ABSTRACT
Background
Cardiovascular changes, such as altered heart rate and blood pressure, have been identified in some individuals following mild traumatic brain injury (mTBI) and may be related to disturbances of the autonomic nervous system and cerebral blood flow.
Methods
We conducted a scoping review according to PRISMA-ScR guidelines across six databases (Medline, CINAHL, Web of Science, PsychInfo, SportDiscus and Google Scholar) to explore literature examining both cardiovascular parameters and neuroimaging modalities following mTBI, with the aim of better understanding the pathophysiological basis of cardiovascular autonomic changes associated with mTBI.
Results
Twenty-nine studies were included and two main research approaches emerged from data synthesis. Firstly, more than half the studies used transcranial Doppler ultrasound and found evidence of cerebral blood flow impairments that persisted beyond symptom resolution. Secondly, studies utilizing advanced MRI identified microstructural injury within brain regions responsible for cardiac autonomic function, providing preliminary evidence that cardiovascular autonomic changes are a consequence of injury to these areas.
Conclusion
Neuroimaging modalities hold considerable potential to aid understanding of the complex relationship between cardiovascular changes and brain pathophysiology associated with mTBI. However, it is difficult to draw definitive conclusions from the available data due to variability in study methodology and terminology.
Introduction
Mild traumatic brain injury (mTBI), also commonly referred to as concussion (Citation1), is the most common type of traumatic brain injury, affecting an estimated 600 per 100,000 people globally (Citation2). mTBI is characterized by transient alterations in brain function as a result of external biomechanical forces or trauma applied to the head or body (Citation3–5), and may occur from mechanisms such as falls, sports injuries, road traffic incidents, military injuries and interpersonal violence (Citation1,Citation6). Symptoms and functional impairments will generally resolve in the weeks following injury, however approximately 50% of the people experience symptoms that may persist for months or even years (Citation7), particularly amongst non-sport related mTBI populations (Citation8). Persisting symptoms may adversely impact many aspects of an individual’s life including their ability to return to work, study and sport, and significantly contribute to the public health burden (Citation6,Citation9).
Presenting signs and symptoms following mTBI can vary significantly between individuals, and it is increasingly recognized that the heterogeneity in mTBI presentations may be a consequence of injury to different brain regions, as well as secondary physiological sequelae in other systems throughout the body (Citation10). Alterations in heart rate and blood pressure following traumatic brain injury have been recognized for some time, with Raab (1949) reporting case studies of two individuals with ‘concussion of the brain’ (Citation11) who experienced hypertension and tachycardia following head injury. Other authors have also reported cardiovascular changes, although there is conflicting evidence in the literature regarding the patterns of change (Citation12), with resting heart rate variously shown to be elevated (Citation13,Citation14), unchanged (Citation15,Citation16) or lowered (Citation17) compared to control participants. Individuals who have recently experienced mTBI are also reported to have lower initial heart rates at exercise onset, and lower than expected heart rates combined with greater perceptions of effort at maximum exertion (Citation18). Altered heart rate variability (HRV), blunted baroreflex responses (Citation13,Citation19,Citation20) and altered blood pressure (Citation21,Citation22) have also been noted after mTBI. Most studies examining HRV have not identified any differences at rest following mTBI, with HRV reductions instead observed during steady-state exercise (Citation23) and other test conditions (Citation24). Alterations in both systolic and diastolic blood pressure have been described: Dobson and colleagues noted significantly higher resting systolic blood pressure within the first 48 h following concussion compared to control participants (Citation21), whilst Balestrini and colleagues (Citation22) found that diastolic blood pressure was elevated in concussed adolescents at initial assessment, but resolved by clinical discharge (approximately 37 days).
The cardiovascular system works in conjunction with the cerebrovascular system in a complex regulatory network to ensure optimal blood supply to the brain using three main mechanisms (Citation25,Citation26). Cerebral autoregulation maintains cerebral blood flow (CBF) at a constant level by compensating for fluctuations in systemic arterial blood pressure; cerebral vascular reactivity (or cerebral vasoreactivity) alters blood flow in response to circulating arterial blood gases, in particular CO2; and neurovascular coupling mediates increased blood flow in response to the demands of localized neuronal activity and metabolism (Citation25,Citation26). Each of these responses are influenced by both the sympathetic and parasympathetic components of the autonomic nervous system (ANS) and may be impacted by concussive injury. The central autonomic network (CAN) describes brain structures within the cerebral cortex (insula, cingulate, parietal, and medial prefrontal regions), sub-cortical areas (amygdala, hypothalamus), cerebellum, and brainstem (periaqueductal gray matter, nucleus tractus solitarius, and ventrolateral medulla), that interact to influence autonomic function (Citation27–29). Uncoupling between the cardiovascular system and CAN has been identified following severe brain injury (Citation30,Citation31), with the degree of uncoupling being proportional to injury severity (Citation31). Leddy and colleagues proposed that similar disturbances of central physiology within the brain following mild brain injury may impact systemic regulatory processes, such as heart rate and cerebral autoregulation and contribute to persisting post-concussion symptoms (Citation10). However, the pathophysiological mechanisms underpinning cardiovascular changes following mTBI has not yet been well characterized (Citation10,Citation12,Citation32,Citation33).
A number of neuroimaging techniques have shown promise in detecting alterations in brain structure and function related to mTBI (Citation34–36), and these modalities may provide further insight into the mechanisms of cardiac autonomic changes following mTBI. Routinely used diagnostic imaging techniques, such as CT scans and clinical MRI are useful in the acute period to identify more serious pathology, but may lack the sensitivity to detect more subtle neuropathology and functional deficits associated with mTBI (Citation37). However, a number of advanced magnetic resonance imaging (MRI) techniques used primarily in research have shown promise in detecting alterations in brain structure and function related to mTBI (Citation35). Studies using T1- and T2-weighted MRI have detected structural changes in brain volume and cortical thickness associated with symptom severity and cognitive dysfunction (Citation38). Diffusion MRI utilizes water diffusivity to provide information about white matter integrity in brain regions of interest or specific white matter tracts (Citation37,Citation38). Altered fractional anisotropy (FA), a metric of diffusion that may reflect cerebral edema and neuroinflammation, has been observed in the initial acute period after injury with normalization over the months to follow (Citation36). Functional modalities including resting-state and task-based functional MRI, and electroencephalography (EEG), are used to assess network functional connectivity within the brain (Citation35). Reduced connectivity in the default mode network (DMN), a group of interacting brain areas active during resting states (Citation39), has been identified in the acute period following mTBI, and there is some evidence that the extent of reduction is predictive of prolonged recovery (Citation36). CBF can be quantified on a global scale using transcranial Doppler ultrasound (Citation40), or to more localized brain areas using arterial spin labeling (ASL) or single photon emission computerized tomography (SPECT) (Citation36). A recent systematic review of ASL by Wang and colleagues (Citation41) found that most studies reported unchanged or reduced CBF in the first week following injury (Citation41,Citation42), although some also demonstrated an increase (Citation43,Citation44).
For the most part, neuroimaging literature in the mTBI space has primarily focused on the relationship between imaging results and neuropsychological assessments of cognition and emotion (Citation35) and motor dysfunction (Citation45). Whilst cardiovascular autonomic dysfunction and altered neuroimaging findings following mTBI have each been identified independently, they have infrequently been studied concurrently (Citation33,Citation46). As such, we conducted a scoping review to explore and summarize the literature that has examined cardiovascular autonomic changes in concert with neuroimaging modalities following mTBI, to better understand the anatomical and physiological basis for cardiac autonomic changes that may be observed following mTBI. A scoping review methodology was chosen to draw together evidence from these two diverse research areas, and to outline key concepts and knowledge gaps in the research literature. This scoping review aims to address the following research question: Can neuroimaging techniques enhance our understanding of the pathophysiology underpinning cardiovascular autonomic changes following mTBI?
Methods
A scoping review was conducted and reported according to the PRISMA-ScR guidelines (Citation47), and informed by the process outlined by Levac et al. (Citation48), and the JBI guidance for scoping reviews (Citation49). A completed PRISMA ScR checklist is included in Appendix A. The protocol was prospectively registered with Open Science Framework (https://doi.org/10.17605/OSF.IO/D9MPQ) to minimize duplication of research work and ensure a transparent process.
Search strategy
A literature search was structured using the PCC criteria (population, concept, and context) described by Peters et al., (2021). The population of interest was individuals who have sustained a mTBI, and the two concepts were (Citation1) cardiovascular parameters and (Citation2) neuroimaging modalities. The context (e.g. geographic location or specific settings) was not explicitly defined in order to keep the search broad. An academic librarian assisted with the development of the search strategy. Subject headings and keyword terms were structured in MEDLINE (see Appendix B for example Medline electronic search strategy), and then adapted as appropriate for the following databases: CINAHL, Web of Science, PsychInfo, SportDiscus and Google Scholar. The first 200 records from Google Scholar were searched for relevance. No limits were placed on the search dates and the final search was conducted independently by one researcher on March 24, 2022. A manual search was also conducted of reference lists of all retained articles.
Study selection
Studies were included in this review if they met the following criteria:
Original research studies presenting primary data from observational and experimental research designs with no restrictions placed on research design; and systematic reviews and meta-analyses.
Studies including human participants of any age and sex. Preclinical research was also considered if it contributed to the study objectives.
