ABSTRACT
Objective: We explored the effects of playing the piano on patients with cognitive impairment after mild traumatic brain injury (mTBI) and, addressed the question if this approach would stimulate neural networks in re-routing neural connections and link up cortical circuits that had been functional inhibited due to disruption of brain tissue. Functional neuroimaging scans (fMRI) and neuropsychological tests were performed pre–post intervention. Method: Three groups participated, one mTBI group (n = 7), two groups of healthy participants, one with music training (n = 11), one baseline group without music (n = 12). The music groups participated in 8 weeks music-supported intervention. Results: The patient group revealed training-related neuroplasticity in the orbitofrontal cortex. fMRI results fit well with outcome from neuropsychological tests with significant enhancement of cognitive performance in the music groups. Ninety per cent of mTBI group returned to work post intervention. Conclusion: Here, for the first time, we demonstrated behavioural improvements and functional brain changes after 8 weeks of playing piano on patients with mTBI having attention, memory and social interaction problems. We present evidence for a causal relationship between musical training and reorganisation of neural networks promoting enhanced cognitive performance. These results add a novel music-supported intervention within rehabilitation of patients with cognitive deficits following mTBI.
Introduction
Musical training has emerged as a useful framework for the investigation of training-related plasticity in the human brain (Citation1). Playing an instrument simultaneously receives and transmits visual, auditory, and kinaesthetic information to a specialised brain network (Citation2), engages emotion and reward systems in the brain, which may facilitate and enhance therapeutic approaches aimed towards rehabilitation from neurological and psychiatric disorder (Citation3–Citation8).
The brain is a dynamically organised structure that changes and adapts as response to repeatedly performed actions and has shown that temporally coherent inputs in competitive neural networks change the efficiency, density, and connectivity of synapses (Citation9). Music-making provides an enriched environment for the brain in promoting dendritic sprouting that is fundamental for synaptic plasticity (Citation10). Multisensory integration during practise enhances training-related changes in sensory and association cortical areas during auditory cognition (Citation1), and causes both structural changes in white matter and functional neuroplasticity, described as a change in the brain’s interconnected processing. Music may engage and link up brain areas that otherwise would not connect with each other (Citation10). There is increasing evidence that music making could be a possible tool in neurologic rehabilitation (Citation1,Citation11,Citation12).
Traumatic brain injury (TBI) has been recognised as a major public health problem worldwide and can lead to neurological, physiological, cognitive, psychological and social dysfunction (Citation13). TBI is traditionally classified from mild to moderate to severe (Citation14). A study of hospital-treated TBI in Oslo, Norway, reported 9000 TBI patients hospitalised every year. A total of 79–90% of all treated brain injuries are mild (Citation13,Citation14). Milder injuries may however be underestimated. In studies that based their incidence rates on hospital-treated TBI, revealed that fewer patients with mild TBI attend emergency departments and more patients are treated in outpatient settings (Citation15,Citation16). This means that the number of TBI patients diagnosed with mild TBI might be higher. Milder injuries to the head may cause microscopic damage to axons, dendrites and synapses. Diffuse axonal injury (DAI) is a typical brain damage as result of TBI. DAI is a widespread disruption of brain tissue and affects interconnected processing in the brain (Citation17). This category of patients with TBI are often inhibited from normal functioning and may benefit from music-supported cognitive rehabilitation as they can employ their rest capacity in playing an instrument in facilitating neurorehabilitation.
There is little knowledge about the potential neuroplastic changes induced by musical training in cognitive rehabilitation of patients with TBI. The scientific evidence for effectiveness of music to improve cognition (Citation18) is weak and there are no studies examining the improved functions over time (Citation3). In contrast, there is extensive research confirming the benefits of music-supported therapy in other areas of neurologic music therapy. In patients with non-fluent aphasia after left frontal lobe damage there is reactivation of inhibited language-related brain regions in right prefrontal areas after intense melodic intonation therapy (Citation19). Results from motor-rehabilitation in stroke patients give evidence for music-supported plasticity in cortical networks (Citation3,Citation4,Citation12,Citation20). Patients with Parkinson’s Disease have stabilised and synchronised their disturbed gait with the help of external auditory rhythm (Citation21).
