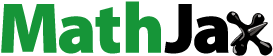
ABSTRACT
Objective
Disrupted sleep is common following combat deployment. Contributors to risk include posttraumatic stress disorder (PTSD) and mild traumatic brain injury (mTBI); however, the mechanisms linking PTSD, mTBI, and sleep are unclear. Both PTSD and mTBI affect frontolimbic white matter tracts, such as the uncinate fasciculus. The current study examined the relationship between PTSD symptom presentation, lateralized uncinate fasciculus integrity, and sleep quality.
Method
Participants include 42 combat veterans with and without PTSD and mTBI. Freesurfer and Tracula were used to establish specific white matter ROI integrity via 3-T MRI. The Pittsburgh Sleep Quality Index and PTSD Checklist were used to assess sleep quality and PTSD symptoms.
Results
Decreased fractional anisotropy in the right uncinate fasciculus (β = −1.11, SE = 0.47, p < .05) and increased hyperarousal symptom severity (β = 3.50, SE = 0.86, p < .001) were associated with poorer sleep quality.
Conclusion
Both right uncinate integrity and hyperarousal symptom severity are associated withsleep quality in combat veterans. The right uncinate is a key regulator of limbic behavior and sympathetic nervous system reactivity, a core component of hyperarousal. Damage to this pathway may be one mechanism by which mTBI and/or PTSD could create vulnerability for sleep problems following combat deployment.
Introduction
Sleep disturbances are among the most commonly endorsed symptoms in military personnel and veterans. In one study of Vietnam War veterans, 94% of the sample (n = 152) reported sleep problems at a clinical level (Citation1). In a larger sample of military personnel from all branches and components of the service (n = 41,225), the Millennium Cohort Study (Citation2) found that 25% to 30.5% of military personnel report trouble sleeping (Citation3). Notably, those who deployed to a theater of combat operations were 52% to 74% more likely to report sleep problems when compared to those without combat deployment. This finding is consistent with a substantial body of research demonstrating a positive correlation between combat exposure and risk for sleep problems (Citation4–9).
Combat deployment may increase the risk for sleep problems through a number of pathways. For instance, during deployment to a theater of combat, service members are routinely exposed to a variety of harmful and potentially traumatic experiences (Citation10). As a result, they maintain a state of heightened arousal, which is associated with overactivation of the noradrenergic system (Citation11). Noradrenaline is closely involved in the regulation of the sleep-wake cycle, and increased activity of this system could contribute to fragmentation of sleep (Citation12,Citation13). In support of this potential pathway, hyperarousal symptoms have been specifically linked to greater sleep disturbance in individuals with posttraumatic stress disorder (PTSD) (Citation14). Furthermore, combat deployment has been associated with increased risk for developing PTSD, which commonly involves sleep disruption (Citation15–17).
Another factor that could increase risk for sleep disturbance and modify the presentation of PTSD among previously combat deployed veterans may be sustaining a mild traumatic brain injury (mTBI). VA and DOD diagnostic guidelines define a mTBI as a traumatically induced structural injury and/or physiological disruption of brain function resulting from an external force that is characterized by loss of consciousness (LOC) for less than 30 minutes, alteration of consciousness (AOC) for less than one day, neurological deficits (i.e., dizziness, loss of balance), and/or posttraumatic amnesia for less than one day (Citation18). mTBI is common across all veterans; however, those with high combat exposure have nearly three times greater odds of meeting criteria for a TBI during service (Citation19). mTBI is in turn associated with a variety of sleep problems, including insomnia, hypersomnia, obstructive sleep apnea, and circadian rhythm abnormalities (Citation20–23). mTBI may also indirectly lead to sleep problems through increasing risk for PTSD or modifying its presentation (Citation24–26).
While the mechanisms linking mTBI, PTSD, and sleep disturbances in combat veterans are largely unknown, sleep disturbances may be related to alterations in white matter microstructure (Citation27). The most common form of primary damage from mTBI is diffuse axonal injury (DAI), which refers to the stretching and tearing of axons that results from rapid acceleration/deceleration of the brain. DAI can damage the axonal cytoskeleton, which impairs axonal transportation and in turn disrupts communication between different brain structures (Citation28). While white matter damage from mTBI is heterogenous, neuroimaging studies and controlled experimental studies with rats have shown that frontolimbic pathways may be particularly vulnerable to damage in mTBI (Citation29–32). Similarly, PTSD in the absence of mTBI has also been shown to affect white matter microstructure, particularly in frontolimbic tracts (Citation33–39). The mechanism for white matter alterations in PTSD may be related to increases in glucocorticoid levels, which can be neurotoxic and affect myelination (Citation40–42). One frontolimbic tract that has been shown to be affected by both PTSD and mTBI is the uncinate fasciculus (Citation31,Citation36). In fact, Williamson et al. have proposed that damage to the uncinate fasciculus may be one mechanism linking mTBI and PTSD and that lateralized injury to the right hemisphere may be a contributor (Citation43).
The uncinate fasciculus is a critical component of the ventrolateral limbic network, which links the amygdala with the orbitofrontal cortex via the anterior temporal pole. This tract plays a key role in prefrontal inhibition of mesial temporal lobe structures, such as the amygdala, that are involved in autonomic nervous system (ANS) regulation (Citation44–46). Therefore, damage to this pathway may induce a loss of inhibitory control over the ANS, which could lead to a variety of sleep problems due to the close relationship between the ANS and sleep/wake regulation (Citation47–49). Furthermore, there is a lateralized differentiation in the control of the ANS, such that the right hemisphere shows dominance in sympathetic nervous system regulation (Citation50–52). Thus, damage to the right uncinate in particular may impact sleep.
Contributors to risk for sleep disturbance are important to consider, as sleep problems in PTSD have wide-ranging adverse effects on health and cognition (Citation53,Citation54). Previous studies investigating predictors of sleep disturbance in PTSD have not specifically examined alterations in white matter microstructure. Several have demonstrated that mTBI is associated with increased incidence and severity of sleep disturbance (Citation55–57). However, they have not employed neuroimaging methods to determine the neurological mechanisms by which mTBI may be impacting sleep. Similarly, many studies have shown that greater daytime PTSD symptom severity is linked to sleep problems (Citation8,Citation58,Citation59). However, they have not examined the contribution of uncinate fasciculus integrity, which is associated with PTSD symptom severity (Citation39,Citation60). Moreover, PTSD symptom expression is heterogeneous, and few studies have examined the relationship between relative expression of different PTSD symptom clusters and sleep disruption. One study showed that prominent expression of hyperarousal symptoms predicts sleep quality (Citation14); however, the study dichotomized hyperarousal symptom severity and did not examine the relative impact of other PTSD symptom clusters. Furthermore, the results have not yet been replicated in a veteran population.
The present study aimed to investigate the relationship between PTSD symptom presentation, uncinate fasciculus integrity, and self-reported sleep quality in a sample of veterans with and without PTSD and mTBI who had previously deployed on active duty to a theater of combat operations. We hypothesized that greater expression of hyperarousal symptoms and lower white matter integrity in the uncinate fasciculus would be associated with poorer sleep quality due to aANS dysregulation. Furthermore, we hypothesized that right hemispheric uncinate fasciculus structural integrity would have a stronger relationship with sleep quality than left hemispheric integrity due to the lateralized differentiation in the control of the ANS and the experience of negative emotions.