Studies describing participants who had sustained mTBI or concussion from any cause, and using any description consistent with definitions outlined by the American Congress of Rehabilitation Medicine (ACRM) (Citation4), World Health Organization (WHO) (Citation5) and the Berlin Consensus Statement on Concussion in Sport (Citation50). Whilst each of these definitions differ slightly, the commonalities include a Glasgow Coma Scale score of 13–15 after 30-min post-injury, and any of the following signs and symptoms: loss of consciousness less than 30 min; loss of memory for events immediately before or after the injury; alteration in mental state at the time of the injury (confusion or disorientation); and other physical signs or symptoms attributable to that injury (including but not limited to headache, dizziness, nausea). Of note, studies that reported ‘complicated mTBI’ findings (intracranial lesions not requiring surgery) were included in this review, although some definitions do not consider these ‘mild’ TBI (Citation4,Citation51).
Studies that included both cardiac parameters and neuroimaging modalities. Cardiac parameters of interest included heart rate, heart rate variability, blood pressure, and baroreflex sensitivity. For the purpose of this review, neuroimaging was defined as any modality with the capacity to visually represent structural and functional brain changes (Citation52). Specific modalities are outlined in the search terms in Appendix B.
Studies were excluded from this review if they were:
Conference abstracts/proceedings, theses, editorials or narrative reviews;
Studies of participants with only moderate and severe TBI, defined as GCS<13 on presentation. Studies that include participants with a combination of mild, moderate, and severe TBI were included if the relevant mTBI findings were reported separately;
Studies reporting only data on sub-concussive or repetitive head impacts;
Publications in languages other than English (unless translated).
Following duplicate removal, the records were imported into Research Screener (Citation53) to sort articles according to relevance, and then two reviewers independently screened the records by title and abstract. Conflicts were reviewed and resolved by discussion between the reviewers to reach a consensus. Full text screening of the final sample was then conducted by both researchers and records were retained or excluded according to the eligibility criteria outlined above. In instances where studies by the same author appeared to use the same participant cohort, the reviewers ensured that different metrics were analyzed and reported in each paper, ensuring that the criteria of individual studies were satisfied.
Data extraction, synthesis, and presentation of results
A data charting form was created using Microsoft Excel (Microsoft Corporation, Redmond, USA) and included variables of interest relating to the study objectives as follows: study characteristics (year of publication, study design, sample size, and timeframe of assessment), participant characteristics (age, sex, and population type), cardiovascular parameters, and neuroimaging modalities utilized in each study and key results relevant to the review questions. The primary reviewer extracted and documented relevant information from each of the eligible studies, and these results were then verified for accuracy by a second reviewer. Data for each variable were then categorized as appropriate to enable synthesis of results. For example, participant age was allocated into categories for children, adolescents, young adults, and adults. The figures were constructed by one reviewer using GraphPad Prism 9.0 for Windows (GraphPad software, San Diego, California, USA). Characteristics of each article are presented using a narrative synthesis of results, incorporating a quantitative description of the number of articles examining each variable of interest and a summary of key findings. A formal critical appraisal of the quality of individual studies and a risk of bias assessment were not conducted as they were considered outside the exploratory remit of this scoping review (Citation49). The results were synthesized and summarized by one author, and all authors then discussed the extracted information to reach a consensus on inferences drawn from the data.
Results
Selection of sources of evidence
provides a visual representation of evidence selection using a PRISMA-ScR flow chart. A total of 1002 potential sources of evidence were identified through database searching. 680 records remained following the removal of duplicates, and 627 citations were removed through title and abstract screening by two independent reviewers. Of the 53 citations remaining for full text review, a further 25 records were excluded. Citations for these records, as well as reasons for exclusion, are listed in full in Appendix C. One further record was identified through hand searching of reference lists of the included articles. The final review included 29 relevant publications.
Study characteristics
Study and participant characteristics are outlined in Table S1. All sources of evidence were journal articles published between 1997 and 2021. No applicable animal studies, systematic reviews or meta-analyses were identified. Most articles were published during the past decade, with only three published prior to 2010 (Citation55–57) (). The majority of studies were conducted by researchers in North America (USA and Canada), with one study from Japan (Citation58) and one from Norway (Citation56).
Figure 2. The number and type of neuroimaging studies are displayed according to year of publication. Year of publication has been divided into four yearly intervals commencing from 1997 to present. Abbreviations: CT- computerized tomography, MRI – magnetic resonance imaging, TCD – transcranial Doppler ultrasound, EEG – electroencephalography, NIRS – near infrared spectroscopy.
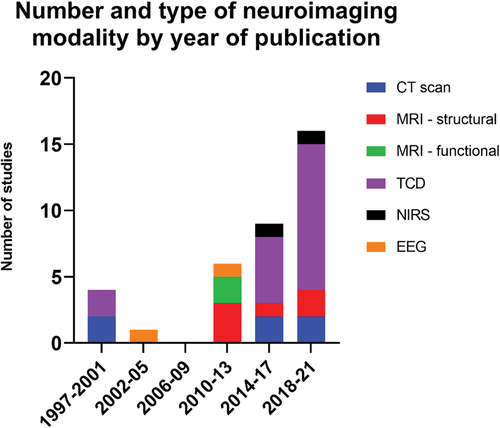
Two of the studies had large sample sizes of n = 485 (Citation59) and n = 201 (Citation60) derived from mTBI in the general population. Both these studies examined patients on initial presentation to hospital and included cardiovascular monitoring and CT scans as part of routine clinical assessments. All other studies had small to medium-sized samples, with eight studies having less than 10 participants (Citation54,Citation57,Citation58,Citation61–65), sixteen studies with between 10 and 29 participants, two studies with 30 to 49 participants (Citation46,Citation66), and three studies having more than 50 participants (Citation59,Citation60,Citation67). Three studies were single-case reports outlining the clinical presentation of patients who had atypical mTBI experiences (Citation54,Citation57,Citation64), 20 were case-control studies with a comparison group of age- and sex-matched healthy controls (Citation15,Citation16,Citation46,Citation55,Citation56,Citation58,Citation61,Citation62,Citation65,Citation66,Citation68–77), and six were cohort studies with no control group (Citation59,Citation60,Citation63,Citation67,Citation78,Citation79). Of the case-control studies, three were intervention studies incorporating aerobic exercise-based interventions and/or a placebo stretching group (Citation61,Citation62,Citation65).
The length of time following mTBI to inclusion in the study was categorized based upon typical time periods for symptom resolution and/or physiological recovery following mTBI (Citation35,Citation46). provides an overview of the sample size and population type according to time since mTBI of the included studies. Sixteen studies assessed participants within the acute period (less than two-week post-mTBI), four studies assessed participants at a sub-acute timeframe (2 weeks to 3 months) (Citation54,Citation64,Citation65,Citation71), four studies included participants with persistent symptoms (3 months to 1 year) (Citation61,Citation62,Citation77,Citation78), and five articles included participants with chronic post-concussion symptoms (1 year or more) or a remote history of mTBI (Citation56,Citation58,Citation67,Citation69,Citation70). Eleven studies were longitudinal in nature with two to three follow-up time points (Citation15,Citation16,Citation46,Citation61–63,Citation66,Citation68,Citation72,Citation73,Citation79), however for ease of reporting only the first-time point has been included in this analysis.
Figure 3. Sample size and population type are displayed according to (A) time since mTBI, and (B) age of participants. Population type is expressed in the categories of sports-related concussions (red), mTBI sustained in military service (green), and mTBI sustained in the general population resulting from causes such as falls, motor vehicle accidents and interpersonal violence (blue). In the literature this may be described as ‘community-dwelling adults’ (Citation70) or ‘mixed populations’ including some athletes and some non-athletes (Citation61,Citation62). Age has been classified according to mean age of study participants.
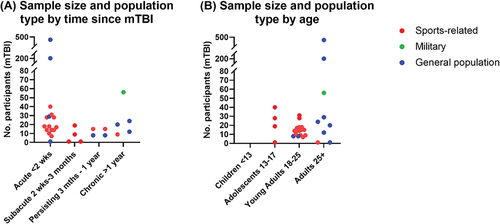
Participant characteristics
Studies were categorized into four groups based upon participant age and neurodevelopment, with recognition that brain maturation is not complete until approximately 25 years of age (Citation80), as shown in . None of the identified studies included children under the age of 13 years. Four articles included adolescents aged 13 to 17 years (Citation54,Citation66,Citation68,Citation71), 15 studies included young adults from 18 to 24 (Citation15,Citation16,Citation46,Citation58,Citation61–63,Citation65,Citation72,Citation73,Citation75–79), and nine included adults older than 25 years (Citation55–57,Citation59,Citation60,Citation64,Citation67,Citation69,Citation70). The majority of studies with adolescents and young adults had participants who had experienced sports-related concussion (predominantly college or university athletes), whilst those that included adult participants were predominantly from the general population. There was only one study that included participants who had sustained mTBI while in the military (Citation67). Four studies included only female participants (all single-case studies) (Citation54,Citation57,Citation64,Citation65), six studies included only males (Citation15,Citation16,Citation58,Citation67,Citation72,Citation79), and 18 studies included both males and females. One study did not specifically state the age or sex of the participants (Citation63). Whilst most studies did not present results for males and females separately, two studies stated that there was no sex-related difference in vasoreactivity (Citation78) or rate of regulation of cerebral blood flow (Citation71). In a cohort of older adults with a history of mTBI, only males exhibited an association between higher systolic blood pressure and lower cortical perfusion (Citation70)
Synthesis of evidence
Table S1 presents data relevant to the objectives of this review, in particular, characteristics of the mTBI sample population of each study, cardiovascular parameters and conditions of testing, neuroimaging modalities and key results from each study. Table S1 has been arranged according to participant age group (adolescent, young adults, adults over 25 years) to facilitate analysis of potential age-related alterations in autonomic function. The sections to follow will first outline relevant cardiovascular and neuroimaging findings separately, and then summarize studies that include both concepts.
