ABSTRACT
Objective
Recent research suggests that patients with neurological disorders without overt seizures may also experience accelerated long-term forgetting (ALF). This term describes unimpaired learning and memory performance after standard retention intervals, but an excessive rate of forgetting over delays of days or weeks. The objective of this retrospective study was to investigate ALF in patients with an acquired brain injury (ABI) and to associate memory performance with executive functions.
Methods
Verbal memory performance (short-term recall, 30-min recall, 1-week recall) was assessed in 34 adult patients with ABI and compared to a healthy control group (n = 54) using an auditory word learning and memory test.
Results
Repeated measure analysis showed significant effects of time and group as well as interaction effects between time and group regarding recall and recognition performance. Patients with ABI had a significantly impaired 1-week recall and recognition performance compared to the healthy control group. Correlations between recall performance and executive functions were nonsignificant.
Discussion
Our results demonstrate that non-epileptic patients with ABI, especially patients with frontal and fronto-temporal lesions, are prone to ALF. Additionally, our data support the assumption that ALF results from a consolidation impairment since verbal recall and recognition were impaired in patients with ABI.
Introduction
Episodic memory enables us to learn, store and recall information about unique personal experiences. Acquired brain injury (ABI) (such as traumatic brain injury (TBI), stroke or encephalitis) may lead to epilepsy and can impair episodic memory performance, which may impact participation in daily life (Citation1). Deficits in episodic memory are detected using standardized memory tests in which patients learn information that they have to freely recall after 20–30 minutes. However, there are patients with a normal recall performance in standardized tests who have accelerated long-term forgetting (ALF) over time. These patients show a reduced memory recall of newly learned information over extended delays (Citation2) and experience memory impairments in daily life that are not detected by standardized memory assessment.
ALF has been consistently reported in patients with temporal lobe epilepsy (TLE) (Citation3) and until recently it was believed to be an epilepsy-specific impairment, caused by seizures. A pioneering review investigating long-term memory in other patient groups did not find convincing evidence for ALF in groups of patients without epilepsy (Citation4). However, most of the studies included in that review investigated ALF by using visual recognition paradigms. This could have led to an underestimation of forgetting since forgetting is more pronounced in tests of recall than recognition (Citation5). Additionally, recent evidence has shown that patients without overt seizures and with normal episodic memory performance in standardized episodic memory tests can have ALF; for example, patients with severe pediatric TBI (Citation6), stroke (Citation7,Citation8), and pre-symptomatic Alzheimer disease (Citation9). These results imply that ALF may also exist in patients with neurological disorders without overt seizures, indicating that ALF is a marker of non-disorder-specific memory disruption (Citation10).
It is not known whether ALF is caused by structural damage, functional changes or both (Citation10). In patients with TLE, structural brain abnormalities within the mesial temporal lobe as well as epileptiform discharges are assumed to cause ALF (Citation11), although study findings regarding the association between hippocampal volume and ALF in epilepsy patients are controversial (Citation12–15). To date, few studies have investigated structural correlates of ALF in non-epilepsy patients, without identifying specific lesion characteristics associated with ALF (Citation6,Citation8).
The cognitive mechanisms underlying ALF also remain unclear (Citation16). A recent review of studies on ALF in patients with various types of epilepsy (TLE, transient epileptic amnesia, generalized and extratemporal epilepsy) concluded that ALF is a result of impaired memory consolidation (Citation10) due to deficient hippocampal-frontal transformation (Citation17). However, other studies have found evidence that only free recall after 1 week was impaired, whereas recognition performance was comparable to a control group (Citation7). Since recognition tests demand less cognitive effort than free recall tests, cued recall recognition tests could be less sensitive to subtle reductions in memory performance over 1 week (Citation4). Alternatively, reduced free recall after 1 week in patients with normal cued recognition performance suggests a retrieval impairment rather than a consolidation problem. In this case, ALF would result from an impairment of the retrieval process and could thus be associated with executive functions (Citation10,Citation18). There is evidence that executive functions influence 30 min recall, as it was indicated by Hill and colleagues (Citation19) who reported that executive functions accounted for about 30% of long-term memory recall 30-min after learning. So far, only few studies have investigated the association between executive functions and delayed memory performance, with contradicting findings. Although there is evidence for a positive association between working memory and delayed memory performance after 1 week in children with genetic generalized epilepsy (GGE (Citation20)), other studies did not find an association between executive functions and delayed memory performance in children with TLE (Citation21) or adults with focal epilepsies (Citation22).
The objective of this retrospective study was to investigate whether patients with ABI – who often experience memory impairments (Citation23) – show ALF regarding recall and recognition performance and whether executive functions are related to delayed verbal memory recall. Second, we explored lesion patterns in subgroups of patients after ABI to investigate whether there is an association between lesions and the occurrence of ALF. Based on the literature, we hypothesized that, in contrast to healthy controls, patients with ABI show an impaired verbal recall and recognition performance after 1 week and we expected recall to be associated with executive dysfunctions.
Methods
The study used data from 2 projects approved by the Faculty of Humanities’ Ethics Committee at the University of Bern, Switzerland (NR 2018-12 -00,005, regarding data from healthy control participants) as well as by the regional ethics board of Berne (KEK BE 2021–01195, regarding retrospective data from patients). Both projects were carried out in accordance with the Code of Ethics of the World Medical Association (Declaration of Helsinki). Healthy controls provided written informed consent; all patients signed a general consent during their stay in the hospital.