The aim of the present study is to investigate the effects of musical training on the brain’s neural networks in respect of reorganising neural circuits and thereby restore cognitive functions in patients suffering from cognitive deficits after mild TBI. We address the question if learning to play an instrument may stimulate the neural networks in re-routing neural connections and link up inhibited neural networks. More specifically, we examined whether this intervention reconnects areas that were disconnected or inhibited through minor damage to axons, dendrites or synapses. We developed a longitudinal design that enabled us to observe the causal influence of multisensory training on higher-order auditory cognition and possible functional and structural changes in the brain’s neural networks.
Methods
Participants
Three groups of participants were recruited for the study:
Group 1: 7 patients with mTBI receiving piano tuition; Group 2: control group of 11 healthy participants with music; Group 3: control group of 12 healthy participants without music. Musicians were excluded in avoiding the possible confounding variable regarding neural brain differences in musicians and non-musicians (Citation23). Participant’s gender and age distribution: Group 1: 4 male (m) and 3 female (f), mean age (m.a.) 38 years; Group 2: m = 5 and f = 6, m.a. 33 years; Group 3: m = 4 and f = 7, m.a. 33 years. Patients with a medical history of psychological problems were excluded. The patients were recruited by invitation, and seven patients enrolled. Participants in the two control groups were recruited by posters located at University of Bergen and at Haukeland University Hospital Bergen. Participants received detailed information about the aim of the study, assessment with pre–post fMRI and neuropsychological tests and signed a written consent with information that they can withdraw from the study at any time.
Patients – clinical data
All patients were diagnosed mild traumatic injury (mTBI), as indicated by the Rivermead Post Concussion Symptoms Questionnaire (RPQ) (). Inclusion criteria was 2 years post injury to omit self-recovery in the acute phase.
Table 1. Scores obtained in the rivermead post-concussion symptoms questionnaire (RPQ): a specific 16-item questionnaire that measures cognitive, emotional and physical symptoms. The patients symptoms during the last 24 hours are compared to before the traumatic brain injury, rating the responses of each item using a 5-point Likert scale as follows: 0 = not experienced at all; 1 = no more of a problem; 2 = a mild problem; 3 = a moderate problem; and 4 = a severe problem. Hospital anxiety (a) and depression scale (d) (had) consists of 14 items detecting states of depression (7 items) and anxiety (7 items, rated on a 4-point scale from 0 to 3:00 no symptoms, and 3 = a severe symptom or symptoms most of the time. The Glasgow Coma Scale (GCS) scores, ranging from 3 to 15, assess the level of consciousness based on eye, verbal and motor responses. Patients with a GCS score of 13–15 are classified as mild TBI, GCS score of 9–12 as moderate TBI or GCS score of 3–8 as severe TBI.
Procedures
Study design
We designed a between-group as well as a longitudinal within-subject design. Group 1 and Group 2 were examined pre–post intervention and, Group 3 was examined twice without any intervention in between. Assessment consisted of a neuropsychological test battery and neuroimaging. Semi-structured interviews pre-post were conducted in Group 1 to investigate their subjective opinion of cognitive performance and social interaction pre–post intervention.
Piano training protocol
Eight-weeks piano-tuition programme was developed and carried out by BV (Citation22) based on knowledge from a case study in which a patient with music alexia (memory loss of reading musical notation) following mTBI recovered after some month of regular piano playing. The patient, a pianist, lost the ability to read musical notation following mTBI. Two years after the accident, still unable to read music and with persistent cognitive impairment she started to denote a score (a written piece of music), by playing what she knew from memory and at the same time look at the score. During this process, she re-learned the notes and improved her cognitive performance in general. The methodology has been modified to fit the present intervention in reference to repertoire, intervention length and structured practise instructions. The curriculum focused on reinforcement of learned material as repetition is one of the factors for possible generating of new neural connections in the brain. Repertoire consisted of small tutorial pieces with progression as described in the appendix. The methodology was to apply both hands simultaneously from the start developing equal motor-movements of both hands to stimulate inter-hemispheric coordination of motor areas. Playing the piano activates the whole brain as musical elements as pitch, rhythm, melody are processed in different parts of the brain. Further, reading and playing single notes with one hand while at the same time read and play a chord with the other hand, will cause increased inter-hemispheric communication and coordination, as single notes are mainly processed in the left hemisphere, whereas chords are perceived as patterns and therefore predominantly activates the right hemisphere (Citation23). Sight-reading a score during performance adds a considerable cognitive load (Citation24). The participants received two lessons a 30 minutes per week with instructor scheduled two days apart with instructions to practise a minimum of 15 minutes each day of the week. Participants in both music groups filled in a form of daily practising time. Group 1 was instructed to report any problems in reference to physical symptoms, such as fatigue, headache, dizziness, blurred vision during practising.