Findings related to cardiovascular parameters
Most articles included either heart rate (n = 25) or blood pressure (n = 24) measures as part of their assessment protocol. Cardiovascular parameters were primarily assessed at rest (n = 19) as well as during exertional activities (n = 13) such as treadmill or stationary bike exercise protocols (Citation61,Citation62,Citation64–66,Citation72,Citation74,Citation76,Citation77), or during a sit-stand maneuver (Citation16,Citation69,Citation79). Four studies used conditions of hypo- or hypercapnia (Citation65,Citation68,Citation73,Citation78), and five studies utilized blood pressure challenges to elicit changes in CBF regulation (Citation55,Citation57,Citation58,Citation75,Citation77). Three studies utilized a mental or visual stimulus (Citation15,Citation56,Citation63), two used Valsalva maneuver (Citation54,Citation77), two monitored heart rate and blood pressure whilst undertaking MRI (Citation74,Citation76), and three reported heart rate and blood pressure taken as part of routine clinical assessments in a hospital emergency department (Citation59,Citation60,Citation67). Only four studies reported HRV parameters (Citation46,Citation68,Citation69,Citation77), whilst baroreflex sensitivity was calculated in two studies in association with cerebrovascular reactivity and autoregulation (Citation69,Citation75).
Studies that identified cardiovascular changes at rest were primarily conducted during the very early acute phase following injury. One study found that mean heart rate was higher in those with minor head injury compared to controls in the first 48 h following injury (Citation55), one identified reduced arterial pressure at rest when assessed at Day 3 (Citation63), and one observed lower pNN50, a measure of HRV, at day 3 which normalized by day 21 (Citation46). A further two studies identified blood pressure recorded at hospital admission as a significant contributor to models predicting outcome at 90 and 180 days (Citation59,Citation60). Studies that reported cardiovascular variables at rest when measured later post-injury found no difference compared to healthy controls. Three of these studies were conducted in the later acute phase (Citation68,Citation74,Citation75), but most were conducted in the sub-acute (Citation71), persistent (Citation77,Citation78) or chronic phases (Citation56,Citation58,Citation67,Citation69). Differences in heart rate in response to exertion were noted in three studies, with elevated heart rate during the first minute of exercise onset in concussed individuals compared to controls (Citation65) and lower heart rate at the point of maximum exercise tolerance, which normalized following a sub-threshold aerobic exercise intervention (Citation61,Citation62,Citation65). Shannon et al. also noted higher mean systolic blood pressure during the tilt table test in previously concussed individuals (Citation77).
Findings related to neuroimaging modalities
The most commonly used neuroimaging modality identified in this review was transcranial Doppler ultrasound, with 18 studies using this technique to identify impairments in cerebral autoregulation, cerebral vasoreactivity, and neurovascular coupling (Citation15,Citation16,Citation46,Citation55,Citation57,Citation58,Citation63–66,Citation68,Citation69,Citation71,Citation73,Citation75,Citation77–79). Of note, one study using transcranial Doppler determined that cerebral autoregulation was impaired or absent in 28% of participants with minor head injury (Citation55) and another single-case study also described a patient who exhibited complete absence of autoregulation (Citation57). Other studies found that cerebral autoregulation was impaired at 72 h following injury but resolved by one month (Citation16), and that concussed participants had reduced rates of regulation compared to healthy controls at 1 month, which improved after a 12-week rehabilitation period (Citation71). One study found that impaired autoregulation more than 6 months following injury was associated with poorer cognitive performance (Citation69), although another study found no association with cognition (Citation58). Two studies identified altered cerebral vasoreactivity in the early acute period (Citation68,Citation73), with one finding vasoreactivity alterations until 90 days post-injury despite symptom resolution by 21 days (Citation73), and the other showing changes that persisted at 8 weeks, but were positively modified by exercise volume (Citation68). Higher vasoreactivity was associated with a greater symptom burden (Citation68), and specifically more severe headache and worse cognitive symptoms (Citation78). Neurovascular coupling was only examined in one study, which found that posterior cerebral artery velocity in response to visual stimulation was elevated at 72 h and remained elevated at 2 weeks despite symptom resolution. This resolved fully by 1 month, and furthermore, there was no difference noted between participants with a history of more than three prior concussions compared to those with no history of prior concussion (Citation15).
Six studies in total used MRI, with four studies using structural MRI sequences (T1/T2-weighted, DTI, FLAIR) (Citation54,Citation61,Citation67,Citation76) and three using either resting-state or task-based functional MRI (Citation62,Citation74,Citation76). Of note, one study using DTI analysis of participants with persisting symptoms found microstructural white matter changes in regions associated with cardiovascular autonomic function, including the corpus callosum, corona radiata, internal and external capsule, and brainstem (Citation61). Whilst symptoms resolved in these participants following an 8-week exercise program, the DTI changes persisted at approximately 4 months post-injury. Three studies using functional MRI also identified altered functional network connectivity in brain areas with the potential to influence autonomic function (Citation62,Citation74,Citation76). In the acute phase approximately 10 days post-injury, one study identified disrupted interhemispheric connectivity both at rest and following an exercise stress test (Citation74), whilst another found no change in the DMN at rest, but identified changes in connectivity between the posterior cingulate cortex and parietal cortex following exercise (Citation76). Leddy and colleagues identified lower activation in the posterior cingulate gyrus and cerebellum in all participants with persisting symptoms of greater than 6-week duration. These changes resolved in participants who undertook a 12-week exercise program but remained the same in a placebo stretching group (Citation62). In military veterans with long-term persistent symptoms, Piantino and colleagues found an association between the number and volume of perivascular spaces and severity of symptoms (Citation67). CT scans were used in five studies, primarily in an emergency department setting to characterize mTBI and exclude more serious injury (Citation55,Citation57,Citation59,Citation60,Citation69), and SPECT was used in one further study of men with a history of head injury (Citation70). EEG (Citation54,Citation56) and NIRS (Citation64,Citation72) were used in two studies each.
When stratified by age, we observed an interesting insight whereby a large proportion of the studies using transcranial doppler ultrasound were conducted in adolescent and young adult age groups (Citation15,Citation16,Citation46,Citation58,Citation63,Citation65,Citation66,Citation68,Citation71,Citation73,Citation75,Citation77–79) whilst the majority of the studies using MRI and CT-based imaging were conducted in adults aged 25 years or more (Citation59,Citation60,Citation67,Citation70). The variation in study methodology and inconsistencies in terminology used makes it challenging to determine whether there are any age-related differences in autonomic findings in these studies. However, as previously outlined, it appears that cardiac autonomic alterations are more commonly seen in young adult participants, and primarily during the early acute phase following injury or with situational challenges, such as exercise. In contrast, alterations in systolic blood pressure are reported more frequently in older adult populations, both during the acute post-injury period and in the longer term. The implications of these findings are beyond the nature of this scoping review but are an important consideration for future research.
Relationship between cardiovascular parameters and neuroimaging modalities
presents the nature of the statistical analysis for each cardiovascular parameter and neuroimaging modality for each study. Although this scoping review primarily aimed to examine the association between cardiovascular parameters and neuroimaging, we were also interested in studies reporting both variables in the same cohort, even if not directly associated, in order to understand the full breadth of research in this area. Thirteen studies directly examined the statistical association between the cardiovascular and neuroimaging findings (Citation16,Citation46,Citation55,Citation57,Citation63,Citation67–72,Citation75,Citation79). Of these, 10 studies used transcranial Doppler ultrasound and as cardiovascular factors are routinely measured as a component of this assessment protocol, the association between cardiovascular and neuroimaging findings is more readily obtained. Even so, there was considerable variability in the parameters that were analyzed and reported, making it difficult to draw conclusions from these data. In studies reporting blood pressure, one study found that acute cerebral autoregulation was worse in those with lower blood pressure (Citation55) and one found that lower mean pressure and lower vasoreactivity were associated with greater symptom burden (Citation68). Furthermore, one study found that the synchronization of cerebral autoregulation and blood pressure were reduced acutely compared to pre-season values and indicated that this was the first evidence that autonomic dysfunction influences cerebrovascular dynamics as well as cardiovascular regulation (Citation16). In those with a history of mTBI more than 3 years prior, one study identified an association between systolic blood pressure and the number and volume of perivascular spaces (Citation67) using structural MRI (T1-weighted and FLAIR), and another found that higher systolic blood pressure was correlated with lower perfusion to cortical brain areas using SPECT imaging in those with a remote history of mTBI (Citation70). Purkayastha and colleagues identified an association between blood flow velocity in the acute stage and HRV (pNN50) during the sub-acute phases at day 21 and day 90 (Citation46), and Neary and colleagues found a significant positive relationship between cerebral hemodynamics measured using NIRS and exercise intensity at specific heart rate ranges (Citation72). None of the studies that examined microstructural and functional deficits using MRI specifically analyzed the relationship between neuroimaging and cardiovascular findings (Citation61,Citation62,Citation74,Citation76), and this represents a shortfall in the literature in this area of research.
Table 1. The nature of the statistical analysis for each cardiovascular parameter and neuroimaging modality for each study.