Participants
This retrospective study included data on German-speaking in-patients with ABI admitted to the acute neuro-rehabilitation unit in the University Hospital, Bern, Switzerland. Inclusion criteria were: an existing general consent, an average verbal learning and recall performance in a standardized memory test and results of verbal recall testing 1 week after learning. Overall, 532 in-patients were treated between December 2018 and May 2021 at the acute neuro-rehabilitation unit. A subgroup of patients (n = 124) were screened for eligibility; of this group, 34 patients were included. Reasons for exclusion were: aphasia (n = 38), memory impairment in standardized testing (n = 38), other reasons (n = 14; including age >70 years (n = 1), foreign language (n = 4), missed testing (n = 9)). illustrates a CONSORT flow chart and shows the included and excluded patients.
As illustrated in , we included 34 patients with ABI with different etiologies (TBI (n = 10), stroke (n = 14, including eight patients with ischemia and six patients with a hemorrhage), neurosurgical interventions with resection of brain tissue (intraaxial and extraaxial tumors, vascular malformations and epilepsy surgery, n = 5), infectious and autoimmune encephalitis and meningoencephalitis (n = 4), hypoxic encephalopathy after cardiac arrest (n = 1)). We concatenated patients with a neurosurgical intervention, encephalitis and hypoxic encephalopathy into the group other diagnosis (n = 10). No patient had epileptic seizures between ABI and neuropsychological testing. However, four patients had confirmed initial seizures and two patients had suspected initial seizures at the incidence. All of these six patients as well as one patient after epilepsy surgery were on a mono-antiepileptic medication during their stay in hospital (n = 7). Using data from the initial MRI/CT scan report, we grouped the patients according to their lesion site as follows: frontal and temporal lesions (n = 11); frontal + lesions (including patients with frontal and other lesions except temporal lesions, n = 11); temporal + lesions (including patients with temporal and other lesions except frontal lesions, n = 4); other lesions (including patients with subcortical or cerebellar lesions, n = 5), no lesions, n = 3) and laterality (right (n = 12), left (n = 8), bilateral (n = 11), no laterality (when the MRI scan was normal, n = 3)).
Table 1. Overview of demographic and clinical characteristics of patients included in the study.
Data from our healthy control group were collected from an ongoing standardization study of delayed memory performance after 1 week. Between January 2020 and June 2022, data on 220 healthy controls were acquired. As illustrated in , we included 54 controls matched with the patients for age, education and sex, and who did not show an elevated score in our screening for depression (DESC (Citation24)). Furthermore, patients and healthy controls had a normal verbal learning performance (sum score of all learning trials) as well as a normal memory recall 30 minutes after learning (standardized z-score ≥ −1.5). In accordance with recent research ((Citation25,Citation26), suggesting that neuropsychological impairment should be defined as a z-score < −1.5 SD below the mean, we applied the same criteria in our study.
Table 2. Overview of clinical data in healthy controls and patients with acquired brain injury (ABI).
Procedure
Data on patients were collected during their in-patient stay in our rehabilitation unit. If patients showed a normal verbal learning and recall performance after 30 minutes (z-score ≥−1.5), unannounced free recall and recognition testing was repeated after a mean delay of 6.87 days (SD = 0.84, range 4–8 days). Attentional and executive performance was measured at the same time as initial memory testing.
Healthy controls in the age range of 18–77 years were recruited by flyers and word-of-mouth advertising. Testing was conducted by trained psychology students and research assistants, supervised by a board-certified clinical neuropsychologist (M.S.). Inclusion criteria for healthy controls were: a normal result of screening for depression (less than 12 points on the DESC-V scale (Citation25)), mother tongue German, no previous psychological or neurological diagnosis, and no regular intake of any medication that could affect cognition. Testing took place between January 2020 and June 2022, in a quiet room in the hospital or at the home of the participant. There were 2 time-points (T1 and T2) separated by 1 week (mean delay: 7 days (SD = 0.18), range 6–7 days). Participants were informed that the study focused on memory, but they were not aware that memory testing would be repeated after a week. When patients asked what the second meeting entailed they were informed that feedback would be given and questionnaires collected. At T1, comprehensive neuropsychological testing was performed, including learning, short-term and 30-min free recall as well as recognition of verbal material; assessment duration was approximately 90 minutes. At T2, 1-week recall and recognition of verbal material was tested again. Furthermore, brief feedback about the neuropsychological results from T1 was given. Assessment duration was approximately 20 minutes. For 3 participants, T2 took place over the phone.
Material
Verbal learning and memory performance were tested using a Swiss adaptation of the Rey Auditory Verbal Learning Test (Auditory Verbal Learning and Memory Test, AVLGT (Citation27)). The AVLGT is a word learning test consisting of 15 unrelated words (list A) which were learned in 5 consecutive learning trials, each followed by an immediate free recall. This learning phase was followed by 1 learning trial and immediate recall of an interference list (list B) with 15 new words. Directly after recall of interference list B, participants had to freely recall the words from list A (short-term recall). Approximately 30 minutes after learning, participants were again asked to recall the words from list A (30-min recall). Free recall was followed up by recognition, where participants have to identify the words from list A out of 30 distractors (15 words from the interference list B and 15 new, semantically or phonologically similar words). For the purposes of the present study, 1 week after learning, participants were asked to freely recall the words from list A (1-week recall). Additionally, we repeated the same recognition task: the participants had to identify the 15 words from list A (with the same distractor words from list B and the 15 new words).
The following dependent variables were used in our analyses: verbal learning (sum of the learning runs 1–5, L1–L5), interference recall, retroactive interference (recall learning trial 5 – short-term recall), short-term recall, 30-min recall and 1-week recall, 30-min recognition (correctly recognized words (hits) minus false-positively (fp) recognized words, hits − fp 30 minutes after learning) and 1-week recognition (hits − fp 1 week after learning). We also calculated percentage recall loss (100*(30-min recall − 1-week recall/30-min recall) as well as percentage recognition loss (100*(30-min recognition − 1-week recognition/30-min recognition)).