Materials and methods
Participants and procedure
Forty-two veterans (37 men, 5 women) who were previously deployed on active duty to a theater of combat operations were recruited from the North Florida/South Georgia Department of Veterans Affairs Medical Center and surrounding community. A structured interview designed for this study and self-report questionnaires were used to gather demographic data, medical and psychiatric history, mTBI history, and PTSD symptomatology. The structured interview assessed for mTBI according to the VA and DOD diagnostic guidelines, and it was supplemented by the Ohio State University TBI Identification Method Short Form as well as VA and DOD medical record review (Citation61). With regard to PTSD history, participants were asked about their experience with PTSD symptoms as outlined in the Diagnostic and Statistical Manual 4th edition (DSM-IV), including the timing and nature of symptom onset (Citation62). Participants were asked about Criterion A event exposure, including traumatic events prior to, during, and after their military service. While not all participants experienced Criterion A event exposure, those who did experience the event during military service. In the structured interview, participants were also asked if they have a history of any psychiatric conditions (e.g., depression, bipolar disorder, schizophrenia) prior to TBI or PTSD onset. If they responded yes, they were asked to verbally provide information regarding the type of psychiatric condition, when the first symptoms began, and when the condition was diagnosed. VA and DOD medical records were reviewed to corroborate participants’ self-report, when available. Following completion of the structured interview, participants completed self-report scales including the PTSD Checklist-Military version (PCL-M) (Citation63) and the Pittsburgh Sleep Quality Index (PSQI) (Citation64). A diagnostic consensus conference was then used to verify participant diagnoses according to VA and DOD diagnostic guidelines.
Exclusion criteria for the study included: neurological disorders aside from TBI, any disorder that might influence measures of the autonomic nervous system (e.g., history of heart attack, atrial fibrillation, diabetes), history of severe psychiatric illness that cannot be reasonably attributed to a trauma reaction/comorbidity (e.g., schizophrenia, bipolar disorder), previous (to trauma) sleep disorder, recent substance abuse (assessed via self-report and record review; no marijuana or alcohol abuse within two weeks of the study, and no drugs such as cocaine or heroin within at least two months), pregnancy, current prescription for medications that influence autonomic activity (e.g., beta-blockers, ace inhibitors), and any contraindications to MRI scanning. Two participants were excluded from statistical analyses because they did not undergo MRI scanning. All study procedures were approved by the University of Florida Institutional Review Board. Participants provided written informed consent and were compensated for their time and travel.
Neuroimaging
Imaging was conducted at a scanning facility located at the University of Florida using a 3-T Philips Achieva MRI scanner. T1-weighted, T2-weighted, 3D fluid attenuated inversion recovery (FLAIR), T2*-weighted echo planar, and high angular resolution diffusion imaging (HARDI) sequences were acquired. The diffusion gradients were applied along six directions with a b-value of 100 s/mm2 and along 64 noncollinear directions using a b-value of 1000 s/mm2, and one image was acquired with diffusion weighting (b= 0).
Measures
PTSD symptoms were assessed using the PCL-M (Citation63), a well-validated and commonly used self-report measure of PTSD symptoms (Citation65). The PCL-M consists of 17 items which correspond to the DSM-IV criteria for PTSD (Citation62). For each item, participants rate the degree to which they were bothered by a particular symptom in the past month using a 5-point Likert scale that ranges from 1 (“not at all”) to 5 (“extremely”). Subscores were calculated for different DSM-IV PTSD symptom clusters by aggregating items on the PCL-M relating to reexperiencing (items 1–5), avoidance (items 6–12), and hyperarousal (items 13–17) (Citation66). The average score across participants was 10.24 for reexperiencing (SD = 4.94), 13.40 for avoidance (SD = 6.81), and 12.79 for hyperarousal (SD = 5.57) (see ). The PTSD Checklist in general has demonstrated high levels of diagnostic accuracy when compared to gold standard measures of PTSD symptomatology, such as the clinician-administered PTSD scale (CAPS) (Citation67). The PCL-M has also demonstrated excellent internal consistency, with a Cronbach’s alpha in the present sample of 0.97.
Table 1. Participant demographics and data from self-report inventories
Sleep quality was assessed with the Pittsburgh Sleep Quality Index (PSQI) (Citation64). This 19-item questionnaire yields component scores for seven different domains of sleep quality: subjective sleep quality, sleep latency, sleep duration, habitual sleep efficiency, sleep disturbances, use of sleep medication, and daytime dysfunction. These component scores were then summed to yield a global score, which ranges from 0 to 21 with greater scores indicating poorer sleep quality. The mean global score in the current sample was 9.43 (SD = 4.89). This measure is widely used in both clinical and research settings, and it has demonstrated strong reliability and validity (Citation68). Cronbach’s alpha in the present sample was 0.84.
Data Analysis
Anatomical reconstruction was performed on each participant’s T1-weighted image using FreeSurfer software to obtain a cortical parcellation and subcortical segmentation for each participant (Citation69). Further, diffusion tractography was performed using FreeSurfer’s TRACULA (TRActs Constrained by UnderLying Anatomy) tool, which provides an automated probabilistic reconstruction of white matter pathways from each participant’s DWI data (Citation70). During preprocessing, data were corrected for head motion-related artifacts and image distortion due to eddy currents using eddy_correct in the FSL toolbox (https://fsl.fmrib.ox.ac.uk/fsl/fslwiki). In addition, data were visually inspected before and after processing and no apparent issues with motion-related artifacts were identified. The vectors of the b-value were rotated using fdt_rotate_bvecs after eddy correction. The DTI parameter fractional anisotropy (FA) was calculated using dtifit tools in FSL. The average FA for the center (i.e., the single-voxel wide path with the highest probability, along the entire tract) of the forceps major and left and right uncinate fasciculus (see ) was used for statistical analysis (Citation70). FA values for the center of each tract were selected to avoid nonwhite matter tissue partial volume effects (Citation71,Citation72). In addition, an aggregate measure of global white matter microstructure excluding the left and right uncinate was calculated by summing the average central FA weighted by volume for all other measured white matter tracts. The tracts included in this global white matter aggregate are the forceps major, forceps minor, left and right anterior thalamic radiation, left and right cingulum angular bundle, left and right cingulum cingulate gyrus, left and right inferior longitudinal fasciculus, left and right superior longitudinal fasciculus parietal, and left and right superior longitudinal fasciculus temporal.
Figure 1. A: Axial view of the right and left uncinate fasciculus in a representative participant. B: Oblique view of the right uncinate fasciculus in a representative participant. The estimated distribution of each white matter pathway is displayed as an isosurface, thresholded at 20% of its maximum, overlaid on the participant’s fractional anisotropy (FA) map
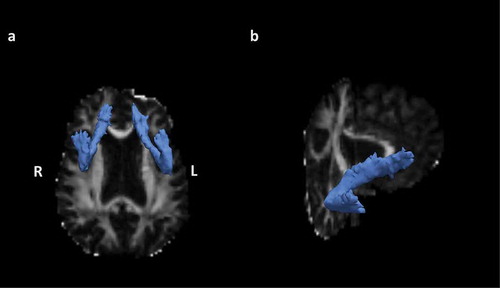
We entered the three PTSD symptom cluster subscores (reexperiencing, avoidance, and hyperarousal), left and right uncinate fasciculus FA, forceps major FA, the global white matter aggregate, years since combat, and age into a Least Absolute Shrinkage and Selection Operator (LASSO) regression model to determine the best fit model for predicting sleep quality. The forceps major was included as a non-frontolimbic white matter tract to contrast with the uncinate, and the global white matter aggregate was included to determine if global white matter integrity or focal frontolimbic integrity was most closely associated with sleep quality. Age and years since combat were included as covariates due to their known relationship with white matter metrics (Citation73,Citation74). The LASSO (Citation75) employs L1-norm regularization, using a compound cost function to optimize the regression coefficients:
In this function, y represents the vector of sleep quality as measured by PSQI score, X is the matrix of standardized candidate predictor variables, and β* represents the vector of fitted regression coefficients for each candidate predictor’s effect on sleep quality. λ is a parameter for controlling the strength of L1-norm regularization. K-fold cross validation was performed using 10 folds to determine the optimal lambda value, which was defined as the one which produced the smallest average mean squared error across folds. For these analyses, we used the “glmnet” package (Citation76) implemented in R, which uses a coordinate descent algorithm to optimize model fitting parameters. We then fitted our LASSO model with the optimal parameters and obtained coefficients for each candidate predictor. The LASSO performs variable selection by setting the coefficient to zero for variables that were not selected in the best fit model. A multiple linear regression was then run using the variables selected by the LASSO as predictors and PSQI score as the dependent variable.
