ABSTRACT
People often need to filter relevant from irrelevant information. Irrelevant emotional distractors interrupt this process. But does the degree to which emotional distractors disrupt attention depend on which visual field they appear in? We thought it might for two reasons: (1) people pay slightly more attention to the left than the right visual field, and (2) some research suggests the right-hemisphere (which, in early visual processing, receives left visual field input) has areas specialised for processing emotion. Participants viewed a rapid image-stream in each visual field and reported the rotation of an embedded neutral target preceded by a negative or neutral distractor. We predicted that the degree to which negative (vs. neutral) distractors impaired target detection would be larger when targets appeared in the left than the right stream. This hypothesis was supported, but only when the distractor and target could appear in the same or opposite stream as each other (Experiments 2a–b), not when they always appeared in the same stream as each other (Experiments 1a–1b). However, this effect was driven by superior left-stream accuracy following neutral distractors, and similar left- and right-stream accuracy following negative distractors. Emotional distractors therefore override visuospatial asymmetries and disrupt attention, regardless of visual field.
Attention is drawn to emotional information (Anderson, Citation2005) – allowing people to detect and respond to potential environmental threats (Carretié, Citation2014). Increased attention to emotional information comes at the cost of processing neutral information: emotional distractors reliably impair people’s ability to notice subsequent neutral targets in a rapidly presented image stream (Most et al., Citation2005). But is the degree to which emotional distractors impair detection of subsequent neutral targets – termed emotion-induced blindness – affected by where the distractors and targets appear? Hemispheric asymmetries in visuospatial attention and emotional processing suggest it might be. First, people pay slightly more attention to the left than the right side of space (i.e. pseudoneglect; Bowers & Heilman, Citation1980), due to greater activation in right- than left-hemisphere regions for tasks that require visuospatial attention (e.g. Karolis et al., Citation2019). Second, there is some evidence that regions within the right-hemisphere are specialised for processing negative stimuli (Davidson & Fox, Citation1982; Gainotti, Citation1972), particularly when stimuli first appear (e.g. Shobe, Citation2014). Therefore, although people are generally better at detecting targets in the left than the right visual field (e.g. Mulckhuyse et al., Citation2017), we proposed that people might be more susceptible to emotional distraction in the left visual field. We adapted the emotion-induced blindness paradigm to test this proposal. In four pre-registered experiments, we tested whether negative distractors presented in the left visual field (initially processed mainly by the right-hemisphere) disrupted attention more than distractors presented in the right visual field (initially processed mainly by the left-hemisphere).
Emotion-induced blindness
Emotion-induced blindness (EIB) is a reliable effect where an emotional distractor impairs people’s awareness of a subsequent neutral target (Most et al., Citation2005). An EIB task comprises multiple trials in which several images appear for 100 ms each in a rapid serial visual presentation (RSVP) stream. Participants identify whether a neutral target image embedded in the stream – a photograph depicting a landscape or building – is rotated 90° clockwise or counter-clockwise. On most trials, a neutral or emotional distractor precedes the target. The number of items between the distractor and target is termed the trial “lag”. When an emotional distractor appears one or two items before the target (i.e. lag 1 and 2 trials), participants’ target detection is reduced relative to when a neutral distractor appears (Kennedy & Most, Citation2015). This impairment weakens but still exists when the target appears four or six items after the distractor (i.e. lag 4 and lag 6 trials), with minimal impairment with eight items between the distractor and target (i.e. lag 8; Ciesielski et al., Citation2010). In other words, target detection gradually improves as participants recover from emotional distraction.
Participants show similar EIB when they respond immediately after seeing the target and when they respond after the last image in the stream (Kennedy & Most, Citation2012). Thus, EIB is not driven by participants’ failure to remember the target rotation by the time they respond. EIB also occurs in response to neutral distractors conditioned with an aversive noise (Smith et al., Citation2006), suggesting EIB is not driven by the emotional distractor images having distinct physical characteristics (see Goodhew & Edwards, Citation2022, for review). Further, EIB is remarkably difficult to extinguish. EIB persists when people are forewarned about the presence of emotional distractors (Kennedy et al., Citation2018), told what type of distractor to expect on each trial (Zhao & Most, Citation2019), incentivised to detect the targets (Most et al., Citation2007) or are shown familiar targets (e.g. the Sydney Opera House; Guilbert et al., Citation2019), suggesting EIB involves exogenous (bottom-up) rather than endogenous (top-down) attention processes (Carretié, Citation2014). In light of these findings, EIB is considered is considered a reflexive perceptual impairment that causes people to fail to encode the target image (Goodhew & Edwards, Citation2022; Onie et al., Citation2022).
EIB also occurs when people monitor two image streams simultaneously (e.g. Most & Wang, Citation2011). With dual streams, the distractor (emotional or neutral) and target can appear in the same or opposite location as one another. EIB occurs reliably when the emotional distractor appears in the same location as the neutral target (Kennedy et al., Citation2018; Most & Wang, Citation2011; Wang & Most, Citation2016) and strikingly less when the distractor and target appear in opposite locations, suggesting that EIB is spatially localised (Wang et al., Citation2012; but see Proud et al., Citation2020). Thus, EIB is attributed to spatiotemporal competition – i.e. the perceptual competition that arises when stimuli occur in overlapping points in time and space – between the emotional distractor and the target. People reflexively prioritise the emotional distractor, giving it the competitive edge (Wang et al., Citation2012).