Besides verbal learning and memory, measures of executive functioning (verbal working memory and verbal fluency) were obtained. Verbal working memory performance was assessed using the backward digit recall task of the Revised Wechsler Memory Scale (WMS-R (Citation28)). Participants had to repeat immediately after hearing the numbers them in the reverse order. For our analysis, we used the number of correct trials. Verbal fluency measures the ability to produce fluent speech and is linked to cognitive flexibility, organization of thought and updating (Citation30,Citation29). Verbal fluency was measured using the phonemic letter fluency task ‘S-Words’ (Citation27), using a Swiss adaptation of the Controlled Oral Word Association Tests (30), in which as many words beginning with the letter s as possible had to be generated within 3 minutes. We included the number of correct words generated.
Statistical analysis
Statistical analyses used SPSS version 28.0 (IBM Corp., Armonk, NY, U.S.A.). For the analysis of verbal memory performance, we used raw scores (because so far there are no standardized values for recall and recognition performance after 1 week). Raw scores of executive tests were transformed into age- and education-corrected z-scores and were used for these tests. Before running our analysis, we analyzed group differences regarding demographics (age, sex and number of years of education). If the assumption of sphericity was violated, we report the Huynh-Feldt corrected degrees of freedom. In correlation analyses, we controlled for multiple comparisons using Bonferroni corrected p-values.
The first step was to compare verbal learning and memory performance between all patients with ABI (n = 34) and healthy controls (n = 54). We used independent t-tests to compare verbal learning, interference recall, recall loss and recognition loss. Additionally, we used repeated measures ANOVAs to investigate the influence of group on memory performance over time (free recall and recognition). We also analyzed group differences in executive functions using independent t-tests and computed group-specific Pearson correlations between verbal recall and executive functions as well as verbal recall and interference recall/retroactive recall.
In the second step, due to the heterogeneity in our overall patient sample with ABI, we compared verbal memory performance between the different patient subgroups (TBI (n = 10) versus stroke (n = 14) versus ‘other diagnosis’ (n = 10)) to our healthy controls (n = 54). Since patient subgroups differed significantly regarding age, we controlled for age in these analyses, using ANCOVAs (to compare verbal learning, interference recall, recall loss and recognition loss) and repeated measures ANCOVAs (to compare recall and recognition performance over time).
In the third step, within patients, we analyzed the influence of lesion location (frontal and temporal (n = 11) versus frontal+ (n = 11, including frontal and other lesions except temporal lesions) versus temporal+ (n = 4, including temporal and other lesions except frontal lesions) versus other lesions (n = 5, including subcortical or cerebellar lesions) versus no lesions (n = 3)) on recall (30-min and 1-week) as well as recognition (30-min and 1-week) performance using non-parametric Kruskal-Wallis tests. Furthermore, we analyzed the influence of laterality (right (n = 12) versus left (n = 8) versus bilateral (n = 11) versus no laterality (n = 3)) on recall (30-min and 1-week) as well as recognition performance (30-min and 1-week) using non-parametric Kruskal-Wallis tests.
Fourth, we calculated z-scores for 1-week recall using age-specific mean and SD values derived from the whole healthy control sample (n = 218; divided into 3 age groups: young (age 18–29 years, n = 86), middle (age 30–59 years, n = 95), old (age ≥60 years, n = 36); z-scores < −1.5 SD below the mean were interpreted as ALF.
Results
Demographics of patients and controls
As shown in , patients with ABI and healthy controls were comparable regarding age, years of education and sex. However, there was a significant difference in age between the 3 patient subgroups, so we controlled for age in all patient subgroup analyses. Patient subgroups were comparable regarding the days between incidence and memory testing. However, patient subgroups differed regarding the localization of lesions and their laterality. Most patients with TBI had bilateral frontal and temporal lesions, whereas most stroke patients had right-sided frontal + lesions (frontal and other regions without temporal lesions); most patients in the ‘other diagnosis’ group had subcortical or no lesions, with either no laterality or a left-sided laterality.
Comparison of verbal learning and memory performance between patients with ABI versus healthy controls
As illustrated in , verbal learning and retroactive interference were comparable between patients with ABI (n = 34) and healthy controls (n = 54). However, patients showed a significantly reduced recall in the interference list compared to healthy controls. Regarding verbal recall over time, repeated measures ANOVA indicated a significant effect of time (F(1.43, 122.93) = 206.16, p < 0.001, ηp2 = .71) as well as a significant interaction effect of time × group (F(1.43, 122.93) = 20.81, p < 0.001, ηp2 = .20) and group effect (F (Citation1) 86) = 4.99, p < 0.05, ηp2 = .06), as illustrated in . Patients recalled fewer words and pairwise post-hoc comparisons revealed that recall after 1 week was significantly reduced compared to recall 30 min after learning (p < 0.001, M = −4.12, 95% CI − 4.75, −3.50) as well as compared to short-term recall after learning (p < 0.001, M = −4.09, 95% CI − 4.78, −3.41). As illustrated in , cross-sectional independent t-tests indicated a significant group difference regarding 1-week recall, with patients having a reduced recall compared to controls, without any further group differences regarding short-term or 30-min recall. Furthermore, independent t-tests indicated a significant difference in recall loss over time, showing that patients had a significantly higher recall loss over 1 week compared to healthy controls.
Figure 2. Verbal recall over time between patients with ABI and healthy controls.
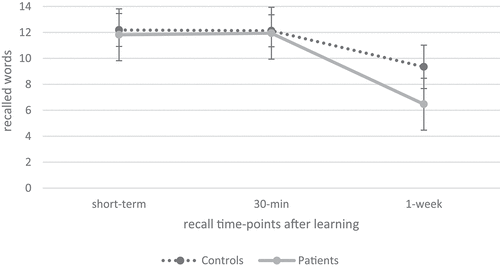
Table 3. Overview of neuropsychological performance in healthy controls and patients with acquired brain injury (ABI).