Exploratory analyses
Primary statistical analyses were conducted across all participants regardless of PTSD or mTBI diagnosis to capture the full range of PTSD symptom severity and white matter injury that combat veterans may experience. To supplement these analyses, exploratory between-group comparisons of right uncinate integrity and sleep quality were conducted using independent samples t-tests in participants with and without mTBI history. It is important to note that the present study was cross-sectional and did not include predeployment neuroimaging, so we are unable to determine the cause of any observed variability in white matter integrity. White matter differences may be preexisting or the result of PTSD, TBI, or an unknown factor.
Results
Within our sample of previously combat deployed veterans, there was a broad range of PTSD symptoms and mTBI history: 12 participants met diagnostic criteria for both PTSD and mTBI, 8 for PTSD only, 7 for mTBI only, and 15 for neither. In terms of demographics, participants varied from 23 to 45 years in age (M = 32.86 years, SD = 6.65). They had an average of 14.36 years of education (SD = 1.81), and the majority were right-handed (n= 35). Length of time since most recent combat tour varied from 1 year to 13 years (M = 5.64, SD = 3.32) (see ).
Cross validation determined an optimal lambda value of 0.41 for our LASSO model. Using this lambda value, the LASSO regression indicated that a three-predictor model was the best fit for our data, with right uncinate fasciculus FA, hyperarousal, and avoidance all yielding nonzero coefficients (see ). FA in the left uncinate fasciculus, forceps major FA, the global white matter aggregate, reexperiencing symptoms, years since combat, and age were not selected by the model, as indicated by their resulting coefficient of zero. Using the selected predictors, we then checked that our data met the assumptions of linearity, multivariate normality, and homoscedasticity for a regression model using the “gvlma” package in R (Citation77). We also ran diagnostic tests to ensure that it met the assumption of no multicollinearity (FA in the right uncinate fasciculus, Tolerance = 1.00, VIF = 1.00; Hyperarousal, Tolerance = 0.30, VIF = 3.36; Avoidance, Tolerance = 0.30, VIF = 3.37). The multiple linear regression model using the selected predictors explained significant variance in PSQI score (adjusted R-squared = 0.6395, F (Citation2,Citation35) = 24.06, p < .001). FA in the right uncinate was negatively and significantly associated with PSQI score, such that a 1 standard deviation (SD) unit decrease in FA was associated with a 1.11 unit increase in PSQI score, indicating a decrement in sleep quality (β = −1.11, SE = 0.47, p < .05). Hyperarousal was positively and significantly associated with PSQI score, such that a 1 SD unit increase in hyperarousal symptoms was associated with a 3.50 unit increase in PSQI score, indicating a decrement in sleep quality (β = 3.50, SE = 0.86, p < .001). Avoidance was positively associated with PSQI score; however, this association was nonsignificant (β = 0.40, SE = 0.86, p = .65) (see ; see also Supplemental for visualization of results excluding participants with history of mTBI). The final equation for the standardized regression model was: PSQI score = −1.11 ± 0.47 (FA in the right uncinate fasciculus) + 3.50 ± 0.86 (Hyperarousal subscore) + 0.40 ± 0.86 (Avoidance subscore). Results are presented in .
Table 2. Multiple linear regression results with right uncinate fasciculus (Unc.) FA, Hyperarousal, and Avoidance PCL-M scores predicting PSQI score. Variables reported were selected by the LASSO model as important predictors of sleep quality
Figure 3. Top left: Semi partial correlation between right uncinate fasciculus (unc.) FA and PSQI score adjusted for hyperarousal and avoidance. Top right: Semi partial correlation between hyperarousal subscore and PSQI score adjusted for right unc. FA and avoidance. Bottom right: Semi partial correlation between avoidance subscore and PSQI score adjusted for right unc. FA and hyperarousal. The 95% confidence interval of the regression line is shown in gray
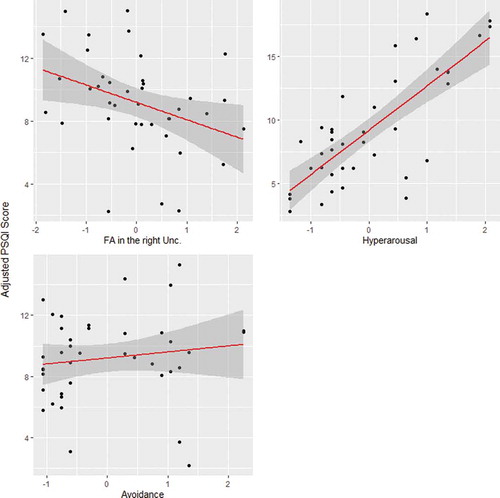
Given that PTSD symptoms are known to affect sleep quality (Citation8,Citation58,Citation59), we also conducted a hierarchical linear regression to further quantify the contribution of right uncinate integrity to sleep quality beyond PTSD symptoms. In the first step, we entered only hyperarousal and avoidance symptoms as predictors of PSQI score. This model explained 59.53% of the variance in sleep quality (adjusted R-squared = 0.5953, F (Citation1,Citation36) = 29.69, p < .001). Then, in the second step, we added right uncinate fasciculus white matter integrity as a predictor. As described above, this model explained 63.95% of the variance in sleep quality (adjusted R-squared = 0.6395, F (Citation2,Citation35) = 24.06, p < .001). Thus, adding right uncinate fasciculus integrity explained an additional 4.42% of the variance in PSQI scores, which was determined to be significant by an analysis of variance comparing the two models (p < .05). The AIC value also decreased from 243.37 in the first step to 205.54 in the final model.
Exploratory analyses
In order to explore the relationship between mTBI history and frontolimbic white matter integrity within our sample, an independent samples t-test was conducted comparing FA in the right uncinate in participants with a history of at least one mTBI (n = 18) versus those without a history of mTBI (n = 22). While there was a trend of small-to-moderate effect size that those with a history of mTBI (M = 0.38, SD = 0.05) on average had lower right uncinate integrity than those without a history of mTBI (M = 0.41, SD = 0.07), the difference between groups was not significant (t(38) = 1.52, p = .14, Cohen’s d = 0.48) (see ).