Discussion
This scoping review aimed to assess the utility of neuroimaging techniques to enhance our understanding of the pathophysiology of cardiovascular autonomic changes associated with mTBI by drawing together evidence from two exploratory research areas. We evaluated 29 studies examining both cardiovascular changes and neuroimaging, and identified two predominant research approaches to neuroimaging for mTBI. The first approach, used by more than half the studies identified in this scoping review, used transcranial Doppler ultrasound to assess the relationship between cardiovascular parameters, particularly blood pressure, and cerebrovascular regulation. This speaks to an awareness of the importance that CBF plays in post-mTBI physiology, and will be discussed in more depth to follow. The second approach assessed structural and functional brain changes using CT and MRI, either as part of routine clinical imaging or using advanced MRI modalities. Three MRI-based studies detected microstructural injury within brain regions aligned with cardiac autonomic function including the corpus callosum and brainstem (Citation61); posterior cingulate cortex (Citation62,Citation76) and cerebellum (Citation62). These are consistent with brain areas of the central autonomic network (Citation27). It has been proposed that cardiovascular autonomic changes may potentially be a direct consequence of injury to these areas (Citation19,Citation61). Whilst both research approaches offer preliminary insight into the anatomical and physiological basis of cardiovascular autonomic changes following mTBI, interpretation of study findings identified in this review is complicated by considerable variability in study methodology, parameters analyzed and reported, participant populations and time points post-injury, making it difficult to draw meaningful and definitive conclusions from these data.
The earliest studies identified in this review were published in 1997 and both examined cerebral autoregulation using transcranial Doppler ultrasound (Citation55,Citation57). This method of quantifying CBF has continued to be commonly utilized, likely because it is inexpensive, readily available and noninvasive, and can be conducted simultaneously with cardiovascular monitoring during different conditions of testing, such as exercise (Citation40,Citation81). Transcranial Doppler can provide an overall indication of CBF quantity and control, but is not able to identify perfusion to specific areas of the brain (Citation66). Advanced MRI modalities, such as arterial spin labeling (ASL) and functional MRI offer better spatial resolution and may provide evidence of neurovascular coupling as a determinant of CBF (Citation26). Of note, no identified studies used ASL as a method of assessing cerebral perfusion together with assessments of cardiovascular changes, and this remains an area of interest for further research. There appeared to be increased uptake of MRI-based techniques during the past decade, and this likely reflects the fact that evolving technology combined with improved post-processing methods mean that MRI is becoming more sensitive to detect abnormalities, and also more feasible and accessible for researchers and clinicians (Citation82). A number of conference proceedings, abstracts, or theses not meeting the criteria for this review included modalities such as DTI (Citation83), ASL (Citation83), resting-state-fMRI (Citation84) and EEG (Citation85–87). All were produced after 2016, highlighting the more recent use of these techniques (Citation84).
Insights regarding cardiovascular parameters
Studies included in this review identified altered cardiovascular parameters including elevated heart rate (Citation55), lower blood pressure (Citation63) and lower HRV (Citation46). Stratifying results according to time since injury revealed that these changes were more likely to be identified during the early acute phase (first three days) (Supplementary Table A1). Cardiac autonomic changes are not typically observed at rest during the sub-acute period (Citation46), but may be detected with situational challenges, such as physical exertion (Citation65) or orthostatic positional changes (Citation77). This supports similar findings of two recent systematic reviews, with the authors of both suggesting that ongoing cardiovascular disturbances indicate an inability of the ANS to respond appropriately to physiological stressors (Citation24,Citation88). A number of studies in this review found no significant difference in cardiovascular hemodynamic variables compared to controls (Citation15,Citation68,Citation74,Citation75), which may reflect the influence of other factors controlling heart rate, including endocrine factors and the intrinsic conduction system of the heart (Citation89). Furthermore, it is recognized that not all individuals who sustain mTBI will experience physiological effects such as ANS dysfunction (Citation10,Citation32), and thus both cardiovascular and neuroimaging changes should be considered within the context of the patient’s clinical presentation (Citation36). Only four studies in this review reported HRV findings, and it is likely that this is a valuable noninvasive measure for evaluating autonomic status for future study. Wright et al. found no differences in pre-season physiological variables between those with a history of more than three concussions compared to those with no history of concussion, and suggested this indicated recovery with time (Citation16). However, some authors hypothesize that there may be an increased risk of cardiac events (Citation13) and even a six-fold increased risk of mortality (Citation90) in individuals with a history of mTBI. Given this, it seems pertinent that researchers and clinicians endeavor to fully understand cardiovascular sequelae following mTBI.
Neuroimaging to enhance our understanding of cardiac autonomic changes
The mechanism by which cardiovascular autonomic changes occur following mTBI remains unclear. One concept suggests that cardiovascular changes may occur as part of a reflex feedback mechanism involved in maintaining optimal blood supply to the brain (Citation10,Citation26). The large number of studies utilizing transcranial Doppler ultrasound to examine CBF dynamics is testament to the importance of these dynamics in mTBI physiology and likely contribution to symptom presentation. The studies in this review suggest that, in general, cerebral autoregulation may be impaired in some individuals in the acute phase but that it resolves by approximately 1 month (Citation16,Citation79). In contrast, cerebral vasoreactivity in response to arterial CO2 may remain compromised for a longer period of time (Citation73), and may be implicated in greater symptom severity (Citation68), worse cognition (Citation78) and exercise intolerance (Citation65). Importantly, CBF impairments persisted beyond symptom resolution and clinical recovery, which has implications for management in a sporting context when considering appropriate timing for return to sport or other risk-related activities. The timeline for neurobiological recovery and its clinical relevance is still to be determined (Citation91) and neuroimaging may be a valuable tool in this area of research. In men with a remote history of mild head injury, higher blood pressure was associated with lower cortical perfusion, and the authors suggested this may have potential implications for long-term adverse neurocognitive function and brain health (Citation70). Several studies in this review examined the effect of exercise rehabilitation on recovery, either by implementing an aerobic exercise intervention (Citation61,Citation62,Citation65) or monitoring the volume of exercise (Citation68). All these studies found that cardiovascular, respiratory, and cerebrovascular physiological variables improved after exercise intervention. Thus, the authors suggested that controlled, sub-symptom aerobic exercise may be beneficial in restoring cardiac autonomic modulation, CBF response and functional connectivity (Citation10,Citation62,Citation92).
Some authors have suggested that cardiovascular autonomic alterations observed following mTBI may be related to injury to brain structures associated with autonomic control (Citation10,Citation19,Citation33). Biomechanical forces associated with mTBI may cause stretching and shearing of brain microstructures leading to slowed neurotransmission and altered cerebral metabolism (Citation93). Structures in specific cortical and sub-cortical brain regions, including the corpus callosum, insular cortex, cingulate and thalamus, are known to be particularly susceptible to tractional and rotational injury mechanisms (Citation19,Citation82). As these areas form part of the CAN (Citation27) it is foreseeable that damage within these areas may result in altered autonomic control with resultant downstream cardiovascular sequelae (Citation19,Citation24). An association between HRV and altered connectivity in brain regions consistent with the CAN has been demonstrated in patients with severe brain injury (Citation30), however similar evidence is still emerging in mTBI research. A study included in this review by Polak and colleagues using DTI identified localized irregularities in sub-cortical regions consistent with the CAN, and also identified altered heart rate in response to exercise testing in participants with persisting post-concussion symptoms (Citation61). Although these changes were noted independently, the association between neuroimaging and cardiovascular parameters was not specifically reported in this study. Similarly, Slobounov and colleagues identified disrupted interhemispheric connectivity in the visual cortex, hippocampus, and dorsolateral pre-frontal cortex (Citation74), and Zhang found alterations in the DMN using resting-state fMRI following physical exertion, but neither study reported specific associations with cardiovascular parameters (Citation76). Thus, it seems there is preliminary evidence that neuroimaging has value in identifying structural and functional deficits in brain regions associated with autonomic function, however, this is yet to be fully elucidated after mTBI.
Limitations
There are a number of limitations to this scoping review. Despite being confident our search terms and screening strategy was thorough and systematic, it is possible that some relevant studies were not identified for inclusion and this may represent a source of bias with regard to the synthesis and interpretation of findings. Only peer-reviewed published articles were included in this review in order to maintain a high level of evidence, however we excluded several recent conference proceedings and theses with relevant content that may have proved insightful and indicate the direction of research in this area. Critical appraisal of individual study quality was considered outside the exploratory remit of this scoping review. However, this is a rapidly expanding field, and future systematic reviews and meta-analyses are warranted to address this shortcoming. Whilst outside the scope of the cardiovascular parameters examined in this review, it was noted that a number of studies included respiratory measures such as respiratory rate, end tidal CO2 and oxygen consumption in their assessments. It is likely that this is an important component of the feedback loop controlling CBF, and further investigation of its mechanistic action would be informative.
Synthesizing information from the neuroimaging studies included in this review was challenging due to variability in methodology and terminology used to report findings, particularly with regard to cerebrovascular regulation. This may also have been limited by what findings were reported by study authors and complicated by the likelihood of technological changes over the timeframe of the search period. This highlights the need for more unified and standardized reporting in neuroimaging, to facilitate direct comparison between study findings.