Regarding recognition performance over time, as illustrated in , repeated measures ANOVA indicated a significant effect of time (F(1.00, 79.00) = 74.66, p < 0.001, ηp2 = .49) and group (F (Citation1) 79) = 4.39, p < 0.05, ηp2 = .05) as well as a significant interaction effect of time × group (F(1.00, 79.00) = 6.50, p < 0.05, ηp2 = .08) on recognition performance. Post-hoc comparisons revealed that recognition after 1 week was significantly reduced compared to recognition 30 min after learning (p < 0.001, M = −2.26, 95% CI −2.78, −1.74). Additionally, as indicated in , cross-sectional independent t-tests indicated a significant group difference regarding recognition performance after 1 week, but not after 30 min. Furthermore, recognition loss over time was significantly elevated in patients compared to healthy controls.
Figure 3. Verbal recognition over time between patients with ABI and healthy controls.
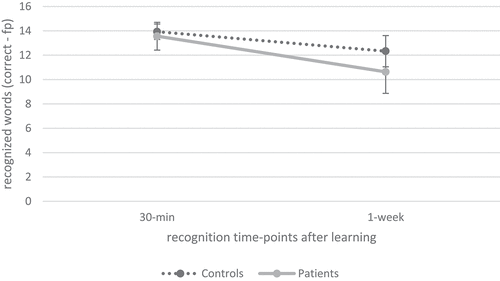
Given that seizures are associated with ALF, we analyzed whether the reduced 1-week recall is still observable by excluding the six patients with confirmed or suspected initial seizures. The results did not change and, repeated measures ANOVA still indicated a significant effect of time (F(1.40, 111.68) = 182.71, p < 0.001, ηp2 = .70) as well as a significant interaction effect of time × group (F(1.40, 111.68) = 18.37, p < 0.001, ηp2 = .19), without an effect of group (F (Citation1) 80) = 3.53, p = 0.06, ηp2 = .04) on verbal recall. Patients recalled significantly fewer words and pairwise post-hoc comparisons revealed that recall after 1 week was significantly reduced compared to recall 30 min after learning (p < 0.001, M = −4.09, 95% CI − 4.76, −3.41) as well as compared to short-term recall after learning (p < 0.001, M = −4.13, 95% CI − 4.86, −3.41). Cross-sectional independent t-tests indicated that patients recalled significantly less words after 1 week compared to healthy controls (t(80)=-3.7, p < 0.001,d = −0.86), whereas short-term and 30-min recall was comparable between groups. Regarding recognition performance, by excluding the six patients with confirmed or suspected seizures, repeated measures ANOVA indicated significant effects of time (F(1.00, 74.00) = 66.81, p < 0.001, ηp2 = .47) as well as a significant interaction effect time × group (F(1.00, 74.00) = 3.71, p = 0.02, ηp2 = .07), without an effect of group (F (Citation1) 74) = 3.20, p = 0.08, ηp2 = .04). Patients recognized significantly fewer words and pairwise post-hoc comparisons revealed that recognition after 1 week was significantly reduced compared to recognition 30 min after learning (p < 0.001, M = −2.25, 95% CI − 2.80, −1.70). Furthermore, cross-sectional independent t-tests indicated that patients recognized significantly less words after 1-week compared to healthy controls (t(29.9)=-1.89, p < 0.05, d=-.55), while 30-min recognition was comparable between groups.
Executive functions and their association with verbal memory performance
As shown in , independent t-tests indicated significant group differences regarding working memory and word fluency. Thus, patients with ABI showed a significantly reduced performance in complex executive functions.
Group-specific, Bonferroni-corrected correlations between verbal recall and executive functions are illustrated in . Overall, correlations were weak, with the exception of a moderate, almost statistically significant correlation between 1-week recall and verbal fluency in patients. In healthy controls, there were weak and non-significant correlations between verbal recall and executive functions. Furthermore, we computed the correlations between interference recall from the verbal learning test and verbal recall, as illustrated in . Whereas, in patients, retroactive interference as well as interference recall correlated significantly with 30-min recall, there were significant correlations between retroactive interference and 30-min recall as well as 1-week recall in healthy adults. Furthermore, interference recall correlated significantly with 1-week recall in healthy adults only.
Table 4. Group-specific Pearson correlation coefficients (p-values) between verbal recall (30-min and 1-week) and executive functions in the overall sample.
Table 5. Group-specific Pearson correlation coefficients (p-values) between verbal recall (30-min and 1-week) and retro- and proactive interference in the overall sample.