Figure 4.. Boxplot of right uncinate fasciculus (unc.) FA in participants with (right) and without (left) a history of mTBI. Lower and upper box boundaries represent the 25th and 75th percentiles respectively, and the horizontal line within the box represents the median value
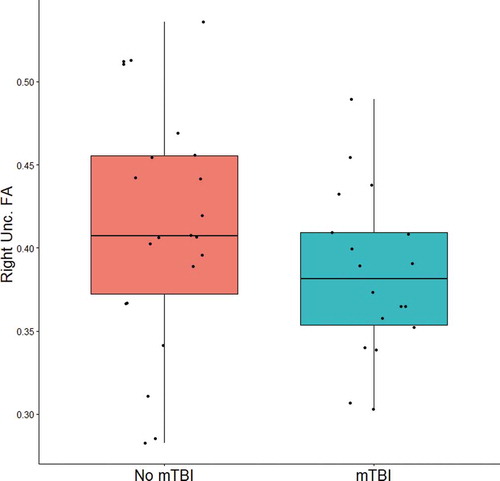
In order to explore the relationship between mTBI history and sleep quality within our sample, an independent samples t-test was conducted comparing PSQI scores in participants with a history of at least one mTBI (n = 7) versus those without a history of mTBI (n = 15). Participants that were determined to meet criteria for PTSD in the diagnostic consensus conference were excluded from this analysis to avoid confounding the relationship between mTBI and sleep with PTSD. While there was a trend of moderate effect size that those with a history of mTBI without PTSD (M = 8.00, SD = 3.65) on average had higher PSQI scores than those without a history of mTBI or PTSD (M = 6.33, SD = 2.92), the difference between groups was not significant (t(20) = 1.15, p = .26, Cohen’s d = 0.53) (see ).
Discussion
The primary findings among this combat veteran cohort were that both right hemispheric uncinate fasciculus structural integrity and hyperarousal symptom severity were significantly associated with self-reported sleep quality. Right hemispheric uncinate fasciculus integrity was negatively associated with PSQI score, such that individuals with lower FA values in the right uncinate fasciculus reported greater sleep problems. Hyperarousal symptoms were positively associated with PSQI score, such that individuals who reported greater levels of hyperarousal symptoms also reported having greater sleep problems. Other types of PTSD symptomatology, such as reexperiencing and avoidance, were not significantly associated with sleep quality. Furthermore, the relationship between white matter integrity and sleep was focal to the uncinate fasciculus and lateralized, such that FA values in the right uncinate fasciculus were associated with sleep quality while FA values in the left uncinate fasciculus were not. Overall, these results may help to identify a subphenotype of combat-exposed veterans who are at greater risk for sleep issues and may suggest a potential mechanistic pathway by which mTBI or traumatic stress may increase risk for sleep problems following combat exposure.
Our finding regarding the relationship between right uncinate fasciculus integrity and self-reported sleep quality is in line with previous work demonstrating a negative correlation between white matter integrity and sleep quality in individuals with mTBI (Citation78), insomnia (Citation79,Citation80), and community-dwelling older adults (Citation81). Notably, we found that the effect of white matter integrity on sleep quality in our sample of combat veterans was focal to the uncinate fasciculus, as opposed to more generalized to global white matter integrity or integrity in non-frontolimbic tracts, such as the forceps major. Damage to the uncinate fasciculus may affect sleep by reducing inhibitory control over the ANS, which is closely involved in sleep/wake regulation (Citation47–49). Consistent with this hypothesis, previous work using a sleep deprivation paradigm has linked sleep quality to frontolimbic connectivity (Citation82). Given that we do not have predeployment data in this study, it is not possible to determine whether lower integrity in the right uncinate was a preexisting factor or the result of mTBI, PTSD, or other unknown factors. In our between-group analysis of right uncinate integrity, though limited by sample size, we saw a small-to-moderate effect size trend for lower right uncinate integrity in those with a history of mTBI with or without PTSD. This finding is in line with numerous previous studies that have found an association between mTBI and uncinate fasciculus integrity (Citation83–85), as well as PTSD and uncinate fasciculus integrity (Citation36,Citation39). Overall, our findings demonstrate that, when controlling for hyperarousal levels, damage to frontolimbic white matter tracts is associated with an increased level of sleep problems. Importantly, these findings may represent one mechanistic pathway by which mTBI and/or PTSD, both of which have been shown to impact white matter integrity in previous studies, could create vulnerability for sleep disturbances following combat deployment.
Another important finding from this study was that the effect of uncinate fasciculus integrity on sleep quality was lateralized, such that integrity in the right hemisphere affected sleep quality while integrity in the left hemisphere did not. This finding is consistent with previous work demonstrating reduced integrity of right lateralized white matter in patients with insomnia (Citation79). There is some evidence that the right hemisphere preferentially mediates both negative emotional experience and mobilization of the sympathetic nervous system (Citation51,Citation86–90). Thus, damage to right hemispheric white matter tracts could have a greater impact on sympathetic nervous system activity, influencing arousal and the ability to fall or stay asleep, as well as on the expression of negative emotions such as fear. This finding regarding laterality has important implications particularly in veterans who have suffered a mTBI, as the hemispheric location of damage could differentially impact sleep quality.
In addition to demonstrating effects of frontolimbic integrity on sleep quality, our findings also highlight the known relationship between hyperarousal symptoms and sleep quality, lending further support to van Wyk and colleagues’ hypothesis that the presence and degree of hyperarousal symptoms may explain why some individuals with PTSD experience marked sleep disturbance while others do not (Citation14). Mechanistically, the relationship between hyperarousal symptoms and sleep quality may be related to dysregulation of the sympathetic nervous system and norepinephrine (NE) levels (Citation11–13). Typically, noradrenergic neurons of the locus coeruleus (LC), the primary source of NE to the forebrain, fire maximally during wakeful states, decrease their activity throughout the sleep stages, and are silent during REM sleep (Citation91,Citation92). However, individuals with PTSD often have elevated levels of NE that do not diminish throughout the night (Citation93,Citation94). This elevation in NE levels is associated with hyperarousal symptoms and could ultimately impair sleep quality, given the role of NE in regulating the sleep-wake cycle (Citation12). Another possible explanation for the relationship between hyperarousal and sleep quality could relate to disinhibition of limbic structures. Hyperarousal symptoms in PTSD can be conceptualized as the manifestation of a bias toward a defensive neurophysiological state (Citation43,Citation95). In order for an individual to shift from this defensive state and into one conducive to sleep (i.e., where the individual determines the environment to be safe), they must inhibit limbic structures, such as the amygdala, which control fight, flight, or freezing behaviors. Different factors related to combat exposure, such as traumatic stress or mTBI, may negatively affect inhibition of these structures, causing them to be chronically disinhibited. Ultimately, this disinhibition could be related to both hyperarousal symptoms as well as sleep problems.
One strength of our study is its focus on sleep quality, which is a potentially modifiable risk factor for poor clinical outcomes among combat veterans. In addition, we used continuous measures of dimensional constructs such as PTSD symptom severity, sleep quality, and white matter integrity, rather than relying on categorical diagnoses. We also included participants with varying levels of PTSD symptomatology, sleep quality, and mTBI history, allowing us to capture the full range of symptoms that combat veterans may experience on these axes and improve the generalizability of our results.