Neuropsychological tests
A test battery of three different neuropsychological tests was applied during the pre–post examinations of the participants containing: Mini Mental Status Test (MMS), California Verbal Learning Test (CVLT 2), which assesses learning strategies, memorisation and retrieval of information, and Stroop Word/Colour Test for assessing reading speed and attention and ability to switch attention. Data were analysed with a 3 (group) × 2 (repetition) repeated-measure ANOVA model, using SPSS 22 (www.ibm.com).
fMRI scanning session
Functional and structural MRI scans were collected pre-post intervention for all groups. The fMRI investigation took place at the radiological department, Haukeland University Hospital, using their 3T General-Electrics Signa MR system. Besides a high-resolution T1-weighted scan, two task related fMRI sessions were included in the scanning protocol, where one task was a pitch-discrimination task and the other one was a passive listening task to Tonika-Dominant-Tonika chords, as will be explained further down.
Pitch discrimination task
The pitch discrimination was administered as block design where participants got the task to judge whether the second of two tones is higher or lower in pitch than the first one. If the tone was higher in pitch they should press their right hand button, if the pitch was lower in pitch they should press their left hand button. The paradigm was controlled by E-Prime 2.0 Professional (https://pstnet.com) that was programmed to present the stimuli and to collect the participants’ responses. During each of the five stimulation blocks 10 trials of tone-pairs were presented. Each trial started with a reference tone (440 Hz, duration 500 ms), followed by 500 ms silence, before the second tone was presented which was either higher (520 Hz, duration 500 ms) or lower (360 Hz, duration 500 ms) in pitch. The next trial started after addition 2500 ms of silence. In total, each block lasted 40 seconds. The order of the stimuli was randomised within each block. To guarantee correct timing of the stimulus presentation, the start of each block of trials was triggered by the MR-machine, using a sync-box. For technical reasons, the responses from in total 10 participants (3 controls with music, 6 controls without music, 1 patient) are not complete with missing data either from the pre- or post-session, but never from both sessions. This was caused by an undiscovered temporary malfunction of the response device. The participants listened through MR compatible headphones and were instructed to press a button as response. This is considered a low level cognitive task adapted from Zatorre (Citation25) and aims to measure low-level attention as well as, response latency. The fMRI data were acquired with an echo-planar imaging (EPI) sequence with the following parameter: TR 3s, TE 30ms, matrix size 128 × 128, 30 slices, voxel size 1.72 × 1.72 × 4.4 mm3. The block design consisted of five blocks with stimulation and five blocks of rest. Each On–Off block cycle lasted 80 seconds. In total, 140 EPI volumes were acquired.
Tonika-dominant-tonika task
A passive listening task was designed, containing extracts of classical and popular Western musical cadence pattern of Tonika-Dominant-Tonika (TDT) chords based on the first, the fifth, and the first tone of the scale. The relationships between individual tones form the basis for a fundamental aspect of musical processing and recognition of melody and there is a melodic pattern in Western music activating semantic networks that are important for long-term representation of familiar tunes (Citation25). Twenty-eight extracts were presented in an event-related design with varying delays between each stimulus. The total length of the paradigm was 08:00 minutes, and stimulus presentation was controlled by E-Prime, as described above. The length of the single stimuli varied between 2 and 19 seconds, with a variable delay between stimuli that varied between 4 and 18 seconds. In total, 160 images were acquired with the same imaging parameter as described earlier.