Verbal learning and memory performance between the patient subgroups (TBI vs. stroke vs. other diagnosis) and healthy controls
In a second step, we investigated verbal memory performance between the patient subgroups (TBI (n = 10) vs. stroke (n = 14) vs. other diagnosis (n = 10)) and healthy controls (n = 54). As illustrated in , our ANCOVAs indicated a comparable performance between the patient subgroups and healthy controls in verbal learning, short-term recall, interference recall, retroactive interference, 30-min recall and 30-min recognition. For verbal recall performance over time, repeated measures ANCOVA indicated a significant effect of time (F(1.51, 125.26) = 34.55, p < 0.001, ηp2 = .29) as well as a significant interaction effect of time × group (F(4.53, 125.26) = 9.16, p < 0.001, ηp2 = .25), but no group effect (F (Citation3) 83) = 1.76, p = 0.16, ηp2 = .06). Post-hoc pairwise comparisons indicated that fewer words were recalled 1 week after learning compared to short-term recall (p < 0.001, M = −4.86, 95% CI [−5.68, −4.03]) as well as 30-min after learning (p < 0.001, M = −4.92, 95% CI [−5.67, −4.17]). As illustrated in , this interaction effect was driven by the patients after TBI and the patients from the ‘other diagnosis’ group. Similar, the ANCOVA indicated a significant difference between the patient subgroups and healthy controls in recall loss, as shown in . Pairwise post-hoc comparisons revealed that patients after TBI (p < 0.001, M = 30.07, 95% CI [11.80, 48.33]) as well as patients in the ‘other diagnosis’ group (p < 0.001, M = 30.62, 95% CI [12.57, 48.67]) had a significantly greater recall loss than healthy controls. This result was confirmed regarding 1-week recall; cross-sectional ANCOVA indicated a significant group difference between patient subgroups and healthy controls; pairwise post-hoc comparisons revealed that patients after TBI (p < 0.01, M = −3.68, 95% CI [−6.60, −0.76]) as well as patients in the ‘other diagnosis’ group (p < 0.01, M = −3.69, 95% CI [−6.57, −0.80]) had a significantly reduced 1-week recall compared to healthy controls. Regarding recognition performance over time, repeated measures ANCOVA indicated a significant interaction of time × group (F(3.00, 76.00) = 6.42, p < 0.01, ηp2 =.20); but no effect of time (F(1.00, 76.00) = 1.42, p = 0.24, ηp2 = .02) or group (F (Citation3) 76) = 2.15, p = 0.10, ηp2 = .08), as illustrated in . Post-hoc pairwise comparisons indicated that fewer words were recognized 1 week after learning than 30 min after learning (p < 0.001, M = −2.74, 95% CI [−3.36, −2.12]), but pairwise post-hoc analysis did not detect any significant group difference between the patient subgroups and controls. There was a significant effect of group on recognition loss over time (); pairwise post-hoc comparisons indicated that patients after TBI (p < 0.05, M = 17.43, 95% CI [0.97, 33.88]) and patients from the ‘other diagnosis’ group (p < 0.05, M = 21.07, 95% CI [2.78, 39.37]) had a greater recognition loss between 30-min and 1-week recognition compared to the healthy controls. Furthermore, patients after TBI (p < 0.05, M = 24.90, 95% CI [2.76, 47.04]) as well as patients in the ‘other diagnosis’ group (p < 0.01, M = 28.55, 95% CI [5.05, 52.05]) had a significantly greater recognition loss than patients after stroke.
Figure 4. Verbal recall over time between patients with ABI subgroups (TBI, stroke and other diagnosis) and healthy controls.
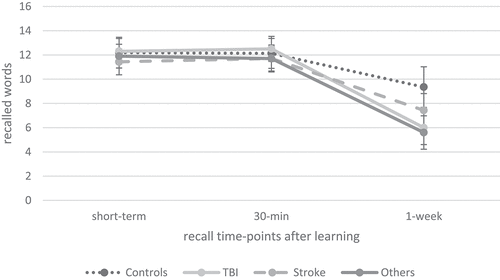
Figure 5. Verbal recognition over time between patients with ABI subgroups (TBI, stroke and other diagnosis) and healthy controls.
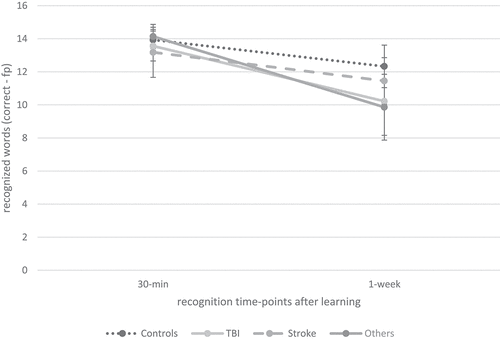
Although there were significant/borderline group effects regarding executive functions (working memory and verbal fluency, as indicated in ), pairwise post-hoc analysis did not detect any significant difference between the patient subgroups (TBI vs. stroke vs. other diagnosis) and controls.
Influence of lesion location and laterality on verbal recall and recognition performance in patients
As illustrated in , we did not find significant effects of lesion location (frontal+ (n = 11); frontal and temporal (n = 11); temporal+ (n = 4); other lesions (n = 5); normal MRI (n = 3)) on recall (30-min and 1-week) or recognition (30-min and 1-week) performance using Kruskal-Wallis H tests. Furthermore, we did not find significant effects of laterality (right laterality (n = 12); left laterality (n = 8); bilateral (n = 11); no laterality (n = 3)) on recall (30-min and 1-week) or recognition (30-min and 1-week) performance using Kruskal-Wallis H tests, as shown in .
Table 6. Overview of memory recall and recognition performance (30-min and 1-week) within patients according to their lesion location.
Table 7. Overview of memory recall and recognition performance (30-min and 1-week) within patients according to their lesion laterality.
Detection of ALF in patients with ABI
We calculated z-scores for the 1-week recall using age -specific mean and SD values derived from the overall healthy control sample. As described above, z-scores below −1.5 SD of the mean were interpreted as ALF. According to this definition, seven patients (21%) as well as three healthy controls (6%) exhibited ALF, which would have been missed using a standardized memory test without a delayed recall time-point. Compared to healthy controls significantly more patients with ABI had z-scores below −1.5 SD, (X2 (1) = 4.68, p < 0.05, φ = 0.23) distributed as follows: 3/10 patients after TBI (30%), 1/14 patient after stroke (7%) and 3/10 patients with other diseases (30%; consisting of 2 patients with a frontal tumor (20%) and 1 patient with limbic encephalitis (10%)) who had a z-score < −1.5 SD below the mean. In the healthy control group, 3/54 participants (6%) had an impaired delayed recall z-score.