Limitations to our study include the cross-sectional design, which prevented us from being able to look at causal relationships among our variables, such as whether a difference in white matter integrity was a predeployment vulnerability factor or the result of mTBI or chronic PTSD. A prospective study with pre- and post-deployment neuroimaging would be necessary to further investigate causal relationships between white matter integrity and sleep quality. Though, it is worthy to note that both animal models and human studies have previously demonstrated vulnerability of frontolimbic connectivity in TBI and PTSD (Citation29,Citation31,Citation34,Citation36). We also measured hyperarousal symptoms and sleep quality using self-report, but in the future work, it would be valuable to examine these constructs objectively, using physiological measures and a sleep study design. While the PCL-M has demonstrated high levels of diagnostic accuracy (Citation67), an additional limitation is that we did not use the gold standard CAPS interview to assess for PTSD symptomatology due to time constraints, and the PCL-M includes one item about sleep in its hyperarousal subscore. In addition, while it was valuable to conduct the primary analyses across all participants regardless of mTBI or PTSD diagnosis in the current investigation, future studies should also examine differences across all four diagnostic subgroups (controls, PTSD, mTBI, PTSD+mTBI) to further parse the effects of PTSD and mTBI on frontolimbic integrity and sleep quality. Given the sample size of the current study, we did not have the statistical power to conduct this type of analysis, although we did report exploratory analyses based on mTBI history alone. We also did not have the power to run a moderator analysis as a function of mTBI history, which would be valuable to do in future studies. Finally, it is also important to acknowledge limitations to our acquisition and analysis of DTI data. This study utilized a b-value of 1000 s/mm2, and higher b-values in future studies may improve accuracy (Citation96). In addition, we used eddy_correct in our preprocessing pipeline, and an updated version of this tool (eddy) has since been released which provides improved correction for eddy current-induced distortions and subject movements (Citation97). Aside from the motion correction inherent to eddy_correct and our visual inspection of movement artifact, we did not quantitatively evaluate motion confounds (Citation98). Moreover, we chose to use central FA in our analyses to minimize non-white matter tissue partial volume effects; however, this approach may also have disadvantages in reducing capture of the full white matter path (Citation99). Lastly, it is worth noting that all DTI models using FA may incorrectly represent complex fiber configurations (e.g., crossing fibers) present in white matter (Citation100,Citation101).
Overall, this study builds upon previous work to further our understanding of sleep problems in combat veterans. While hyperarousal has been previously linked to sleep problems in individuals with PTSD, our study also examines the contribution of frontolimbic white matter integrity, which is critical to consider in combat veteran populations, given that conditions affecting white matter integrity, such as PTSD and mTBI, are common. Our findings help to identify a subgroup of individuals who may be at greater risk for sleep problems, namely those with high levels of hyperarousal and damage to right frontolimbic white matter tracts. Ultimately, these results could help to inform better early detection and treatment efforts for at-risk individuals, and they warrant continued investigation into the role that white matter integrity may play in sleep disturbance, particularly following combat deployment.
Acknowledgments
We would like to acknowledge Mr. Tyron Slack’s work in data quality assessment and Dr. Kajal Claypool’s suggestions on data analysis for this study. The contents of this paper do not represent the views of the Department of Veterans Affairs or the United States Government.
Disclosure Statement
This work was supported by the US Department of Veterans Affairs grant IK2RX000707 (JBW) and the Brain Rehabilitation Research Center. The content of this paper is solely the responsibility of the authors and does not necessarily represent the official views of the Department of Veterans Affairs or the University of Florida. The authors report no conflicts of interest related to this project.
Additional information
Funding
References
- Lewis V, Creamer M, Failla S. Is poor sleep in veterans a function of post-traumatic stress disorder? Mil Med. 2009;174(9):948–51.
- Ryan MAK, Smith TC, Smith B, Amoroso P, Boyko EJ, Gray GC, Gackstetter GD, Riddle JR, Wells TS, Gumbs G, et al. Millennium Cohort: enrollment begins a 21-year contribution to understanding the impact of military service. J Clin Epidemiol. 2007;60(2):181–91.doi:10.1016/j.jclinepi.2006.05.009.
- Seelig AD, Jacobson IG, Smith B, Hooper TI, Boyko EJ, Gackstetter GD, Gehrman P, Macera CA, Smith TC. Sleep patterns before, during, and after deployment to Iraq and afghanistan. Sleep 2010;33(12):1615–22.doi:10.1093/sleep/33.12.1615.
- Alexander M, Ray MA, Hébert JR, Youngstedt SD, Zhang H, Steck SE, Bogan RK, Burch JB. The national veteran sleep disorder study: descriptive epidemiology and secular trends, 2000–2010. Sleep 2016;39(7):1399–410.doi:10.5665/sleep.5972.
- Caldwell JA, Knapik JJ, Shing TL, Kardouni JR, Lieberman HR. The association of insomnia and sleep apnea with deployment and combat exposure in the entire population of US army soldiers from 1997 to 2011: a retrospective cohort investigation. Sleep [Internet]. 2019 [cited 2020 Apr 13];42. Available from: 8 10.1093/sleep/zsz112
- Capaldi VF, Guerrero ML, Killgore WDS. Sleep disruptions among returning combat veterans from Iraq and Afghanistan. Mil Med. 2011;176(8):879–88.doi:10.7205/MILMED-D-10-00440.
- Mysliwiec V, Gill J, Lee H, Baxter T, Pierce R, Barr TL, Krakow B, Roth BJ. Sleep disorders in US military personnel. Chest 2013;144(2):549–57.doi:10.1378/chest.13-0088.
- Neylan TC, Marmar CR, Metzler TJ, Weiss DS, Zatzick DF, Delucchi KL, Wu RM, Schoenfeld FB. Sleep disturbances in the Vietnam generation: findings from a nationally representative sample of male Vietnam veterans. AJP 1998;155(7):929–33.doi:10.1176/ajp.155.7.929.
- Plumb TR, Peachey JT, Zelman DC. Sleep disturbance is common among servicemembers and veterans of operations enduring freedom and Iraqi freedom. Psychol Serv. 2014;11(2):209–19.doi:10.1037/a0034958.
- Hoge CW, Auchterlonie JL, Milliken CS. Mental health problems, use of mental health services, and attrition from military service after returning from deployment to Iraq or Afghanistan. JAMA 2006;295(9):1023–32.doi:10.1001/jama.295.9.1023.
- O’Donnell T, Hegadoren KM, Coupland NC. Noradrenergic mechanisms in the pathophysiology of post-traumatic stress disorder. Neuropsychobiology 2004;50(4):273–83.doi:10.1159/000080952.
- Mitchell HA, Weinshenker D. Good Night and Good Luck: norepinephrine in sleep pharmacology. Biochem Pharmacol. 2010;79(6):801–09.doi:10.1016/j.bcp.2009.10.004.
- Pace-Schott EF, Germain A, Milad MR. Sleep and REM sleep disturbance in the pathophysiology of PTSD: the role of extinction memory. Biol Mood Anxiety Disord. 2015;5:3.
- van Wyk M, Thomas KGF, Solms M, Lipinska G. Prominence of hyperarousal symptoms explains variability of sleep disruption in posttraumatic stress disorder. Psychological Trauma: Theory, Research, Practice, and Policy. 2016;8(6):688–96.doi:10.1037/tra0000115.
- Germain A. Sleep disturbances as the hallmark of PTSD: where are we now? Am J Psychiatry. 2013;170(4):372–82.doi:10.1176/appi.ajp.2012.12040432.
- Shen Y-C, Arkes J, Pilgrim J. The effects of deployment intensity on post-traumatic stress disorder: 2002–2006. Mil Med. 2009;174(3):217–23.doi:10.7205/MILMED-D-03-4307.
- Spoormaker VI, Montgomery P. Disturbed sleep in post-traumatic stress disorder: secondary symptom or core feature? Sleep Med Rev. 2008;12(3):169–84.doi:10.1016/j.smrv.2007.08.008.
- Department of Veterans Affairs. VA/DoD Clinical practice guideline for the management of concussion-mild traumatic brain injury (version 2.0). 2016.