Future directions
There is a distinct lack of research examining structural or functional neuroimaging in brain regions aligned with autonomic control in association with cardiovascular alterations in individuals following mTBI. To address this, it would be valuable to monitor cardiovascular parameters during the imaging process, including with task-based or other hemodynamic challenges, or to correlate neuroimaging findings with clinical assessments in population samples who specifically demonstrate cardiovascular alterations. There is some evidence from research in the field of epilepsy that hemispheric location of injury, particularly within the insular cortex, may influence cardiac autonomic responses, such that right insular activation may increase sympathetic tone and result in tachycardia whilst the left insular cortex is more aligned with parasympathetic tone (Citation33,Citation94,Citation95). To our knowledge, this lateralization effect on cardiac autonomic function has not been examined in mTBI populations and would be worthwhile exploring further using advanced MRI techniques. Further to this, the corpus callosum has been identified as one of the most commonly injured white matter structures (Citation61), and given its role in enabling communication between brain hemispheres, injury to this structure has the potential to negatively impact sympathetic and parasympathetic ‘balance’ or interaction (Citation13).
Whilst each neuroimaging modality may have the capacity to identify specific structural or functional abnormalities, it is likely that combining multiple metrics derived from imaging techniques may provide a more complete picture of brain alterations in the mTBI population. For example, no studies in this review using transcranial Doppler ultrasound addressed all three components of cerebrovascular function. Examining these components simultaneously may be necessary to achieve a comprehensive understanding of CBF dynamics (Citation16). Likewise, utilizing a multi-modal approach with different MRI sequences may provide further insight into the pathophysiology of mTBI (Citation35,Citation82). Given the volume and complexity of information generated in this way, machine learning approaches may be required to analyze data in more depth (Citation36). Furthermore, neuroimaging is most informative when considered in the clinical context of a concussion, and should be used as an adjunct to functional assessment findings to better define concussion sub-types, and thus enable more targeted, individualized management (Citation36).
We identified two important gaps in the literature of this review with regards to participant characteristics. Firstly, no studies included children, and fewer studies (n = 9) involved adults over 25 years of age, albeit these studies had large participant sample sizes. Secondly, almost two-thirds of research articles included adolescent and young adult athlete participants who sustained mTBI in sport-related contexts, and there was a lack of research examining adults who have sustained mTBI from causes other than sport. This finding is consistent with the majority of research in the mTBI space. It is important to study homogenous population samples initially in order to identify relationships and correlations, however it should also be recognized that mTBI incidence occurs across the lifespan and that physiological responses and recovery are likely to be different in pediatric, adult, and geriatric populations (and non-athletes) compared to adolescents (Citation81). It is also important to account for biological sex in mTBI research as differences between males and females have been identified with regard to the role of hormones during the menstrual cycle (Citation96) and axon cytoskeletal size and structure (Citation97), and may impact clinical presentation and neuroimaging findings.
Conclusion
This scoping review has provided valuable insight into the complex relationship between cardiovascular autonomic changes and brain pathophysiology following mTBI. Two main research approaches to neuroimaging following mTBI have been identified. More than half the research articles included in this scoping review used transcranial Doppler ultrasound to assess the relationship between cardiovascular parameters and cerebrovascular regulation. The findings of these studies demonstrate the importance that CBF plays in physiological alterations in individuals with mTBI, particularly when compared to persons without mTBI. There is also preliminary evidence that cardiovascular changes may be a consequence of microstructural injury within brain regions responsible for cardiac autonomic function, as visualized using neuroimaging, such as DTI or fMRI. The combination of cardiovascular autonomic assessment and neuroimaging is in a nascent state. Athough it has thus far identified some interesting findings following mTBI, the dual assessment of these measures has several key knowledge gaps, which, when addressed, hold significant promise for future research.
Authors contributions
JT, SH, and MF conceptualized the study and participated in initial study design. JT and SH conducted Title/Abstract and Full Text screening. JT extracted the data, with secondary checking by SH. JT prepared all tables and figures. JT drafted the initial manuscript. All authors reviewed and further contributed the manuscript. JT coordinated manuscript revisions.
Supplemental Material
Download MS Word (34 KB)Disclosure statement
No potential conflict of interest was reported by the author(s).
Supplemental data
Supplemental data for this article can be accessed online at https://doi.org/10.1080/02699052.2023.2211352.
Additional information
Funding
References
- King NS. Mild traumatic brain injury’ and ‘Sport-related concussion’: different languages and mixed messages? Brain Inj. 2019;33(12):1556–63. doi:10.1080/02699052.2019.1655794.
- Cassidy JD, Carroll LJ, Peloso PM, Borg J, von Holst H, Holm L, Kraus J, Coronado V. Incidence, risk factors and prevention of mild traumatic brain injury: results of the WHO collaborating centre task force on mild traumatic brain injury. J Rehabil Med Suppl. 2004;36(0):28–60. doi:10.1080/16501960410023732.
- Kristman VL, Borg J, Godbolt AK, Salmi LR, Cancelliere C, Carroll LJ, Holm LW, Nygren-de Boussard C, Hartvigsen J, Abara U, et al. Methodological issues and research recommendations for prognosis after mild traumatic brain injury: results of the international collaboration on mild traumatic brain injury prognosis. Arch Phys Med Rehabil. 2014;95(3):S265–77. doi:10.1016/j.apmr.2013.04.026.
- Kay T. American congress of rehabilitation medicine, definition of mild traumatic brain injury. J Head Trauma Rehabil. 1993;8(3):86–87. doi:10.1097/00001199-199309000-00010.
- Carroll LJ, Cassidy JD, Holm L, Kraus J, Coronado VG. Methodological issues and research recommendations for mild traumatic brain injury: the WHO collaborating centre task force on mild traumatic brain injury. J Rehabil Med. 2004;36(43):113–25. doi:10.1080/16501960410023877.
- James SL, Bannick MS, Montjoy-Venning WC, Lucchesi LR, Dandona L, Dandona R, Abbasi N, Abdulkader R, Abraha HN, Adsuar JC, et al. Global, regional, and national burden of traumatic brain injury and spinal cord injury, 1990–2016: a systematic analysis for the global burden of disease study 2016. Lancet Neurol. 2019;18(1):56–87. doi:10.1016/S1474-4422(18)30415-0.
- Theadom A, Parag V, Dowell T, Mcpherson K, Starkey N, Barker-Collo S, Jones K, Ameratunga S, Feigin VL. Persistent problems 1 year after mild traumatic brain injury: a longitudinal population study in New Zealand. Br J Gen Pract. 2016;66(642):e16. doi:10.3399/bjgp16X683161.
- Beauchamp F, Boucher V, Neveu X, Ouellet V, Archambault P, Berthelot S, Chauny J-M, de Guise E, Émond M, Frenette J, et al. Post-concussion symptoms in sports-related mild traumatic brain injury compared to non-sports-related mild traumatic brain injury. Can J Emerg Med. 2021;23(2):223–31. doi:10.1007/s43678-020-00060-0.
- McCrea M, Guskiewicz K, Randolph C, Barr WB, Hammeke TA, Marshall SW, Powell MR, Woo Ahn K, Wang Y, Kelly JP. Incidence, clinical course, and predictors of prolonged recovery time following sport-related concussion in high school and college athletes. J Int Neuropsych Soc. 2013;19(1):22–33. doi:10.1017/S1355617712000872.
- Leddy JJ, Kozlowski KF, Fung M, Pendergast DR, Willer B, Willer B, Leddy JJ. Regulatory and autoregulatory physiological dysfunction as a primary characteristic of post concussion syndrome: implications for treatment. NeuroRehabilitation. 2007;22(3):199–205. doi:10.3233/NRE-2007-22306.
- Raab W. Hypertension and tachycardia due to concussion of the brain. Am Heart J. 1949;37(2):237–48. doi:10.1016/0002-8703(49)90565-7.
- Blake TA, McKay CD, Meeuwisse WH, Emery CA. The impact of concussion on cardiac autonomic function: a systematic review. Brain Inj. 2016;30(2):132–45. doi:10.3109/02699052.2015.1093659.
- Hilz MJ, DeFina PA, Anders S, Koehn J, Lang CJ, Pauli E, Flanagan SR, Schwab S, Marthol H. Frequency analysis unveils cardiac autonomic dysfunction after mild traumatic brain injury. J Neurotrauma. 2011;28(9):1727–38. doi:10.1089/neu.2010.1497.
- Pyndiura KL, Di Battista AP, Hutchison MG. A history of concussion is associated with minimal perturbations to heart rate variability in athletes. Brain Inj. 2020;34(10):1416–21. doi:10.1080/02699052.2020.1802661.
- Wright AD, Smirl JD, Bryk K, van Donkelaar P. A prospective transcranial doppler ultrasound-based evaluation of the acute and cumulative effects of sport-related concussion on neurovascular coupling response dynamics. J Neurotrauma. 2017;34(22):3097–106. doi:10.1089/neu.2017.5020.
- Wright AD, Smirl JD, Bryk K, Fraser S, Jakovac M, van Donkelaar P. Sport-related concussion alters indices of dynamic cerebral autoregulation. Front Neurol. 2018;9(MAR):1–11. doi:10.3389/fneur.2018.00196.
- Haider MN, Johnson BD, Horn EC, Leddy JJ, Wilber CG, Reed EL, O’Leary M, Bloomfield A, Decezaro LL, Willer BS. Blunted cardiac parasympathetic activation in student athletes with a remote history of concussion: a pilot study. Front Neurol. 2020;11. doi:10.3389/fneur.2020.547126.
