenuate the same pathological/pathophysiological process in the patient as observed in the preclinical animal model. If this is not done, wide heterogeneity in the types of TBI combined with the effects of inescapable injury-induced complications in patients can have the potential to mask beneficial effects of treatment.
2.1. Treatment approaches should be tested in preclinical models for their ability to attenuate the clinically important forms of pathology and pathophysiology seen in patients (i.e. target engagement strategy). The optimal treatment strategy The optimal treatment should include all measures relevant to the acute and chronic effects of TBI/BINT, and take into account the systemic effects of poly-trauma/TBI. Thus, the assessments should include cognitive and behavioural measures as well as any known biochemical, physiological or pathological alterations relevant to the underlying pathophysiology. Strategies might include analysis of serum biomarkers, brain neurotransmitters and the application of advanced neuroimaging techniques. When experimental drugs are tested, targets known or suspected as being affected by drug action should be assessed. Approaches should be focused on those targets with the most direct clinical importance.
2.2. The panel recommends the creation of VA- and/or NIH-supported centres for providing pretrial screening to reduce large variance among participants enrolled in clinical studies and ensure a higher-level compliance with CDEs. Stratification strategies might include selection based on genetic predispositions to respond to the selected treatment, gender or epigenetic changes due to specific environmental exposures, among others. One model for such centres already in place is the VA Cooperative Studies Programme, which plans and conducts large multicentre clinical trials and epidemiological studies funded by the Department of VA. The Cooperative Studies Programme provides a clinical research infrastructure that includes data and statistical coordinating centres, a clinical research pharmacy-coordinating centre and epidemiological resource centres. Consideration should be given to expanding this programme to include collaborative efforts with other federal agencies including NIH and DoD as well as academic and industry partners or creating new interagency initiatives.
3. Novel organizational structures are needed to provide overarching guidance supporting the preclinical-clinical research continuum and the clinically and militarily relevant research studies.
3.1. New funding platforms for long-term financial research support are needed that would enable chronic studies with preclinical models and foster more collaboration across disciplines.
3.2. DoD/VA-sponsored training centres should be organized to teach best-practice preclinical and clinical research methods addressing TBI and BINT in a synchronized manner, and foster joint research efforts involving clinicians and basic science researchers. After undergoing joint training, the teams should have access to special funding mechanisms to support collaborative projects.
3.3. Develop both preclinical and clinical tissue and fluid (blood, urine, cerebrospinal fluid and saliva) banks for TBI/BINT, and establish an organizational structure for synchronized sample and knowledge sharing. Accepting biological materials only from sources that provide full information about CDEs and TBI/BINT dosimetry-criteria ensures synchronization of the clinical-preclinical research continuum, and significantly reduces the generation of misleading research data that slows down the progress of neurotrauma research.
Declaration of Interest
The authors report no declarations of interest.
Disclaimer
The views and opinions expressed in this article are those of the authors and do not necessarily reflect the official policy or position of any institution, organization or government agency.
References
- Hyder AA, Wunderlich CA, Puvanachandra P, Gururaj G, Kobusingye OC. The impact of traumatic brain injuries: a global perspective. NeuroRehabilitation. 2007;22(5):341–53.
- Holtkamp MD, Grimes J, Ling G. Concussion in the military: an evidence-base review of mTBI in US military personnel focused on posttraumatic headache. Curr Pain Headache Rep. 2016;20(6):37.
- Giannotti M, Al-Sahab B, McFaull S, Tamim H. Epidemiology of acute head injuries in Canadian children and youth soccer players. Injury. 2010;41(9):907–12.
- Harmon KG, Drezner JA, Gammons M, Guskiewicz KM, Halstead M, Herring SA, Kutcher JS, Pana A, Putukian M, Roberts WO. American Medical Society for Sports Medicine position statement: concussion in sport. Br J Sports Med. 2013;47(1):15–26.