- Lindquist LK, Love HC, Elbogen EB.Traumatic brain injury in Iraq and Afghanistan veterans: new results from a national random sample study. J Neuropsychiatry Clin Neurosci. 2017;29(3):254–59.doi:10.1176/appi.neuropsych.16050100.
- Grima N, Ponsford J, Rajaratnam SM, Mansfield D, Pase MP. Sleep disturbances in traumatic brain injury: a meta-analysis. J Clin Sleep Med. 2016;12(3):419–28.doi:10.5664/jcsm.5598.
- Mathias JL, Alvaro PK.Prevalence of sleep disturbances, disorders, and problems following traumatic brain injury: a meta-analysis. Sleep Med. 2012;13(7):898–905.doi:10.1016/j.sleep.2012.04.006.
- Ouellet M-C, Beaulieu-Bonneau S, Morin CM. Sleep-wake disturbances after traumatic brain injury. The Lancet Neurology. 2015;14(7):746–57.doi:10.1016/S1474-4422(15)00068-X.
- Sandsmark DK, Elliott JE, Lim MM. Sleep-wake disturbances after traumatic brain injury: synthesis of human and animal studies. Sleep 2017;40(5):40.doi:10.1093/sleep/zsx044.
- Stein MB, Jain S, Giacino JT, Levin H, Dikmen S, Nelson LD, Vassar MJ, Okonkwo DO, Diaz-Arrastia R, Robertson CS, et al. Risk of posttraumatic stress disorder and major depression in civilian patients after mild traumatic brain injury: a TRACK-TBI study. JAMA Psychiatry. 2019;76(3):249–58.doi:10.1001/jamapsychiatry.2018.4288.
- Stein MB, Kessler RC, Heeringa SG, Jain S, Campbell-Sills L, Colpe LJ, Fullerton CS, Nock MK, Sampson NA, Schoenbaum M, et al. Prospective longitudinal evaluation of the effect of deployment-acquired traumatic brain injury on posttraumatic stress and related disorders: results from the army study to assess risk and resilience in servicemembers (Army STARRS). AJP 2015;172(11):1101–11.doi:10.1176/appi.ajp.2015.14121572.
- Yurgil KA, Barkauskas DA, Vasterling JJ, Nievergelt CM, Larson GE, Schork NJ, Litz BT, Nash WP, Baker DG. Association between traumatic brain injury and risk of posttraumatic stress disorder in active-duty marines. JAMA Psychiatry. 2014;71(2):149–57.doi:10.1001/jamapsychiatry.2013.3080.
- Gilbert KS, Kark SM, Gehrman P, Bogdanova Y. Sleep disturbances, TBI and PTSD: implications for treatment and recovery. Clin Psychol Rev. 2015;40:195–212.
- Narayana PA White matter changes in patients with mild traumatic brain injury: MRI perspective. Concussion [Internet]. 2017 [cited 2020 Apr 9];2. Available from: 2 10.2217/cnc-2016-0028
- Badea A, Kamnaksh A, Anderson RJ, Calabrese E, Long JB, Agoston DV. Repeated mild blast exposure in young adult rats results in dynamic and persistent microstructural changes in the brain. Neuroimage Clin. 2018;18:60–73.
- Kulkarni P, Morrison TR, Cai X, Iriah S, Simon N, Sabrick J, Neuroth L, Ferris CF. Neuroradiological changes following single or repetitive mild TBI. Front Syst Neurosci [Internet]. 2019 [cited 2020 Jan 5];13. Available from: https://www.frontiersin.org/articles/10.3389/fnsys.2019.00034/full?report=reader.
- Niogi SN, Mukherjee P, Ghajar J, Johnson C, Kolster RA, Sarkar R, Lee H, Meeker M, Zimmerman RD, Manley GT, et al. Extent of microstructural white matter injury in postconcussive syndrome correlates with impaired cognitive reaction time: a 3T diffusion tensor imaging study of mild traumatic brain injury. AJNR Am J Neuroradiol. 2008;29(5):967–73.doi:10.3174/ajnr.A0970.
- Wright DK, Johnston LA, Kershaw J, Ordidge R, O’Brien TJ, Shultz SR. Changes in apparent fiber density and track-weighted imaging metrics in white matter following experimental traumatic brain injury. J Neurotrauma. 2017;34(13):2109–18.doi:10.1089/neu.2016.4730.
- Averill CL, Averill LA, Wrocklage KM, Scott JC, Akiki TJ, Schweinsburg B, Southwick SM, Krystal JH, Abdallah CG. Altered white matter diffusivity of the cingulum angular bundle in posttraumatic stress disorder. MNP 2018;4:75–82.
- Dennis EL, Disner SG, Fani N, Salminen LE, Logue M, Clarke EK, Haswell CC, Averill CL, Baugh LA, Bomyea J, et al. Altered white matter microstructural organization in posttraumatic stress disorder across 3047 adults: results from the PGC-ENIGMA PTSD consortium. Mol Psychiatry. 2019;24: 1–16.
- Fani N, King TZ, Jovanovic T, Glover EM, Bradley B, Choi K, Ely T, Gutman DA, Ressler KJ. White matter integrity in highly traumatized adults with and without post-traumatic stress disorder. Neuropsychopharmacol 2012;37(12):2740–46.doi:10.1038/npp.2012.146.
- Koch SBJ, van Zuiden M, Nawijn L, Frijling JL, Veltman DJ, Olff M. Decreased uncinate fasciculus tract integrity in male and female patients with PTSD: a diffusion tensor imaging study. Journal of Psychiatry & Neuroscience. 2017;42(5):331–42.doi:10.1503/jpn.160129.
- Lepage C, de Pierrefeu A, Koerte IK, Coleman MJ, Pasternak O, Grant G, Marx CE, Morey RA, Flashman LA, George MS, et al. White matter abnormalities in mild traumatic brain injury with and without post-traumatic stress disorder: a subject-specific diffusion tensor imaging study. Brain Imaging Behav. 2018;12(3):870–81.doi:10.1007/s11682-017-9744-5.
- Sanjuan PM, Thoma R, Claus ED, Mays N, Caprihan A. Reduced white matter integrity in the cingulum and anterior corona radiata in posttraumatic stress disorder in male combat veterans: a diffusion tensor imaging study. Psychiatry Res. 2013;214(3):260–68.doi:10.1016/j.pscychresns.2013.09.002.
- Santhanam P, Teslovich T, Wilson SH, Yeh P-H, Oakes TR, Weaver LK. Decreases in white matter integrity of ventro-limbic pathway linked to post-traumatic stress disorder in mild traumatic brain injury. J Neurotrauma. 2019;36(7):1093–98.doi:10.1089/neu.2017.5541.
- Antonow-Schlorke I, Helgert A, Gey C, Coksaygan T, Schubert H, Nathanielsz PW, Witte OW, Schwab M. Adverse effects of antenatal glucocorticoids on cerebral myelination in sheep. Obstet Gynecol. 2009;113(1):142–51.doi:10.1097/AOG.0b013e3181924d3b.
- Sherin JE, Nemeroff CB. Post-traumatic stress disorder: the neurobiological impact of psychological trauma. Dialogues Clin Neurosci. 2011;13:263–78.
- Uno H, Eisele S, Sakai A, Shelton S, Baker E, DeJesus O, Holden J. Neurotoxicity of glucocorticoids in the primate brain. Horm Behav. 1994;28(4):336–48.doi:10.1006/hbeh.1994.1030.