fMRI data analysis
Image processing and statistical analyses were performed using Statistical Parametrical Mapping (SPM12; Wellcome Department of Cognitive Neurology, London, UK) running under MATLAB (Mathworks, Inc. Natick, MA, USA). The preprocessing of the fMRI data contained first a correction of head movements and movement related distortions (realigned and unwarp). This process was followed by transforming each individual brain into a standard space, defined by a template defined by the Montreal Neurological Institute (MNI). This was achieved by warping the mean image, created in the first step, into to the standard space and applying this transformation to all other images. Finally, the data were smoothed with a Gaussian filter of 8mm FWHM (full-width at half maximum). First-level statistical analyses of the two task-related paradigms were performed by defining a general-linear model (GLM) for each individual participant and for the two tasks separately. The GLM model comprised the onsets and duration of the stimulations, as well as the realignment parameters. Contrasts were defined that tested the difference between stimulation and the rest condition.
Group statistics were performed by using the resulting contrast images from the first-level analysis. For the two paradigms separately, a 3 (group) × 2 (repetition) repeated-measure ANOVA model was defined within the GLM framework to test for averaged effects across groups and repetitions, main effect of group, main effect of repetition, and for any interactions. All results were initially explored at a family-wise error (FWE) corrected threshold of p (FWE) < 0.05. In case of significant effects, follow-up tests were performed between Group 1 and Group 2 as 2 (group) × 2 (repetition) repeated-measure ANOVAs, followed by t-tests.
Ethics
The Regional Ethics Committee of Norway (REK-Vest) approved the protocol.
Results
Semi-structured interviews – patient group
Patients were interviewed pre-post intervention to receive subjective information of the individual’s status regarding cognitive performance and social functioning. The pre-intervention questionnaire was divided into three sections. The first was questions about the actual accident, hospitalisation, and if any rehabilitation had been carried out. All patients had received rehabilitation programme within the national health service. The second section was in reference to post-concussion symptoms in which they were asked to outline problems of emotional, behavioural, cognitive and physical signs. Common problems were fatigue, blurred vision, light sensitive, dizziness, vertigo, headache, sensitive to sound. Some reported problems in finding words. The third section was questions about their present work situation. They were either sick-listed or had part time jobs with adjusted work. Post-intervention questionnaire were also divided into three sections. Firstly, how they would describe the intervention. All patients found the intervention pleasant. However, one patient did not participate in all lessons because his priorities were other activities or he forgot to meet up. This was an open question and had follow up questions about the eventually positive and negative effect they experienced during the 8 weeks intervention period. There were no report on negative issues, on the contrary, all patients had a positive experience of the intervention. Secondly, there were questions about when they noticed any improvements of the problems reported in the pre-intervention questionnaire. The average time for improved ability to concentrate and less fatigue was after four weeks of training. These answers correlated with the individual training logs where the participants were instructed to write notes of any changes in cognitive ability and social functionality. A total of 90% of the participants reported progressive improvement of both cognitive and social functioning during the remaining four weeks of intervention period. Six out of seven patients reported improved mental capacity after the intervention and better function of social interaction. The third section was about their opinion of a possible return to work or studies as before the accident. Six participants (90%) were able to return to their previous employment as before accident or studies post intervention.
Neuropsychological tests
CVLT 2 test demonstrated a highly significant effect of musical training on executive functions related to attention, learning strategies and retrieval of memories in Group 1 and Group 2. A 3 (group) × 2 (repetition) repeated-measure ANOVA was performed for the three groups on CVLT and Stroop Word/Colour test. MMS test has been excluded from the study because of a ceiling effect of all participants.
The CVLT test revealed no significant but strong trend of a main effect of group (F (2,26) = 3.15, p = 0.059), but a significant main effect of repetition (F(2,26) = 35.66, p < 0.001), and a significant group by repetition interaction (F (1,26) = 4.067, p = 0.029). Group 1 demonstrated an increase in performance up to the level of Group 2 and Group 3, where the patient’s end-point corresponds to the starting point of Group 2 and Group 3 before the intervention . Both groups receiving music intervention, Group 1 and Group 2 demonstrated a significant effect of musical training on performance, as indicated by a post hoc Fisher’s LSD-test (Group 1: p < 0.001, Group 2, p < 0.001). Group 3 has only the expected 10% increase of learning effect for pre–post testing, but the differences did not reach significance (Fisher’s LSD-test p = 0.155). There was no correlation across Group 1 and Group 2 between the improvement and the total number of hours, spent for the training (r = −0.436, p = 0.104).