- Stern RA, Riley DO, Daneshvar DH, Nowinski CJ, Cantu RC, McKee AC. Long-term consequences of repetitive brain trauma: chronic traumatic encephalopathy. PM R. 2011;3(10 Suppl 2):S460–7.
- Guskiewicz KM, McCrea M, Marshall SW, Cantu RC, Randolph C, Barr W, Onate JA, Kelly JP. Cumulative effects associated with recurrent concussion in collegiate football players: the NCAA Concussion Study. JAMA. 2003;290(19):2549–55.
- Witcher KG, Eiferman DS, Godbout JP. Priming the inflammatory pump of the CNS after traumatic brain injury. Trends Neurosci. 2015;38(10):609–20.
- Pryor J, Larson A, DeBeliso M. The prevalence of depression and concussions in a sample of active north american semi-professional and professional football players. J Lifestyle Med. 2016;6(1):7–15.
- Gubata ME, Packnett ER, Blandford CD, Piccirillo AL, Niebuhr DW, Cowan DN. Trends in the epidemiology of disability related to traumatic brain injury in the US army and marine corps: 2005 to 2010. J Head Trauma Rehabil. 2014;29(1):65–75.
- Bryan CJ, Clemans TA. Repetitive traumatic brain injury, psychological symptoms, and suicide risk in a clinical sample of deployed military personnel. JAMA Psychiatry. 2013;70(7):686–91.
- Voss JD, Connolly J, Schwab KA, Scher AI. Update on the epidemiology of concussion/mild traumatic brain injury. Curr Pain Headache Rep. 2015;19(7):32.
- Hoge CW, Goldberg HM, Castro CA. Care of war veterans with mild traumatic brain injury-flawed perspectives. N Engl J Med. 2009;360(16):1588–91.
- Hoge CW, McGurk D, Thomas JL, Cox AL, Engel CC, Castro CA. Mild traumatic brain injury in U.S. Soldiers returning from Iraq. N Engl J Med. 2008;358(5):453–63.
- MacGregor AJ, Dougherty AL, Morrison RH, Quinn KH, Galarneau MR. Repeated concussion among U.S. military personnel during Operation Iraqi Freedom. J Rehabil Res Dev. 2011;48(10):1269–78.
- Nelson C, St Cyr K, Weiser M, Gifford S, Gallimore J, Morningstar A. Knowledge gained from the brief traumatic brain injury screen—implications for treating Canadian military personnel. Mil Med. 2011;176(2):156–60.
- Patel HD, Dryden S, Gupta A, Ang SC. Pattern and mechanism of traumatic limb amputations after explosive blast: experience from the 07/07/05 London terrorist bombings. J Trauma Acute Care Surg. 2012;73(1):276–81.
- Rosenfeld JV, McFarlane AC, Bragge P, Armonda RA, Grimes JB, Ling GS. Blast-related traumatic brain injury. Lancet Neurol. 2013;12(9):882–93.
- Elder GA, Stone JR, Ahlers ST. Effects of low-level blast exposure on the nervous system: is there really a controversy? Front Neurol. 2014;5:269.
- McGinn MJ, Povlishock JT. Cellular and molecular mechanisms of injury and spontaneous recovery. Handb Clin Neurol. 2015;127:67–87.
- Barkhoudarian G, Hovda DA, Giza CC. The molecular pathophysiology of concussive brain injury—an update. Phys Med Rehabil Clin N Am. 2016;27(2):373–93.
- Gilmer LK, Roberts KN, Joy K, Sullivan PG, Scheff SW. Early mitochondrial dysfunction after cortical contusion injury. J Neurotrauma. 2009;26(8):1271–80.
- Pandya JD, Pauly JR, Sullivan PG. The optimal dosage and window of opportunity to maintain mitochondrial homeostasis following traumatic brain injury using the uncoupler FCCP. Exp Neurol. 2009;218(2):381–9.