Discussion
ALF is a relatively recently identified memory phenomenon that describes accelerated forgetting over time after an initially normal learning and memory performance using standardized tests (Citation2). Similar to results of earlier studies involving patients with TLE (Citation3), minor stroke (Citation7) or TBI (Citation6), this retrospective study revealed that patients in the post-acute period after an ABI had a significantly impaired 1-week recall and recognition performance compared to a well-matched healthy control group. Given that the associations between executive functions and verbal recall were nonsignificant in patients, we interpret the significantly reduced verbal recall and recognition performance in patients with ABI as an impairment of memory consolidation, rather than an impairment of retrieval. Further evidence for a consolidation impairment comes from the patient subgroup analysis, which aimed to investigate the influence of ABI etiology on delayed memory performance. These results illustrate that patients after TBI and patients in the ‘other diagnosis’ group (consisting of patients who had a resection of brain tissue, an encephalitis or hypoxic encephalopathy) had a significantly reduced 1-week recall. Furthermore, patients after TBI and patients with other diagnosis had a significantly elevated recognition loss compared to healthy controls and patients after stroke. While patients after TBI may be prone to ALF due to typical widespread fronto-temporal lesions, impairing the hippocampal-neocortical memory transformation (Citation17), the explanation why patients from the ‘other diagnosis’ group were impaired on 1-week recall is at first sight less clear, especially since three patients in this group had a normal MRI scan. By analyzing the individual results in the ‘other diagnosis’ group, we found that the impaired 1-week recall was driven by two patients with a frontal tumor and one patient with limbic encephalitis who showed ALF (see ). Thus, our results support the finding of a recent study (Citation22) who found that limbic encephalitis was associated with ALF, leading to the conclusion that it could represent a non-lateralized fronto-limbic dysfunction rather than a lateralized hippocampal impairment.
Although studies on ALF were unable to identify specific lesion characteristics in patients with ALF (Citation6,Citation8), recent evidence from animal studies using optogenetics suggest that 3 phases of synaptic plasticity are involved in stabilization of new memories: the first phase occurs locally in the hippocampus, the second during sleep on the same day, and the third phase in the anterior cingulate cortex during sleep on the second day (Citation31). These data suggest that the hippocampal-neocortical transformation underlying memory building takes place within the first few hours after learning and that frontal regions are involved early on in consolidation. Thus, in patients with frontal lesions, episodic long-term recall may be impaired 1 week after learning.
Although we assume that ALF is associated with a consolidation impairment, we cannot be sure that ALF might not also reflect, to some extent, a retrieval impairment. Given that we did not examine strategies applied during encoding, which improve delayed recall in standardized memory tests in other patient samples such as those with mild TBI (Citation32,Citation33), we may have underestimated the influence of executive functions on delayed memory performance 1 week after learning. Thus, future research should investigate the role of learning in delayed recall performance as well as the influence of sleep. Given that sleep, especially slow wave sleep, is known to be important for episodic memory consolidation by strengthening and stabilizing memories (Citation34), disrupted sleep might impair memory consolidation and might thus be associated with ALF. Interestingly, so far only a few studies with adult patients have investigated the influence of sleep on delayed memory performance, with controversial results (Citation35–37). It seems that sleep does not always improve memory consolidation overnight, especially in patients with epilepsy, implying that epileptiform activity at night may confound consolidation. Thus, the influence of sleep on memory consolidation needs further investigation, especially in non-epileptic patients. Besides supporting consolidation, sleep protects new memories against interference (Citation38). Interestingly, retroactive interference was significantly associated with delayed 1-week recall in healthy participants, but not in patients with ABI. This means that healthy participants who are less distracted by new material have a significantly better delayed 1-week recall than participants who are more affected by retroactive interference. In patients, this association was only observable in the 30-min recall tests, but not the 1-week recall, suggesting that disruption of memory consolidation in patients occurred within a week.
Overall, our study supports the assumption that ALF is not an epilepsy-specific disorder as it is also observable in patients without seizures. Although all included patients with ABI showed an average learning and recall performance in a standardized memory test, our results imply that reduced delayed memory recall 1 week after learning may also occur in patients with neurological disorders without overt seizures, supporting the idea that ALF is a marker of non-disorder-specific memory disruption (Citation10). Future studies should investigate whether impaired delayed verbal recall after 1 week is clinically relevant, including questionnaires on memory skills and participation in everyday life. We are convinced that it is, since affected patients often show average memory performance in standardized memory tests, but nurses or family members observe memory impairments in daily life. In epilepsy patients, there is also evidence that recall performance after an extended delay was related to self-reported memory impairments whereas recall performance after 30 minutes was not (Citation39). Furthermore, there is evidence that self-reported memory problems are associated with the number of recalled words 4 weeks after learning, whereas recall performance 1 week after learning did not correlate with subjective memory rating (Citation40). Thus, applying longer retention intervals may help to illustrate subjectively perceived memory impairments as well as advance the ecological validity of list learning tests.
Our study has some limitations that should be considered: First, we do not have a measure of pre-morbid cognitive performance (IQ). However, given that all patients visited regular schools, we assume a normal intelligence. Second, we had a generous, but clinically useful, definition of normal learning and 30-minutes recall including patients with a standardized z-score ≥−1.5. Third, we did not ask all healthy controls whether they expected a memory recall at T2 after one week and could thus overestimate memory recall in healthy controls. Fourth, we did not investigate familiarity and recollection processes, which would have been informative regarding consolidation impairment. Fifth, although none of the patients had overt seizures between ABI and neuropsychological testing, there were six patients with confirmed or suspected initial seizures. Nevertheless, even after excluding these six patients, the patient group still showed a significantly reduced verbal recall and recognition performance after 1 week, supporting our assumption that reduced delayed memory performance can also be observed in patients without current or recently experienced seizures.
Conclusions
This study demonstrates that patients with ABI, especially patients after TBI and frontal tumors, are at risk of an impaired delayed verbal memory performance 1 week after learning. Our results support the assumption that ALF results from a consolidation impairment since verbal recall and recognition after 1 week was impaired in patients with ABI, without significant correlations between executive functions and delayed memory performance. However, future studies should examine strategies applied during encoding which can influence delayed memory performance. We propose to include delayed memory measures in clinical memory evaluations, especially in patients with frontal and temporal lesions as well as in patients with limbic encephalitis. Furthermore, there is a need for age- and education-appropriate standard norms for memory recall after 1 week to enable clinicians to detect patients with impaired 1-week recall.