Figure 1. Neuropsychological tests.
Figure displays the results from (a) CVLT and (b) Stroop tests. Error bars denote standard errors. Note that there is a highly significant improvement in the two training groups for the CVLT test (a), while the Stroop test (b) showed a significant improvement only for control groups, irrespective of training.
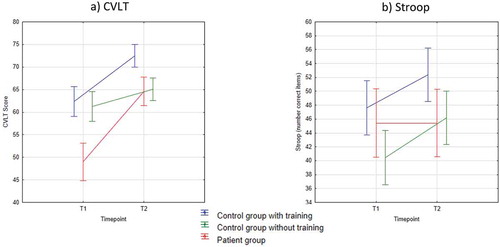
For the Stroop test, we found a slightly different picture. There was no significant main effect of groups (F(2,26) = 0.855, p = 0.437) and no group × repetition interaction (F(2,26) = 1.038, p = 0.368). However, there was a significant repetition effect (F(1,26) = 4.640, p = 0.041). Only Group 2 and Group 3 demonstrated a repetition effect, hence there was no significant effect of training . Accordingly, there were no correlations between the changes of the test scores and the total number of hours, spent for the training (r = −0.302, p = 0.273).
It should be noted that there are only 11 participants in Group 3 performing neuropsychological tests post-intervention. There was one person in this group unable to attend post testing.
Functional neuroimaging scans
Pitch discrimination task
To explore the averaged effects of the pitch discrimination task, mean contrasts across repetitions have been specified for each group and then summarised as conjunction analysis across groups. The result indicated a widespread, mostly bilateral network, comprising supplementary motor area (SMA), anterior insular, cerebellum, thalamus, putamen, Heschl’s gyrus/superior temporal gyrus, supramarginal gyrus (. ). The ANOVA results revealed neither significant main effects of group or repetition nor a significant interaction. The response data from this paradigm, which was a simple reaction time task, have also been analysed with a repeated-measure ANOVA. The result demonstrated a significant main effect of repetition (F(1,16) = 5.626 p = 0.031) and a group by repetition effect (F(2,16) = 4.247, p = 0.033), but only a strong trend towards a main effect of group (F(2,16) = 3.409, p = 0.058). These significant effects were mainly driven by an improved performance of Group 2 (Fisher’s LSD p < 0.001).
Table 2. Patient group: Scores pre-post intervention neuropsychological tests including training-time during 8 weeks intervention.
Table 3. fMRI results for the two conditions as conjunction across group means. Table describes areas of activation in terms of anatomical localisation, MNI coordinates, as well as t-statistics and cluster size with related FWE corrected p-values.
Tonika-dominant-tonika task
The conjunction across group averages revealed for the Tonika-Dominant-Tonika task that this task activated mainly the left and right auditory cortex (, ). Further, the ANOVA results revealed no main effect of group or repetition, but, more importantly, a significant group × repetition interaction in the medial orbitofrontal gyrus [MNIxyz: 10, 24, −20], peak-level: F (2,51) = 19.82, p(FWE) = 0.016, cluster-level: 2 voxel, p(FWE) = 0.028. This effect was followed up by a 2 × 2 ANOVA between Group 1 and Group 2. This analysis confirmed the previous results with the following statistical values: [MNIxyz: 10, 24, −22], peak-level: F(1,30) = 40.36, p(FWE) = 0.020, cluster-level: 7 voxel, p(FWE) = 0.014. A follow-up, directed t-test indicated that the activation in the medial orbitofrontal gyrus increased in Group 1, against a slight decrease in Group 2. It should be noted that there were 12 participants in Group 3 ().
Figure 3. Tonika-dominant-tonika task.