- Williamson JB, Heilman KM, Porges EC, Lamb DG, Porges SW. A possible mechanism for PTSD symptoms in patients with traumatic brain injury: central autonomic network disruption. Front Neuroeng [Internet]. 2013 [cited 2019 Oct 23];6. Available from: 10.3389/fneng.2013.00013
- Bhatia K, Henderson L, Yim M, Hsu E, Dhaliwal R. Diffusion tensor imaging investigation of uncinate fasciculus anatomy in healthy controls: description of a subgenual stem. NPS 2017;75:132–40.
- Hornberger M, Geng J, Hodges JR. Convergent grey and white matter evidence of orbitofrontal cortex changes related to disinhibition in behavioural variant frontotemporal dementia. Brain 2011;134(9):2502–12.doi:10.1093/brain/awr173.
- Mincic AM. Neuroanatomical correlates of negative emotionality-related traits: a systematic review and meta-analysis. Neuropsychologia 2015;77:97–118.doi:10.1016/j.neuropsychologia.2015.08.007.
- Fink AM, Bronas UG, Calik MW.Autonomic regulation during sleep and wakefulness: a review with implications for defining the pathophysiology of neurological disorders. Clin Auton Res. 2018;28(6):509–18.doi:10.1007/s10286-018-0560-9.
- Kalmbach DA, Cuamatzi-Castelan AS, Tonnu CV, Tran KM, Anderson JR, Roth T, Drake CL. Hyperarousal and sleep reactivity in insomnia: current insights. Nat Sci Sleep. 2018;10:193–201.
- Zoccoli G, Amici R. Sleep and autonomic nervous system. Current Opinion in Physiology. 2020;15:128–33.doi:10.1016/j.cophys.2020.01.002.
- Craig ADB. Forebrain emotional asymmetry: a neuroanatomical basis? Trends Cogn Sci (Regul Ed). 2005;9(12):566–71.doi:10.1016/j.tics.2005.10.005.
- Oppenheimer S. Forebrain lateralization of cardiovascular function: physiology and clinical correlates. Ann Neurol. 2001;49(5):555–56.doi:10.1002/ana.1038.
- Wittling W, Block A, Genzel S, Schweiger E. Hemisphere asymmetry in parasympathetic control of the heart. Neuropsychologia 1998;36(5):461–68.doi:10.1016/S0028-3932(97)00129-2.
- Williamson JB, Porges EC, Lamb DG, Porges SW. Maladaptive autonomic regulation in PTSD accelerates physiological aging. Front Psychol [Internet]. 2015 [cited 2020 Jul 27];5. Available from: https://www.frontiersin.org/articles/10.3389/fpsyg.2014.01571/full.
- Wilson MA, Liberzon I, Lindsey ML, Lokshina Y, Risbrough VB, Sah R, Wood SK, Williamson JB, Spinale FG. Common pathways and communication between the brain and heart: connecting post-traumatic stress disorder and heart failure. Stress 2019;22(5):530–47.doi:10.1080/10253890.2019.1621283.
- Balba NM, Elliott JE, Weymann KB, Opel RA, Duke JW, Oken BS, Morasco BJ, Heinricher MM, Lim MM. Increased sleep disturbances and pain in veterans with comorbid traumatic brain injury and posttraumatic stress disorder. J Clin Sleep Med. 2018;14(11):1865–78.doi:10.5664/jcsm.7482.
- Gellis LA, Gehrman PR, Mavandadi S, Oslin DW. Predictors of sleep disturbances in operation Iraqi freedom/operation enduring freedom veterans reporting a trauma. Mil Med. 2010;175(8):567–73.doi:10.7205/MILMED-D-09-00123.
- King PR, Donnelly KT, Warner G, Wade M, Pigeon WR. The natural history of sleep disturbance among OEF/OIF veterans with TBI and PTSD and the role of proxy variables in its measurement. J Psychosom Res. 2017;96:60–66.
- Mellman TA, David D, Bustamante V, Torres J, Fins A. Dreams in the acute aftermath of trauma and their relationship to PTSD. J Trauma Stress. 2001;14(1):241–47.doi:10.1023/A:1007812321136.
- Short NA, Raines AM, Oglesby ME, Zvolensky MJ, Schmidt NB. Insomnia and emotion dysregulation: independent and interactive associations with posttraumatic stress symptoms among trauma-exposed smokers. J Affect Disord. 2014;165:159–65.
- Harnett NG, Ference EW, Knight AJ, Knight DC. White matter microstructure varies with post-traumatic stress severity following medical trauma. Brain Imaging Behav [Internet]. 2018 [cited 2018 Jul 24]; Available from: 14 4 10.1007/s11682-018-9995-9
- Corrigan JD, Bogner J. Initial reliability and validity of the Ohio state university TBI identification method. J Head Trauma Rehabil. 2007;22(6):318–29.doi:10.1097/01.HTR.0000300227.67748.77.
- American Psychiatric Association. Diagnostic and statistical manual of mental disorders. 4th ed. ed. Washington, DC: American Psychiatric Publishing, Inc.; 1994.
- Weathers FW, Litz BT, Herman DS, Husaka JA, Keane TM. The PTSD checklist: reliability. TX: validity and diagnostic utility. Presented at the Annual Meeting of the International Society for Traumatic Stress Studies San Antonio; 1993.
- Buysse DJ, Reynolds CF, Monk TH, Berman SR, Kupfer DJ. The Pittsburgh sleep quality index: a new instrument for psychiatric practice and research. Psychiatry Res. 1989;28(2):193–213.doi:10.1016/0165-1781(89)90047-4.
- Wilkins KC, Lang AJ, Norman SB. Synthesis of the psychometric properties of the PTSD checklist (PCL) military, civilian, and specific versions. Depress Anxiety. 2011;28(7):596–606.doi:10.1002/da.20837.
- Williams JL, Monahan CJ, McDevitt-Murphy ME. Factor structure of the PTSD checklist in a sample of OEF/OIF veterans presenting to primary care: specific and nonspecific aspects of dysphoria. J Psychopathol Behav Assess. 2011;33(4):514–22.doi:10.1007/s10862-011-9248-3.
- Forbes D, Creamer M, Biddle D. The validity of the PTSD checklist as a measure of symptomatic change in combat-related PTSD. Behav Res Ther. 2001;39(8):977–86.doi:10.1016/S0005-7967(00)00084-X.
- Mollayeva T, Thurairajah P, Burton K, Mollayeva S, Shapiro CM, Colantonio A. The Pittsburgh sleep quality index as a screening tool for sleep dysfunction in clinical and non-clinical samples: a systematic review and meta-analysis. Sleep Med Rev. 2016;25:52–73.
- Fischl B. FreeSurfer. Neuroimage. 2012;62(2):774–81.doi:10.1016/j.neuroimage.2012.01.021.
- Yendiki A, Panneck P, Srinivasan P, Stevens A, Zöllei L, Augustinack J, Wang R, Salat D, Ehrlich S, Behrens T, et al. Automated probabilistic reconstruction of white-matter pathways in health and disease using an atlas of the underlying anatomy. Front Neuroinform [Internet]. 2011 [cited 2019 Oct 24];5. Available from: https://www.ncbi.nlm.nih.gov/pmc/articles/PMC3193073/.
- Alexander AL, Hasan KM, Lazar M, Tsuruda JS, Parker DL. Analysis of partial volume effects in diffusion-tensor MRI. Magn Reson Med. 2001;45(5):770–80.doi:10.1002/mrm.1105.
- Roine T, Jeurissen B, Perrone D, Aelterman J, Leemans A, Philips W, Sijbers J. Isotropic non-white matter partial volume effects in constrained spherical deconvolution. Front Neuroinform [Internet]. 2014 [cited 2021 Apr 22];8. Available from: https://www.frontiersin.org/articles/10.3389/fninf.2014.00028/full.