- Arun P, Abu-Taleb R, Oguntayo S, Wang Y, Valiyaveettil M, Long JB, Nambiar MP. Acute mitochondrial dysfunction after blast exposure: potential role of mitochondrial glutamate oxaloacetate transaminase. J Neurotrauma. 2013;30(19):1645–1651.
- Jeter CB, Hergenroeder GW, Ward NH, 3rd, Moore AN, Dash PK. Human mild traumatic brain injury decreases circulating branched-chain amino acids and their metabolite levels. J Neurotrauma. 2013;30(8):671–9.
- Cernak I. The importance of systemic response in the pathobiology of blast-induced neurotrauma. Front Neurol. 2010;1:151.
- Peskind ER, Brody D, Cernak I, McKee A, Ruff RL. Military- and sports-related mild traumatic brain injury: clinical presentation, management, and long-term consequences. J Clin Psychiatry. 2013;74(2):180–8; quiz 188.
- Bazarian JJ, Cernak I, Noble-Haeusslein L, Potolicchio S, Temkin N. Long-term neurologic outcomes after traumatic brain injury. J Head Trauma Rehabil. 2009;24(6):439–51.
- Elder GA. Update on TBI and cognitive impairment in military veterans. Curr Neurol Neurosci Rep. 2015;15(10):68.
- Hellewell S, Semple BD, Morganti-Kossmann MC. Therapies negating neuroinflammation after brain trauma. Brain Res. 2016;1640(Pt A):36–56.
- Stein DG. Progesterone in the treatment of acute traumatic brain injury: a clinical perspective and update. Neuroscience. 2011;191:101–106.
- Ahmed F, Cernak I, Plantman S, Agoston DV. The temporal pattern of changes in serum biomarker levels reveal complex and dynamically changing pathologies after exposure to a single low-intensity blast in mice. Front Neurol. 2015;6(114):1–13.
- Jeter CB, Hergenroeder GW, Hylin MJ, Redell JB, Moore AN, Dash PK. Biomarkers for the diagnosis and prognosis of mild traumatic brain injury/concussion. J Neurotrauma. 2013;30(8):657–70.
- Cernak I. Animal models of head trauma. NeuroRx. 2005;2(3):410–22.
- Howard RB, Sayeed I, Stein D. Suboptimal dosing parameters as possible factors in the negative Phase III clinical trials of progesterone in TBI. J Neurotrauma. 2015. doi:10.1089/neu.2015.4179
- Faden AI, Wu J, Stoica BA, Loane DJ. Progressive inflammation-mediated neurodegeneration after traumatic brain or spinal cord injury. Br J Pharmacol. 2016;173(4):681–91.
- Nimmo AJ, Cernak I, Heath DL, Hu X, Bennett CJ, Vink R. Neurogenic inflammation is associated with development of edema and functional deficits following traumatic brain injury in rats. Neuropeptides. 2004;38(1):40–7.
- Elder GA, Gama Sosa MA, De Gasperi R, Stone JR, Dickstein DL, Haghighi F, Hof PR, Ahlers ST. Vascular and inflammatory factors in the pathophysiology of blast-induced brain injury. Front Neurol. 2015;6:48.
- Stein DG. Embracing failure: what the Phase III progesterone studies can teach about TBI clinical trials. Brain Inj. 2015;29(11):1259–72.
- Hicks R, Giacino J, Harrison-Felix C, Manley G, Valadka A, Wilde EA. Progress in developing common data elements for traumatic brain injury research: version two—the end of the beginning. J Neurotrauma. 2013;30(22):1852–61.
- Smith DH, Hicks RR, Johnson VE, Bergstrom DA, Cummings DM, Noble LJ, Hovda D, Whalen M, Ahlers ST, LaPlaca M, et al. Pre-clinical traumatic brain injury common data elements: toward a common language across laboratories. J Neurotrauma. 2015;32(22):1725–35.