(a) Figure displays the results from a conjunction across group means, with a threshold of FWE-corrected threshold of p(FWE) <0.05.(b) Figure displays BOLD response and localisation of significant the group × repetition interaction effect (p(FWE) <0.05). For display purpose, the threshold is set to p < 0.001
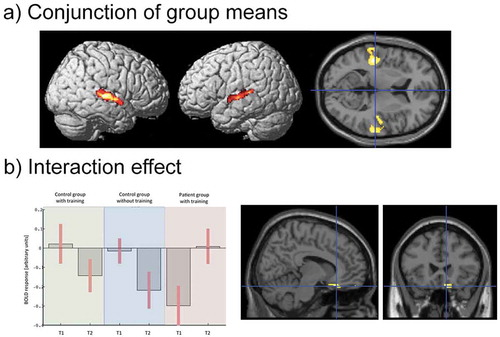
Discussion
The results strength the evidence that active training of music production influence neural activity and cognitive functioning in both patients but also healthy controls. We found distinct changes in cognitive performance but also focal changes in brain activation. Some of these effects appeared only in Group 1 and Group 2, others only in Group 2 and Group 3, and some only in Group 1. The data from neuropsychological tests pre–post music intervention demonstrated significant enhancement of cognitive performance in both Group 1 and Group 2. The results of the CVLT clearly indicated an effect of musical training on cognitive performance in Group 1 and Group 2, which was absent in Group 3 (). It is also important to note that Group 1 had a post-intervention score that was at a comparable level as the baseline of Group 2 and Group 3. Hence, the performance improved up to the normal level. Qualitative data from interviews pre–post in Group 1 supports these results.
The Stroop word/colour test result were a contradiction to the CVLT result. In the Stroop word/colour test, the Group 1 did not have any increase in score post-intervention and only Group 2 and Group 3 showed a repetition effect (). However, one has to bear in mind that these two tests measure different cognitive abilities. CVLT is sensitive in measuring functions as perception, memorisation and retrieval of information, as well as learning strategies. This test fits well with the aim of the study to enhance cognitive performance of patients with memory deficits. By contrast, the Stroop word/colour test measures tempo as reading speed, switch of attention, and inhibition, specific cognitive abilities other than those for the CVLT. Accordingly, the patient’s post-intervention score were highly significant on the CVLT but not on the Stroop word/colour test. This indicates that the intervention method promoted a specific effect on the neural networks in reference to attention, learning strategies and retrieval of information. Nevertheless, the patient group’s outcome of the Stroop test was lower than expected. Future studies should investigate the absence of a transfer effect, to disentangle the different mechanisms that cause specific changes in some cognitive functions, while performance in another cognitive function remains.
For the fMRI data, we do not find any differences in activation for the pitch discrimination task. However, we do find a significant improvement of reaction time in Group 2, reflecting a possible transfer effect of the training that may also appear in Group 1 if the training would be continued.
More importantly, we found a patient specific change in the Tonika-Dominant-Tonika task. Group 1 demonstrated a significant change of activation within the medial orbitofrontal cortex (OFC) (), which is part of the pre-frontal cortex. The OFC network regulates higher order cognitive processing, such as executive functions, including attention, decision-making, impulse control and social behaviour (Citation26). This structure has also been associated with the default mode network (Citation27). The latter notion is supported by the low to negative BOLD response under task performance in the controls. Moreover, Group 1 demonstrated a normalisation of the BOLD signal after training, from a strong deactivation before the training. A normalisation of this activation may indicate a better interplay with systems of the executive system, or the so-called extrinsic mode network (Citation27). OFC, in general, has a widely distributed interconnected neural network to almost all areas of the brain and has a wide spectrum of functions. For instance, OFC plays an important role in stimulus-reinforcement associations learning (Citation26,Citation28,Citation29), which is the ability to associate a sensory stimulus with a (positive) reinforcer. In this circumstance, auditory, visual, and motor areas are densely interconnected with other prefrontal cortical regions, reflecting integration for executive motor control, which is evident in learning to play the piano. OFC’s connectivity to association cortex is of special interest as this is one of the most important networks in developing new pathways and emotional associations (Citation10). Neural activity during music production promotes neural cross activity between music and non-music brain areas and activates association cortex by stimulating episodic and semantic memory networks (Citation10,Citation33).