- Hinds A, Leddy J, Freitas M, Czuczman N. The effect of exertion on heart rate and rating of perceived exertion in acutely concussed individuals. J Neurol Neurophysiol. 2016;7(4). doi:10.4172/2155-9562.1000388.
- Pertab JL, Merkley TL, Cramond AJ, Cramond K, Paxton H, Wu T. Concussion and the autonomic nervous system: an introduction to the field and the results of a systematic review. NeuroRehabilitation. 2018;42(4):397–427. doi:10.3233/NRE-172298.
- La Fountaine MF, Hohn AN, Testa AJ, Weir JP. Attenuation of spontaneous baroreceptor sensitivity after concussion. Med Sci Sports Exerc. 2019 Apr;51(4):792–97. doi:10.1249/MSS.0000000000001833.
- Dobson JL, Yarbrough MB, Perez J, Evans K, Buckley T. Sport-related concussion induces transient cardiovascular autonomic dysfunction. Am J Physiol Regul Integr Comp Physiol. 2017;312(4):R575–84. doi:10.1152/ajpregu.00499.2016.
- Balestrini CS, Moir ME, Abbott KC, Klassen SA, Fischer LK, Fraser DD, Shoemaker JK. Autonomic dysregulation in adolescent concussion is sex- and posture-dependent. Clin J Sport Med. 2021;31(3):257–65. doi:10.1097/JSM.0000000000000734.
- Gall B, Parkhouse W, Goodman D. Heart rate variability of recently concussed athletes at rest and exercise. Med Sci Sports Exerc. 2004;36(8):1269–74. doi:10.1249/01.MSS.0000135787.73757.4D.
- Mercier LJ, Batycky J, Campbell C, Schneider K, Smirl J, Debert CT. Autonomic dysfunction in adults following mild traumatic brain injury: a systematic review. NeuroRehabilitation. 2022;50(1):3–32. doi:10.3233/NRE-210243.
- Willie CK, Tzeng YC, Fisher JA, Ainslie PN. Integrative regulation of human brain blood flow. J Physiol. 2014 Mar 1;592(5):841–59. doi:10.1113/jphysiol.2013.268953.
- Tan CO, WP M 3rd, Iverson GL, Taylor JA. Cerebrovascular regulation, exercise, and mild traumatic brain injury. Neurology. 2014;83(18):1665–72. doi:10.1212/WNL.0000000000000944.
- Benarroch E. The central autonomic network: functional organization, dysfunction, and perspective. Mayo Clin Proc. 1993;68(10):988–1001. doi:10.1016/S0025-6196(12)62272-1.
- Roy HA, Green AL. The central autonomic network and regulation of bladder function. Frontiers in Neuroscien. 2019;13(535). doi:10.3389/fnins.2019.00535.
- Wong SW, Massé N, Kimmerly DS, Menon RS, Shoemaker JK. Ventral medial prefrontal cortex and cardiovagal control in conscious humans. Neuroimage. 2007;35(2):698–708. doi:10.1016/j.neuroimage.2006.12.027.
- Riganello F, Karl Larroque S, Ali Bahri M, Heine L, Martial C, Carrière M, Charland-Verville V, Aubinet C, Vanhaudenhuyse A, Chatelle C, et al. A heartbeat away from consciousness: heart rate variability entropy can discriminate disorders of consciousness and is correlated with resting-state fMRI brain connectivity of the central autonomic network. Front Neurol. 2018;9:769.
- Goldstein B, Toweill D, Lai S, Sonnenthal K, Kimberly B. Uncoupling of the autonomic and cardiovascular systems in acute brain injury. Am J Physiol Regul Integr Comp Physiol. 1998;275(4):R1287–92. doi:10.1152/ajpregu.1998.275.4.R1287.
- Ellis MJ, Leddy JJ, Willer B. Physiological, vestibulo-ocular and cervicogenic post-concussion disorders: an evidence-based classification system with directions for treatment. Brain Inj. 2015;29(2):238–48. doi:10.3109/02699052.2014.965207.
- La Fountaine MF. An anatomical and physiological basis for the cardiovascular autonomic nervous system consequences of sport-related brain injury. Int J Psychophysiol. 2018 Oct 1;132:155–66. doi:10.1016/j.ijpsycho.2017.11.016.
- Churchill NW, Hutchison MG, Richards D, Leung G, Graham SJ, Schweizer TA. The first week after concussion: blood flow, brain function and white matter microstructure. Neuroimage Clin. 2017;14:480–89. doi:10.1016/j.nicl.2017.02.015.
- Eierud C, Craddock RC, Fletcher S, Aulakh M, King-Casas B, Kuehl D, LaConte SM. Neuroimaging after mild traumatic brain injury: review and meta-analysis. Neuroimage Clin. 2014;4:283–94. doi:10.1016/j.nicl.2013.12.009.
- Chong CD, Schwedt TJ. Research imaging of brain structure and function after concussion. Headache. 2018;58(6):827–35. doi:10.1111/head.13269.
- Koerte IK, Hufschmidt J, Muehlmann M, Lin AP, Shenton ME. Advanced neuroimaging of mild traumatic brain injury. In: Laskowitz D, Grant G, editors. Translational research in traumatic brain injury. Boca Raton, Florida: CRC Press/Taylor and Francis Group; 2016. pp. 277–97.
- Wiegand TLT, Sollmann N, Bonke EM, Umeasalugo KE, Sobolewski KR, Plesnila N, Shenton ME, Lin AP, Koerte IK. Translational neuroimaging in mild traumatic brain injury. J Neurosci Res. 2021;100(5):1–17. doi:10.1002/jnr.24840.
- Raichle ME. The brain’s default mode network. Annu Rev Neurosci. 2015;38(1):433–47. doi:10.1146/annurev-neuro-071013-014030.
- Purkayastha S, Sorond F. Transcranial doppler ultrasound: technique and application. Semin Neurol. 2012;32(4):411–20. doi:10.1055/s-0032-1331812.
- Wang Y, Bartels HM, Nelson LD. A systematic review of ASL perfusion MRI in mild TBI. Neuropsychol Rev. 2020;33(1):160–91. doi:10.1007/s11065-020-09451-7.
- Meier TB, Bellgowan PSF, Singh R, Kuplicki R, Polanski DW, Mayer AR. Recovery of cerebral blood flow following sports-related concussion. JAMA Neurol. 2015;72(5):530–38. doi:10.1001/jamaneurol.2014.4778.
- Barlow KM, Marcil LD, Dewey D, Carlson HL, Macmaster FP, Brooks BL, Lebel RM. Cerebral perfusion changes in post-concussion syndrome. J Neurotrauma. 2017;1004(5):996–1004. doi:10.1089/neu.2016.4634.
- Churchill NW, Hutchison MG, Graham SJ, Schweizer TA. Mapping brain recovery after concussion: from acute injury to 1 year after medical clearance. Neurology. 2019;93(21):e1980–92. doi:10.1212/WNL.0000000000008523.
- Hellewell SC, Welton T, Pearce AJ, Maller JJ, Grieve SM. Diffusion MRI as a complementary assessment to cognition, emotion, and motor dysfunction after sports-related concussion: a systematic review and critical appraisal of the literature. Brain Imaging Behav. 2021;15(3):1685–704. doi:10.1007/s11682-020-00336-0.
- Purkayastha S, Williams B, Murphy M, Lyng S, Sabo T, Bell KR. Reduced heart rate variability and lower cerebral blood flow associated with poor cognition during recovery following concussion. Auton Neurosci. 2019;220:102548. doi:10.1016/j.autneu.2019.04.004.
- Tricco AC, Lillie E, Zarin W, O’Brien KK, Colquhoun H, Levac D, Moher D, Peters MDJ, Horsley T, Weeks L, et al. PRISMA extension for scoping reviews (PRISMA-ScR): checklist and explanation. Ann Intern Med. 2018;169(7):467–73. doi:10.7326/M18-0850.
- Levac D, Colquhoun H, O’Brien KK. Scoping studies: advancing the methodology. Implement Sci. 2010;5(1):69. doi:10.1186/1748-5908-5-69.
- Peters MDJ, Marnie C, Tricco AC, Pollock D, Munn Z, Alexander L, McInerney P, Godfrey CM, Khalil H. Updated methodological guidance for the conduct of scoping reviews. JBI Evid Implement. 2021;19(1):3–10. doi:10.1097/XEB.0000000000000277.
- McCrory P, Meeuwisse W, Dvorak J, Aubry M, Bailes J, Broglio S, Cantu RC, Cassidy D, Echemendia RJ, Castellani RJ, et al. Consensus statement on concussion in sport-the 5th international conference on concussion in sport held in Berlin, october 2016. Br J Sports Med. 2017;51(11):838–47. doi:10.1136/bjsports-2017-097699.
- Ruff RM, Iverson GL, Barth JT, Bush SS, Broshek DK. Recommendations for diagnosing a mild traumatic brain injury: a national academy of neuropsychology education paper. Arch Clin Neuropsych. 2009;24(1):3–10. doi:10.1093/arclin/acp006.
- Sharma A, Weintraub A. Neuroimaging. In: Kreutzer J, DeLuca J Caplan B, editors. Encyclopedia of clinical neuropsychology. Cham: Springer International Publishing; 2016. pp. 1–5.
- Chai KEK, Lines RLJ, Gucciardi DF, Ng L. Research screener: a machine learning tool to semi-automate abstract screening for systematic reviews. Syst Rev. 2021;10(1):1–13. doi:10.1186/s13643-021-01635-3.