Credit author statement
Martina Studer: Conceptualization, Methodology, Formal Analysis, Investigation, Data Curation, Writing – Original Draft, Supervision, Project administration. Adrian Guggisberg: Methodology, Resources, Formal Analysis, Writing – Review & Editing, Supervision. Naomi Gyger: Investigation, Data Curation, Visualization, Project administration. Klemens Gutbrod: Conceptualization, Writing – Review & Editing. Katharina Henke: Conceptualization, Methodology, Writing – Review & Editing, Supervision. Dörthe Heinemann: Conceptualization, Methodology, Writing – Review & Editing, Supervision
Acknowledgments
The authors would like to thank Susan Kaplan for proofreading this manuscript. Additionally, the authors thank the Reviewers for critically reading and improving the manuscript with their inputs.
Disclosure statement
No potential conflict of interest was reported by the author(s).
Additional information
Funding
References
- Dickerson BC, Eichenbaum H. The episodic memory system: neurocircuitry and disorders. Neuropsychopharmacology. 2010;35(1):86–104. doi:10.1038/npp.2009.126.
- Savage S, Hoefeijzers S, Milton F, Streatfield C, Dewar M, Zeman A. The evolution of accelerated long-term forgetting: evidence from the TIME study. Cortex. 2019;110:16–36. doi:10.1016/j.cortex.2017.09.007.
- Muhlert N, Milton F, Butler CR, Kapur N, Zeman AZ. Accelerated forgetting of real-life events in transient epileptic amnesia. Neuropsychologia. 2010;48(11):3235–44. doi:10.1016/j.neuropsychologia.2010.07.001.
- Geurts S, van der Werf SP, Kessels RPC. Accelerated forgetting? An evaluation on the use of long-term forgetting rates in patients with memory problems. Front Psychol. 2015;6:6. doi:10.3389/fpsyg.2015.00752.
- Isaac CL, Mayes AR. Rate of forgetting in amnesia: II. Recall and recognition of word lists at different levels of organization. J Exp Psychol Learn Mem Cogn. 1999;25(4):963–77. doi:10.1037/0278-7393.25.4.963.
- Lah S, Black C, Gascoigne MB, Gott C, Epps A, Parry L. Accelerated long-term forgetting is not epilepsy specific: evidence from childhood traumatic brain injury. J Neurotrauma. 2017;34(17):2536–44. doi:10.1089/neu.2016.4872.
- Geurts S, van der Werf SP, Kwa VIH, Kessels RPC. Accelerated long-term forgetting after TIA or minor stroke: a more sensitive measure for detecting subtle memory dysfunction? Cortex. 2019;110:150–56. doi:10.1016/j.cortex.2018.04.002.
- Lammers NA, Lugtmeijer S, de Haan EHF, Kessels RPC. Accelerated long-term forgetting: prolonged delayed recognition as sensitive measurement for different profiles of long-term memory and metacognitive confidence in stroke patients. J Int Neuropsychol Soc. 2022;28(4):327–36. doi:10.1017/S1355617721000527.
- Weston PSJ, Nicholas JM, Henley SMD, Liang Y, Macpherson K, Donnachie E. Accelerated long-term forgetting in presymptomatic autosomal dominant Alzheimer’s disease: a cross-sectional study. Lancet Neurol. 2018;17(2):123–32. doi:10.1016/S1474-4422(17)30434-9.
- Mameniškienė R, Puteikis K, Jasionis A, Jatužis D. A review of accelerated long-term forgetting in epilepsy. Brain Sci. 2020;10(12):945. doi:10.3390/brainsci10120945.
- Zeman A, Butler C, Muhlert N, Milton F. Novel forms of forgetting in temporal lobe epilepsy. Epilepsy Behav. 2013;26(3):335–42. doi:10.1016/j.yebeh.2012.09.030.
- Butler CR, Bhaduri A, Acosta-Cabronero J, Nestor PJ, Kapur N, Graham KS, Hodges JR, Zeman AZ. Transient epileptic amnesia: regional brain atrophy and its relationship to memory deficits. Brain. 2009;132(2):357–68. doi:10.1093/brain/awn336.
- Lah S, Mohamed A, Thayer Z, Miller L, Diamond K. Accelerated long-term forgetting of verbal information in unilateral temporal lobe epilepsy: is it related to structural hippocampal abnormalities and/or incomplete learning? J Clin Exp Neuropsychol. 2014;36(2):158–69. doi:10.1080/13803395.2013.874405.
- Miller LA, Mothakunnel A, Flanagan E, Nikpour A, Thayer Z. Accelerated long term forgetting in patients with focal seizures: incidence rate and contributing factors. Epilepsy Behav. 2017;72:108–13. doi:10.1016/j.yebeh.2017.04.039.
- Wilkinson H, Holdstock JS, Baker G, Herbert A, Clague F, Downes JJ. Long-term accelerated forgetting of verbal and non-verbal information in temporal lobe epilepsy. Cortex. 2012;48(3):317–32. doi:10.1016/j.cortex.2011.01.002.
- Baker J, Zeman A. Accelerated long-term forgetting in epilepsy—and beyond. In: Cognitive neuroscience of memory consolidation. Cham, Switzerland: Springer International Publishing; 2017. pp. S. 401–17.
- Huijgen J, Samson S. The hippocampus: a central node in a large-scale brain network for memory. Rev Neurol (Paris). 2015;171(3):204–16. doi:10.1016/j.neurol.2015.01.557.