Although speculative, a possible explanation for the reorganisation of neural networks during musical training may be the factor of shared neural networks for language/music (Citation30–Citation33). Association is a key word in explaining how new neural pathways may be facilitated by interaction of neural networks between musical cognition and non-musical cognitive functions as brain areas serving cognition and emotion are overlapping and interconnected. Additional information from the literature within cognitive rehabilitation provides evidence that music stimulates cognition and perception in activating neural networks engaged in attention by analysing perceptual patterns in music. Fundamental organisational processes for memory formation in music have their parallels in temporal chunking principles in non-musical memory processes (semantic and episodic memory) (Citation34). Together with Hebbian learning rules, whereby synapses are driven to change by coherent inputs in a competitive neural network (Citation9), these four interconnected factors may be the major basic elements in how music supported interventions may reorganise a broken brain system.
Conclusion
The key findings of this study are the clear evidence of a causal relationship between musical training and cognitive improvement, as well as reorganisation of neural networks. The results demonstrated significant training-related effects and enhanced cognitive performance in both music groups. These findings support the aim of the study to restore cognitive function in patients with brain injury and add a novel music-supported intervention within rehabilitation of patients with cognitive deficits following mTBI. Limitation to the study is the small number of participants in the patient group. Future research may consider the variable of intervention length and practising time, together with increased number of participants to establish an intervention adapted to future cognitive rehabilitation programmes for patients with mTBI.
Declaration of interest
The authors report no declarations of interest.
Acknowledgements
The authors thank all the participants who took time to participate in the study and the staff of the radiological department of the Haukeland University Hospital (Bergen, Norway) for their help during data acquisition. The study was supported by a grant to KS from the Research Council of Norway (217932: It’s time for some music).
References
- Herholz SC, Coffey EBJ, Pantev C, Zatorre R. Dissociation of neural networks for predisposition and for training-related plasticity in auditory-motor learning. Cerebral Cortex. 2015;1–10. doi: 10.1093/cercor/bhv138.
- Thaut MH. Therapy in Cognitive Rehabilitation. Music Perception. 2010;27(4):281–85.
- Hegde S. Music-based cognitive remediation therapy for patients with traumatic brain injury. Frontiers in Neurology. 2014;5. doi: 10.3389/fneur.2014.00034.
- Jäncke L. Music drives brain plasticity. Floor Reports Biology. 2009. doi: 10.3410/B1-78.
- Stewart L, Walsh V. Music of the hemispheres. Current Biology. 2001;11(4):R125–R27.
- Schlaug G. Music, musicians, and brain plasticity. In: Hallam S, Cross I, Thaut MH, editors. The oxford handbook of music psychology. New York: Oxford University Press; 2009. p. 197–207.
- Särkamö T, Tervaniemi M, Huotilainen M Music perception and cognition: development, neural basis,and rehabilitative use of Music. WIREs CognSci. 2013. doi: 10.1002/wcs.1237.
- Altenmüller E. Music as a driver for brain plasticity. Clinical Neurophysiology. 2016;127:e22–e22. doi: 10.1016/j.clinph.2015.11.062.
- Hebb D. Distinctive features of learning in the higher animal. In: Delafresnaye JF, editor. Brain Mechanisms and Learning. London: Oxford University Press; 1961.
- Schlaug G. Music, musicians, and brain plasticity. In: Hallam S Cross I, Thaut MH, editors. The oxford handbook of music psychology. New York: Oxford University Press; 2009.
- Jäncke L. Music drives brain plasticity. Floor Reports Biology. 2009; Rep:2009:1–78 doi: 10.3410/B1-78.
- Särkamö T, Ripolles P, Vepsäläinen H, Autii T, Silvennoinen HM, Salli E, Laitinen S, Forsblom A, Soinila S, Rodriguez-Fornells A. Structural changes induced by daily music listening in the recovering brain after middle cerebral artery stroke; a voxel-based morphometry study. Frontiers in Human Neuroscience. 2014; doi: 10.3389/fnhum.2014.00245.
- Andelic N, Hammergren N, Bautz-Holter SU, Brunborg C, Røe C. Functional outcome and healthrelated quality of life 10 years after moderate-to severe traumatic brain injury. Acta Neurologica Scandinavia. 2009;120:16–23.
- Teasdale G, Jennett B. Assessment of coma and impaired conscioiusness. A practical scale. Lancet. 1974;2:81–84.