- Middleton K, Krabak BJ, Coppel DB. The influence of pediatric autonomic dysfunction on recovery after concussion. Clin J Sport Med. 2010;20(6):491–92. doi:10.1097/JSM.0b013e3181fac088.
- Jünger EC, Newell DW, Grant GA, Avellino AM, Ghatan S, Douville CM, Lam AM, Aaslid R, Winn HR. Cerebral autoregulation following minor head injury. J Neurosurg. 1997;86(3):425–32. doi:10.3171/jns.1997.86.3.0425.
- Solbakk AK, Reinvang I, Svebak S, Nielsen CS, Sundet K. Attention to affective pictures in closed head injury: event-related brain potentials and cardiac responses. J Clin Exp Neuropsychol. 2005;27(2):205–23. doi:10.1080/13803390490515739.
- Strebel S, Lam AM, Matta BF, Newell DW. Impaired cerebral autoregulation after mild brain injury. Surg Neurol. 1997;47(2):128–31. doi:10.1016/S0090-3019(96)00459-4.
- Kumazaki A, Ogoh S, Hirasawa A, Sakai S, Hirose N. Cognitive function and dynamic cerebral blood flow regulation in multiple concussions. Asian J Sports Med. 2018;9(1):e67660. doi:10.5812/asjsm.67660.
- Si B, Dumkrieger G, Wu T, Zafonte R, Valadka AB, Okonkwo DO, Manley GT, Wang L, Dodick DW, Schwedt TJ, Li J, et al. Sub-classifying patients with mild traumatic brain injury: a clustering approach based on baseline clinical characteristics and 90-day and 180-day outcomes. PLos One. 2018;13(7):e0198741. doi:10.1371/journal.pone.0198741.
- Schwed AC, Boggs MM, Watanabe D, Plurad DS, Putnam BA, Kim DY. Admission variables associated with a favorable outcome after mild traumatic brain injury. Am Surg. 2016;82(10):898–902. doi:10.1177/000313481608201008.
- Polak P, Leddy JJ, Dwyer MG, Willer B, Zivadinov R. Diffusion tensor imaging alterations in patients with postconcussion syndrome undergoing exercise treatment: a pilot longitudinal study. J Head Trauma Rehabil. 2015;30(2):E32–42. doi:10.1097/HTR.0000000000000037.
- Leddy JJ, Cox JL, Baker JG, Wack DS, Pendergast DR, Zivadinov R, Willer B. Exercise treatment for postconcussion syndrome: a pilot study of changes in functional magnetic resonance imaging activation, physiology, and symptoms. J Head Trauma Rehabil. 2013;28(4):241–49. doi:10.1097/HTR.0b013e31826da964.
- Kostoglou K, Wright AD, Smirl JD, Bryk K, van Donkelaar P, Mitsis GD Dynamic cerebral autoregulation in young athletes following concussion. IEEE Engineering in Medicine and Biology Society Annual International Conference; Orlando, Florida, USA. 2016; 2016(101763872). 696–99.
- Imhoff S, Malenfant S, Nadreau E, Poirier P, Bailey DM, Brassard P. Uncoupling between cerebral perfusion and oxygenation during incremental exercise in an athlete with postconcussion syndrome: a case report. Physiol Rep. 2017;5(2):e13131. doi:10.14814/phy2.13131.
- Clausen M, Pendergast DR, Willer B, Leddy J. Cerebral blood flow during treadmill exercise is a marker of physiological postconcussion syndrome in female athletes. J Head Trauma Rehabil. 2016;31(3):215–24. doi:10.1097/HTR.0000000000000145.
- Howell DR, Hunt DL, Aaron SE, Hamner JW, WP M 3rd, Tan CO. Association of hemodynamic and cerebrovascular responses to exercise with symptom severity in adolescents and young adults with concussion. Neurology. 2021;97(22):e2204–12. doi:10.1212/WNL.0000000000012929.
- Piantino J, Schwartz DL, Luther M, Newgard C, Silbert L, Raskind M, Pagulayan K, Kleinhans N, Iliff J, Peskind E. Link between mild traumatic brain injury, Poor sleep, and magnetic resonance imaging: visible perivascular spaces in veterans. J Neurotrauma. 2021;38(17):2391–99. doi:10.1089/neu.2020.7447.
- Aaron SE, Hamner JW, Ozturk ED, Hunt DL, Iaccarino MA, Meehan WP, Howell DR, Tan CO. Cerebrovascular neuroprotection after acute concussion in adolescents. Ann Neurol. 2021 Jul 1;90(1):43–51. doi:10.1002/ana.26082.
- Ding K, Tarumi T, Tomoto T, McColloster M, Le T, Dieppa M, Diaz-Arrastia R, Bell K, Madden C, Cullum CM, et al. Impaired cerebral blood flow regulation in chronic traumatic brain injury. Brain Res. 2020;1743:146924.
- Kisser JE, Allen AJ, Katzel LI, Wendell CR, Siegel EL, Lefkowitz D, Waldstein SR. Relations of blood pressure and head injury to regional cerebral blood flow. J Neurol Sci. 2016;365:9–14. doi:10.1016/j.jns.2016.03.033.
- Moir ME, Balestrini CS, Abbott KC, Klassen SA, Fischer LK, Fraser DD, SHOEMAKER JK. An investigation of dynamic cerebral autoregulation in adolescent concussion. Med Sci Sports Exerc. 2018;50(11):2192–99. doi:10.1249/MSS.0000000000001695.
- Neary JP, Dude CM, Singh J, Len TK, Bhambhani YN. Pre-frontal cortex oxygenation changes during aerobic exercise in elite athletes experiencing sport-related concussion. Front Hum Neurosci. 2020;14:35. doi:10.3389/fnhum.2020.00035.
- Purkayastha S, Sorond FA, Lyng S, Frantz J, Murphy MN, Hynan LS, Sabo T, Bell KR. Impaired cerebral vasoreactivity despite symptom resolution in sports-related concussion. J Neurotrauma. 2019;36(16):2385–90. doi:10.1089/neu.2018.5861.
- Slobounov SM, Gay M, Zhang K, Johnson B, Pennell D, Sebastianelli W, Horovitz S, Hallett M. Alteration of brain functional network at rest and in response to YMCA physical stress test in concussed athletes: RsFMRI study. Neuroimage. 2011;55(4):1716–27. doi:10.1016/j.neuroimage.2011.01.024.
- Worley ML, O’Leary MC, Sackett JR, Schlader ZJ, Willer B, Leddy JJ, Johnson BD. Preliminary evidence of orthostatic intolerance and altered cerebral vascular control following sport-related concussion. Front Neurol. 2021;12:620757. doi:10.3389/fneur.2021.620757.
- Zhang K, Johnson B, Gay M, Horovitz SG, Hallett M, Sebastianelli W, Slobounov S. Default mode network in concussed individuals in response to the YMCA physical stress test. J Neurotrauma. 2012;29(5):756–65. doi:10.1089/neu.2011.2125.
- Shannon CA, Pike KL, Dejonge SR, Nagelkirk PR, Del Pozzi AT. Head trauma not associated with long term effects on autonomic function. Int J Exerc Sci. 2021;14(3):779–90.
- Albalawi T, Hamner JW, Lapointe M, Meehan WP, Tan CO. The relationship between cerebral vasoreactivity and post-concussive symptom severity. J Neurotrauma. 2017;34(19):2700–05. doi:10.1089/neu.2017.5060.
- Wright AD, Smirl JD, Bryk K, van Donkelaar P. Systolic and diastolic regulation of the cerebral pressure-flow relationship differentially affected by acute sport-related concussion. Acta Neurochir Suppl. 2018;126:303–08.
- Arain M, Haque M, Johal L, Mathur P, Nel W, Rais A. Maturation of the adolescent brain. Neuropsychiatr Dis Treat. 2013;9:449–61. doi:10.2147/NDT.S39776.
- Snyder AR, Giza CC. The future of concussion. Semin Pediatr Neurol. 2019;30:128–37. doi:10.1016/j.spen.2019.03.018.
- Bigler ED, Abildskov TJ, Goodrich-Hunsaker NJ, Black G, Christensen ZP, Huff T, Wood DMG, Hesselink JR, Wilde EA, Max JE. Structural neuroimaging findings in mild traumatic brain injury. Sports Med Arthrosc Rev. 2016;24(3):e42–52. doi:10.1097/JSA.0000000000000119.
- Reed EL, O’Leary MC, Sackett JR, Schlader ZJ, Leddy JJ, Johnson BD. Cardiovascular responses to the cold pressor test are correlated with resting cerebral blood flow in healthy controls and concussed athletes. Faseb J. 2019;33(S1). doi:10.1096/fasebj.2019.33.1_supplement.838.21.
- Bickart K, Sheridan CA, Thibeault CM, Hamilton R, LeVangie J, Giza C, Choe M. Heart rate variability interacts with recovery of resting-state connectivity in amygdala circuitry and the persistence of symptoms after sport-related concussion. Neurology. 2020;95(20 Supplement 1):S15–6. doi:10.1212/01.wnl.0000720016.51384.d0.
- Ibarra LP. Quantifying post-concussion balance deficits with dual tasks, heart rate variability, muscle synergies, and cortical activity, to identify a novel concussion biomarker. State University of New York at Stony Brook: Proquest; 2019.
- Gay M The effects of performing the YMCA bike protocol on general brain function in athletes with and without mild traumatic brain injury. Dissertation Abstracts International: Section B: The Sciences and Engineering. 2016;76(10–B(E)).