- Grayson-Collins J, Gascoigne MB, Barton B, Webster R, Gill D, Lah S. Longitudinal study of accelerated long-term forgetting in children with genetic generalized epilepsy: evidence of ongoing deficits. Cortex. 2019;110:5–15. doi:10.1016/j.cortex.2017.08.028.
- Hill BD, Alosco M, Bauer L, Tremont G. The relation of Executive functioning to CVLT-II learning, memory, and process indexes. Appl Neuropsychol Adult. 2012;19(3):198–206. doi:10.1080/09084282.2011.643960.
- Joplin S, Webster R, Gill D, Barton B, Lawson JA, Mandalis A. Accelerated long-term forgetting in children with genetic generalized epilepsy: the temporal trajectory and contribution of executive skills. Epilepsy Behav. 2020;113:107471. doi:10.1016/j.yebeh.2020.107471.
- Joplin S, Gascoigne M, Barton B, Webster R, Gill D, Lawson JA. Accelerated long-term forgetting in children with temporal lobe epilepsy: a timescale investigation of material specificity and executive skills. Epilepsy Behav. 2022;129:108623. doi:10.1016/j.yebeh.2022.108623.
- Helmstaedter C, Winter B, Melzer N, Lohmann H, Witt JA. Accelerated long-term forgetting in focal epilepsies with special consideration given to patients with diagnosed and suspected limbic encephalitis. Cortex. 2019;110:58–68. doi:10.1016/j.cortex.2018.01.003.
- Spreij LA, Visser-Meily JMA, van Heugten CM, Nijboer TCW. Novel insights into the rehabilitation of memory post acquired brain injury: a systematic review. Front Hum Neurosci. 2014;8. doi:10.3389/fnhum.2014.00993.
- Forkmann T, Norra C, Wirtz M, Gauggel S, Boecker M. Psychometric evaluation of the rasch-based depression screening in patients with neurologic disorders. Arch Phys Med Rehabil. 2010;91(8):1188–93. doi:10.1016/j.apmr.2010.04.021.
- Holthe IL, Dahl HM, Rohrer-Baumgartner N, Eichler S, Elseth MF, Ø H. Neuropsychological impairment, brain injury symptoms, and health-related quality of life after Pediatric TBI in Oslo. Front Neurol. 2021;12:719915. doi:10.3389/fneur.2021.719915.
- Tanner-Eggen C, Balzer C, Perrig WJ, Gutbrod K. The neuropsychological assessment of cognitive deficits considering measures of performance variability. Arch Clin Neuropsychol. 2015;30(3):217–27. doi:10.1093/arclin/acv008.
- Balzer C, Berger JM, Caprez G, Gonser A, Gutbrod K, Keller M. Materialien und Normwerte für die neuropsychologische Diagnostik MNND. Rheinfelden: Verlag Normdaten; 2011.
- Wechsler D. WMS-R: Wechsler memory scale-revised. San Antonio, TX: Psychological Corporation; 1987.
- Aita SL, Beach JD, Taylor SE, Borgogna NC, Harrell MN, Hill BD. Executive, language, or both? An examination of the construct validity of verbal fluency measures. Appl Neuropsychol Adult. 2019;26(5):441–51. doi:10.1080/23279095.2018.1439830.
- Benton AL, Hamsher K. Controlled oral word association test (COWAT). Multilingual aphasia examination. Iowa City: AJA; 1989.
- Goto A, Bota A, Miya K, Wang J, Tsukamoto S, Jiang X. Stepwise synaptic plasticity events drive the early phase of memory consolidation. Sci. 2021;374(6569):857–63. doi:10.1126/science.abj9195.
- Broadway JM, Rieger RE, Campbell RA, Quinn DK, Mayer AR, Yeo RA. Executive function predictors of delayed memory deficits after mild traumatic brain injury. Cortex J Devoted Study Nerv Syst Behav. 2019 November;120:240–48. doi:10.1016/j.cortex.2019.06.011.
- Geary EK, Kraus MF, Rubin LH, Pliskin NH, Little DM. Verbal learning strategy following mild traumatic brain injury. J Int Neuropsychol Soc. 2011;17(4):709–19. doi:10.1017/S1355617711000646.
- Diekelmann S, Büchel C, Born J, Rasch B. Labile or stable: opposing consequences for memory when reactivated during waking and sleep. Nat Neurosci. 2011;14(3):381–86. doi:10.1038/nn.2744.
- Atherton KE, Nobre AC, Zeman AZ, Butler CR. Sleep-dependent memory consolidation and accelerated forgetting. Cortex. 2014;54:92–105. doi:10.1016/j.cortex.2014.02.009.
- Atherton KE, Nobre AC, Lazar AS, Wulff K, Whittaker RG, Dhawan V. Slow wave sleep and accelerated forgetting. Cortex. 2016;84:80–89. doi:10.1016/j.cortex.2016.08.013.
- Hoefeijzers S, Dewar M, Della Sala S, Butler C, Zeman A. Accelerated long-term forgetting can become apparent within 3-8 hours of wakefulness in patients with transient epileptic amnesia. Neuropsychology. 2015;29(1):117–25. doi:10.1037/neu0000114.
- Norman KA. Declarative memory: sleep protects new memories from interference. Curr Biol. 2006;16(15):R596–7. doi:10.1016/j.cub.2006.07.008.
- Fitzgerald Z, Thayer Z, Mohamed A, Miller LA. Examining factors related to accelerated long-term forgetting in epilepsy using ambulatory EEG monitoring. Epilepsia. 2013;54(5):819–27. doi:10.1111/epi.12090.
- Witt JA, Glöckner C, Helmstaedter C. Extended retention intervals can help to bridge the gap between subjective and objective memory impairment. Seizure. 2012;21(2):134–40. doi:10.1016/j.seizure.2011.10.007.