- Liu H, Yang Y, Xia Y, Zhu W, Leak RK, Wei Z, Wang J, Hu X. Aging of cerebral white matter. Ageing Res Rev. 2017;34:64–76.
- Trotter BB, Robinson ME, Milberg WP, McGlinchey RE, Salat DH. Military blast exposure, ageing and white matter integrity. Brain 2015;138(8):2278–92.doi:10.1093/brain/awv139.
- Tibshirani TR. Regression shrinkage and selection via the lasso. Journal of the Royal Statistical Society: Series B (Methodological). 1996;58(1):267–88.doi:10.1111/j.2517-6161.1996.tb02080.x.
- Friedman J, Hastie T, Tibshirani R. Regularization paths for generalized linear models via coordinate descent. J Stat Softw. 2010;33(1):1–22.doi:10.18637/jss.v033.i01.
- Peña EA, Slate EH. Global validation of linear model assumptions. J Am Stat Assoc. 2006;101(473):341.doi:10.1198/016214505000000637.
- Raikes AC, Bajaj S, Dailey NS, Smith RS, Alkozei A, Satterfield BC, Killgore WDS. Diffusion tensor imaging (DTI) correlates of self-reported sleep quality and depression following mild traumatic brain injury. Front Neurol [Internet]. 2018 [ cited 2021 Mar 5];9. Available from:. 9 10.3389/fneur.2018.00468
- Li S, Tian J, Bauer A, Huang R, Wen H, Li M, Wang T, Xia L, Jiang G. Reduced integrity of right lateralized white matter in patients with primary insomnia: a diffusion-tensor imaging study. Radiology 2016;280(2):520–28.doi:10.1148/radiol.2016152038.
- Spiegelhalder K, Regen W, Prem M, Baglioni C, Nissen C, Feige B, Schnell S, Kiselev VG, Hennig J, Riemann D. Reduced anterior internal capsule white matter integrity in primary insomnia. Hum Brain Mapp. 2014;35(7):3431–38.doi:10.1002/hbm.22412.
- Sexton CE, Zsoldos E, Filippini N, Griffanti L, Winkler A, Mahmood A, Allan CL, Topiwala A, Kyle SD, Spiegelhalder K, et al. Associations between self-reported sleep quality and white matter in community-dwelling older adults: a prospective cohort study. Hum Brain Mapp. 2017;38(11):5465–73.doi:10.1002/hbm.23739.
- Yoo -S-S, Gujar N, Hu P, Jolesz FA, Walker MP. The human emotional brain without sleep—a prefrontal amygdala disconnect. Current Biology. 2007;17(20):R877–R878.doi:10.1016/j.cub.2007.08.007.
- Hayes JP, Miller DR, Lafleche G, Salat DH, Verfaellie M. The nature of white matter abnormalities in blast-related mild traumatic brain injury. Neuroimage Clin. 2015;8:148–56.
- Johnson CP, Juranek J, Kramer LA, Prasad MR, Swank PR, Ewing-Cobbs L. Predicting behavioral deficits in pediatric traumatic brain injury through uncinate fasciculus integrity. J Int Neuropsychol Soc. 2011;17(4):663–73.doi:10.1017/S1355617711000464.
- Klimova A, Korgaonkar MS, Whitford T, Bryant RA. Diffusion tensor imaging analysis of mild traumatic brain injury and posttraumatic stress disorder. BPS CNNI. 2019;4:81–90.
- Burtis DB, Heilman KM, Mo J, Wang C, Lewis GF, Davilla MI, Ding M, Porges SW, Williamson JB. The effects of constrained left versus right monocular viewing on the autonomic nervous system. Biol Psychol. 2014;100:79–85.
- Davidson RJ. Anterior cerebral asymmetry and the nature of emotion. Brain Cogn. 1992;20(1):125–51.doi:10.1016/0278-2626(92)90065-T.
- Davidson RJ, Hugdahl K. Brain Asymmetry. Cambridge, MA: MIT Press; 1996.
- Heilman KM, Schwartz HD, Watson RT. Hypoarousal in patients with the neglect syndrome and emotional indifference. Neurology 1978;28(3):229–32.doi:10.1212/WNL.28.3.229.
- McGinley JJ, Friedman BH. Autonomic responses to lateralized cold pressor and facial cooling tasks. Psychophysiology 2015;52(3):416–24.doi:10.1111/psyp.12332.
- Gottesmann C. The involvement of noradrenaline in rapid eye movement sleep mentation. Front Neurol [Internet]. 2011 [cited 2019 Dec 29];2. Available from: 10.3389/fneur.2011.00081
- Takahashi K, Kayama Y, Lin JS, Sakai K. Locus coeruleus neuronal activity during the sleep-waking cycle in mice. Neuroscience 2010;169(3):1115–26.doi:10.1016/j.neuroscience.2010.06.009.
- Geracioti TD, Baker DG, Ekhator NN, West SA, Hill KK, Bruce AB, Schmidt D, Rounds-Kugler B, Yehuda R, Keck PE, et al. CSF norepinephrine concentrations in posttraumatic stress disorder. Am J Psychiatry. 2001;158(8):1227–30.doi:10.1176/appi.ajp.158.8.1227.
- Mellman TA, Kumar A, Kulick-Bell R, Kumar M, Nolan B. Nocturnal/daytime urine noradrenergic measures and sleep in combat-related PTSD. Biol Psychiatry. 1995;38(3):174–79.doi:10.1016/0006-3223(94)00238-X.
- Lamb DG, Porges EC, Lewis GF, Williamson JB. Non-invasive vagal nerve stimulation effects on hyperarousal and autonomic state in patients with posttraumatic stress disorder and history of mild traumatic brain injury: preliminary evidence. Front Med [Internet]. 2017 [cited 2019 Dec 29];4. Available from: https://www.frontiersin.org/articles/10.3389/fmed.2017.00124/full.
- Tournier J-D, Calamante F, Connelly A. Determination of the appropriate b value and number of gradient directions for high-angular-resolution diffusion-weighted imaging. NMR Biomed. 2013;26(12):1775–86.doi:10.1002/nbm.3017.
- Yamada H, Abe O, Shizukuishi T, Kikuta J, Shinozaki T, Dezawa K, Nagano A, Matsuda M, Haradome H, Imamura Y. Efficacy of distortion correction on diffusion imaging: comparison of FSL eddy and eddy_correct using 30 and 60 directions diffusion encoding. PLoS One. 2014;9(11):e112411.doi:10.1371/journal.pone.0112411.
- Yendiki A, Koldewyn K, Kakunoori S, Kanwisher N, Fischl B. Spurious group differences due to head motion in a diffusion MRI study. Neuroimage 2014;88:79–90.
- Bach M, Laun FB, Leemans A, Tax CMW, Biessels GJ, Stieltjes B, Maier-Hein KH. Methodological considerations on tract-based spatial statistics (TBSS). NeuroImage 2014;100:358–69.
- Tournier J-D, Calamante F, Connelly A. Robust determination of the fibre orientation distribution in diffusion MRI: non-negativity constrained super-resolved spherical deconvolution. NeuroImage 2007;35(4):1459–72.doi:10.1016/j.neuroimage.2007.02.016.
- Jeurissen B, Leemans A, Tournier J-D, Sijbers J. Investigating the prevalence of complex fiber configurations in white matter tissue with diffusion magnetic resonance imaging. Hum Brain Mapp. 2013;34(11):2747–66.doi:10.1002/hbm.22099.