- Coenen J, Van Den Bongard F, Delling AC, Reinsberger C. Functional connectivity within the default mode network in response to exercise during return-to-sport following concussion. Neurology. 2022;98(1 (Supplement 1):S18.1–S18. doi:10.1212/01.wnl.0000801904.27836.ef.
- Charron J, Soto-Catalan C, Marcotte L’Heureux V, Comtois AS. Unclear outcomes of heart rate variability following a concussion: a systematic review. Brain Inj. 2021;35(9):987–1000. doi:10.1080/02699052.2021.1891459.
- Gordan R, Gwathmey JK, Xie LH. Autonomic and endocrine control of cardiovascular function. World J Cardiol. 2015;7(4):204. doi:10.4330/wjc.v7.i4.204.
- McMillan TM, Teasdale GM, Weir CJ, Stewart E. Death after head injury: the 13 year outcome of a case control study. J Neurol Neurosurg Psychiatry. 2011;82(8):931–35. doi:10.1136/jnnp.2010.222232.
- Kamins J, Bigler E, Covassin T, Henry L, Kemp S, Leddy JJ, Mayer A, McCrea M, Prins M, Schneider KJ, et al. What is the physiological time to recovery after concussion? A systematic review. Br J Sports Med. 2017;51(12):935–40. doi:10.1136/bjsports-2016-097464.
- Leddy JJ, Haider MN, Ellis MJ, Willer BS. Exercise is medicine for concussion. Curr Sports Med Rep. 2018;17(8):262–70. doi:10.1249/JSR.0000000000000505.
- Giza CC, Hovda D. The new neurometabolic cascade of concussion. Neurosurgery. 2014;75(4):S24–33. doi:10.1227/NEU.0000000000000505.
- Nagai M, Hoshide S, Kario K. The insular cortex and cardiovascular system: a new insight into the brain-heart axis. J Am Soc Hypertens. 2010 Jul 1;4(4):174–82. doi:10.1016/j.jash.2010.05.001.
- Oppenheimer SM, Gelb A, Girvin JP, Hachinski VC. Cardiovascular effects of human insular cortex stimulation. Neurology. 1992;42(9):1727–32. doi:10.1212/WNL.42.9.1727.
- Wunderle K, Hoeger KM, Wasserman E, Bazarian JJ. Menstrual phase as predictor of outcome after mild traumatic brain injury in women. J Head Trauma Rehabil. 2014;29(5):E1–8.
- Dollé JP, Jaye A, Anderson SA, Ahmadzadeh H, Shenoy VB, Smith DH. Newfound sex differences in axonal structure underlie differential outcomes from in vitro traumatic axonal injury. Exp Neurol. 2018;300:121–34.
Appendix A:
PRISMA-ScR Checklist
Appendix B:
Example Medline electronic search strategy
Appendix C:
Citations for records excluded following full text review with reasons for exclusion
1. Akira K, Ogoh S, Hirasawa A, Sakai S, Hirose N. Cognitive function and dynamic cerebral blood flow regulation in multiple concussions. AsianJ Sports Med. 2018;9(1):e67660.
Duplicate of article listed as Kumazaki, 2018
2. Bickart K, Sheridan CA, Thibeault CM, Hamilton R, LeVangie J, Giza C, et al. Heart rate variability interacts with recovery of resting-state connectivity in amygdala circuitry and the persistence of symptoms after sport-related concussion. Neurology. 2020;95(20 (Supplement 1)):S15–6.
Not peer reviewed (Abstract only)
3. Coenen J, Van Den Bongard F, Delling AC, Reinsberger C. Functional connectivity within the default mode network in response to exercise during return-to-sport following concussion. Neurology. 2022;98(1 (Supplement 1)).
Not peer reviewed (Abstract only)
4. Danielli E., DeMatteo C., Hall G.B., Noseworthy M.D. A Review of MRI and Exercise Treatment for Improved Concussion Diagnosis and Recovery. CritRevBiomedEng. 2020;48(5):261–83.
Narrative review
5. DeWitt DS, Prough DS. Cerebral Blood Flow and Blood Pressure: Dependent or Codependent? Crit Care Med [Internet]. 2019;47(7):1007–9.
Narrative review (editorial)
6. Emery C.A., Smirl J. Early targeted heart rate aerobic exercise for sport-related concussion. Lancet Child Adolesc Health. 2021;5(11):769–71.
Not peer reviewed (Comment only)
7. Gay M. The effects of performing the YMCA bike protocol on general brain function in athletes with and without mild traumatic brain injury. Dissertation Abstracts International: Section B: The Sciences and Engineering. 2016;76(10-B(E)).
Not peer reviewed (Thesis)
8. Gibson TJ, Johnson CB, Murrell JC, Mitchinson SL, Stafford KJ, Mellor DJ. Electroencephalographic responses to concussive non-penetrativecaptive-bolt stunning in halothane-anaesthetised calves. N Z Vet J. 2009;57(2):90–5.
Did not meet criteria for mTBI (not external force)
9. Gibson TJ, Johnson CB, Murrell JC, Mitchinson SL, Stafford KJ, Mellor DJ. Amelioration of electroencephalographic responses to slaughter by non- penetrative captive-bolt stunning after ventral-neck incision in halothane-anaesthetised calves. N Z Vet J. 2009;57(2):96–101.
Did not meet criteria for mTBI (not external force)
10. Ibarra LP. Quantifying Post-Concussion Balance Deficits with Dual Tasks, Heart Rate Variability, Muscle Synergies, and Cortical Activity, to Identify a Novel Concussion Biomarker. State University of New York at Stony Brook; 2019.
Not peer reviewed (Thesis)
11. Jarosz BS. Postural orthostatic tachycardia syndrome (POTS): Its relevance, and importance, in the management of sport related concussion. Chiropractic Journal of Australia. 2021;48(1):1–4.
Narrative review (editorial)
12. Knox A, Falvo M, Favre M, Serrador J. Blood Pressure and Cerebral Blood Flow Following Concussion: Implications for Diagnosis. FASEB JOURNAL. 2017;31.
Not peer reviewed (Abstract only)
13. Kuhne CA, Homann M, Ose C, Waydhas C, Nast-Kolb D, Ruchholtz S. Emergency room patients. Der Schockraumpatient Einschatzung ernster Schadelverletzungen anhand der GCS bei vermeintlich leichtem und mittelschwerem Schadel-Hirn-Trauma. 2003;106(5):380–6.
Publication in language other than English
14. Lagos LM. A Case Report on Heart Rate Variability Biofeedback and Postconcussion Syndrome: Healing the Autonomic Nervous System. Biofeedback. 2021;49(4):86–8.
Narrative review
15. Lagos L, Bottiglieri T, Vaschillo B, Vaschillo E. Heart Rate Variability Biofeedback for Postconcussion Syndrome: Implications for Treatment. Biofeedback. 2012 Dec 1;40(4):150–3.
Full text not available
16. Moir ME. Impaired Dynamic Cerebrovascular Autoregulation in Adolescent Concussion. ProQuest Dissertations &Theses Global; 2017.
Not peer reviewed (Thesis)
17. Navarro JC, Pillai S, Cherian L, Garcia R, Grill RJ, Robertson CS. Histopathological and behavioral effects of immediate and delayed hemorrhagic shock after mild traumatic brain injury in rats. J Neurotrauma [Internet]. 2012;29(2):322–34.
Did not meet criteria for mTBI (not external force)
18. Prat R, Markiv V, Dujovny M, Misra M. Failure of cerebral autoregulation in an experimental diffuse brain injury model. Acta Neurochir Suppl. 1998;71(100962752):123–6.
Full text not available
19. Reed EL, O’Leary MC, Sackett JR, Schlader ZJ, Leddy JJ, Johnson BD. Cardiovascular Responses to the Cold Pressor Test are Correlated with Resting Cerebral Blood Flow in Healthy Controls and Concussed Athletes. FASEB JOURNAL. 2019;33.
Not peer reviewed (Abstract only)
20. Shannon CA. Effects of head trauma on heart rate, blood pressure, cerebral blood flow and orthostatic tolerance. Ball State University, Indiana;2020.
Not peer reviewed (Thesis)
21. Thompson JWG, Hagedorn D. Multimodal Analysis: New Approaches to the Concussion Conundrum. J Clin Sport Psychol [Internet]. 2012;6(1):22–46.
Narrative review
22. Wang R, Poublanc J, Crawley AP, Sobczyk O, Kneepkens S, Mcketton L, et al. Cerebrovascular reactivity changes in acute concussion: a controlled cohort study. Quant Imaging Med Surg. 2021;11(11):4530.
Did not include a cardiovascular parameter
23. Wang Y, Nencka AS, Meier TB, Guskiewicz K, Mihalik JP, Alison Brooks M, et al. Cerebral blood flow in acute concussion: preliminary ASL findings from the NCAA-DoD CARE consortium. Brain Imaging Behav. 2019;13(5):1375–85.
Did not include a cardiovascular parameter
24. Ward JW, Clark SL. The electroencephalogram in experimental concussion and related conditions. J Neurophysiol [Internet]. 1948;11:59–74.
Did not meet criteria for mTBI (moderate-severe TBI)
25. Wright AD, Smirl JD, Bryk K, Fraser S, Jakovac M, van Donkelaar P. Cerebral Autoregulation Is Disrupted Following a Season of Contact Sports Participation. Front Neurol [Internet]. 2018;9(101546899):868.
Did not meet criteria for mTBI (sub-concussive injury).