- Carroll LJ, Cassidy JD, Holm L, Kraus J, Coronado V. Methodological issues and research recommendations for mild traumatic brain injury: the WHO collaborating centre task force on mild traumatic brain injury. Journal of Rehabilitation Medicine. 2004;36:113–25.
- Levine B, Fujiwara E, O’Connor C, Richard N, Kovacevic N, Mandic M, Restagno A, Easdon C, Robertson IH, Graham SJ, et al. In vivo characterization of traumatic brain injury neuropathology with structural and functional neuroimaging. Journal of Neurotrauma. 2006;23:1396–411.
- Voller B, Auff E, Schnider P, Aicher F. To do or not to do? Magnetic resonance imaging in mild traumatic brain injury. Brain Injury. 2001;15:107–15.
- Schiavio A, Exploring Music-Based AE. Rehabilitation forParkinsonism through embodied cognitive science. Front Neurol. 2015;19:6–217.
- Pantev C. Auditory-somatosensory integration and cortical plasticity in musical training. In: Dalla Bella S, Kraus J, Overy K, Pantev C, Snyder SJ, Tervaniemi M, Tillmann B, Schlaug G, editors. The Neurosciences and Music III Disorders and Plasticity. Boston: The New York Academy of Sciences; 2009.
- Rojo N, Amengual J, Juncadella M, Rubio F, Camara E, Marco-Pallares J, et al. Music-supported Therapy induces plasticity in the sensorimotor cortex in chronic stroke: a single case study using multimodal imaging (fMRI-TMS). Brain Injury. 2011; 25(7–8):787–93.
- Dalla Bella S, Benoit CE, Farrugia N, Schwartz ML, Kotz S. Effects of musically cued gait-training in Parkinsons’s disease: beyond a motor benefit: auditory cueing in Parkinson’s disease. Ann NY Acad Sci. 2015;1337(1):77–85.
- Vik,B Musical alexia with recovery - a personal report. Unpublished paper presented at Comphrehensive Rehabilitation and 4th Congress of the Baltic Spinal Chord Society. Vilnius, Lithuania; 2006.
- Gordon HW. Music and the Right Hemisphere. In: Young A, editor. Functions of the Right Cerebral Hemisphere. London: Academic Press; 1983.
- Parsons L, Sergent J, Hodges DA, Fox PT. The brain basis of Piano performance. Neuro-Psychologia. 2005;43:199–215.
- Zatorre R. Neural Specialiation for Tonal Processing. In: Perez I, Zatorre R, editors. The Cognitive Neuroscience of Music. Oxford: Oxford University Press; 2007. p. 232 and 237.
- Rolls E. Emotion and decision-making explained. Cortex. 2014;59:185–93.
- Hugdahl K, Raichle ME, Mitra A, Specht K. On the existence of a generalized non-specific task-dependent network. Front Hum Neurosci. 2015;9:1–15.
- Rolls. E. The functions of the orbitofrontal cortex. Brain and Cognition. 2004;55:11–29.
- Rolls E, Grabenhorst F. The orbifrontal cortex and beyond: from affect to decision-making. Progress in Neurobiology. 2008;86:216–44.
- Chan AS, Ho YC, Cheung MC. Music training improves verbal memory. Nature. 1998;396(6707):128.
- Brown S, Martinez M, Parsons L. Music and language side by side in the brain: a PET study of the generation of melodies and sentences. European Journal of Neuroscience. 2006;23(10):2791–803.
- Patel A, Peretz I, Tramo M, Labreque R. Processing prosodic and musical patterns: a neuropsychological investigation. Brain and Language. 1998;61(1):123–44.
- Parsons L, Himonides E, Craig N, Vakil M, Papadakis N, Turner R, Wilkinson E. Simultanous dual fMRI scanning of duetters. Edinburgh, Scotland: British Research Association Meeting; 2008.
- Deutsch, D. Grouping Mechanisms in Music. In: Deutsch D, editor. The Psychology of Music. New York: Academic Press; 1982.
Appendix
Piano training protocol – 8 weeks.
OBJECTIVES TEACHING MATERIAL: Tuition book: Agnestig,C.B. AB Carl Gehrmans Musikforlag, Stockholm, Sweden.