In dual-stream EIB experiments to date, images have appeared above and below fixation (e.g. Kennedy et al., Citation2018). Researchers initially arranged the streams vertically, instead of horizontally, to avoid interference between the stream locations (left, right) and the “left” and “right” responses participants used to indicate target rotation (i.e. a variation of the Simon effect; Simon & Wolf, Citation1963). However, we do not yet know how EIB operates with horizontal streams. It may be that horizontal streams cause different patterns of EIB due to unequal distribution of attention across the left and right visual fields (i.e. visuospatial attention asymmetries; de Schotten et al., Citation2011).
We acknowledge that a horizontal stream version of the EIB task appears similar to the commonly used dot-probe task (MacLeod et al., Citation1986). However, evidence suggests that EIB involves different mechanisms to the dot probe; for example, whereas the dot probe reflects shifts of spatial attention from one location to another, emotion-induced blindness appears to reflect spatiotemporal competition between targets and emotional distractors (Wang et al., Citation2012). Further, emotional stimuli lead to shorter and less reliable disruptions in the dot-probe (∼20 ms) than the EIB task (100–400 ms; McHugo et al., Citation2013). Consequently, the EIB task is more appropriate than the dot-probe for determining how people generally engage with emotional stimuli (Goodhew & Edwards, Citation2022), including examining – in the current experiments – the influence of visuospatial attention and emotional processing asymmetries.
Visuospatial attention asymmetries
Most people pay slightly more attention to the left than the right visual field (e.g. Zago et al., Citation2016) – a phenomenon known as pseudoneglect (Bowers & Heilman, Citation1980). For example, when asked which of two equiluminant bars looks darker, people choose the bar that is darker on the left more often than the bar that is darker on the right (Mattingley et al., Citation2004). Similarly, when asked to bisect a horizontal line, people bisect it slightly to the left of centre; and when asked which side of a pre-bisected line looks longer, people more often choose the left side (Jewell & McCourt, Citation2000). Even in more complex attention tasks, such as visual search, people are faster and (sometimes) more accurate at identifying targets that appear on the left side of a displayFootnote1 (Mulckhuyse et al., Citation2017; Nicholls et al., Citation2017). In fact, pseudoneglect is most likely to benefit performance in the left visual field for high perceptual load tasks involving rapid stimuli presentation, like the EIB task (e.g. Asanowicz et al., Citation2012). However, it is not clear if and how emotional stimuli affect this leftward advantage. One possibility is that emotional stimuli disrupt attention more in the left than the right visual field because of emotional processing asymmetries.
Emotional processing asymmetries
Despite decades of research, the nature of emotional processing asymmetries remains debated. Arguably, the two most influential traditional theories are the right-hemisphere hypothesis (Gainotti, Citation1972) and the valence-specific (Davidson & Fox, Citation1982) or approach/avoidance (modified by Harmon-Jones, Citation2003) hypothesis. According to the right-hemisphere hypothesis, the right-hemisphere is responsible for processing all emotions, regardless of valence. According to the valence-specific hypothesis, the right-hemisphere is specialised for negative emotion, whereas the left-hemisphere primarily processes positive emotion. Despite their differences, traditional theories converge on right-hemisphere superiority for processing negative emotion, particularly highly arousing negative stimuli like the emotional distractors typically used in an EIB task.
Both the right hemisphere hypothesis and the valence-specific hypothesis are supported, and contradicted, by empirical work, depending on the tested population (patient vs. neurotypical) and type of stimuli (e.g. facial expressions, sounds), presentation (unilateral vs. bilateral) and response (behavioural vs. neuroimaging) (e.g. Ocklenburg & Güntürkün, Citation2018; Prete et al., Citation2014, Citation2018). Consequently, there is an expanding view that we should move away from the concept of a basic hemispheric dichotomy and acknowledge that several cortical areas in both the right- and left-hemispheres contribute to our experience of emotion (Palomero-Gallagher & Amunts, Citation2021). Further, emotional processing models have been predominantly informed by facial expression research. This limitation is notable, because facial expressions predominantly represent communicative and social aspects of emotional processing (Mavratzakis et al., Citation2016) and elicit few basic emotions (e.g. angry, happy). By contrast, emotional scenes lead to stronger and more stable affective neural activity and behavioural responses (e.g. spontaneous facial reactions of the viewer; Mavratzakis et al., Citation2016), promote more intense self-reported emotions (Thom et al., Citation2014), and provide more stimulus variation than faces. For these reasons, the EIB paradigm – which uses naturalistic scenes – is suitable for advancing understanding of emotional processing asymmetries; a secondary aim of this research.
The current research
In four pre-registered experiments we used the EIB paradigm to test whether people were more susceptible to emotional distraction in the left than the right visual field. Our primary interest in how visual field varied the size of the EIB effect informed the experimental design. First, we chose lags (i.e. the number of items between the distractor and target) where EIB reliably occurs (Kennedy & Most, Citation2015); we presented the distractor either two items before the target (lag 2) – which generally yields a robust EIB effect – or four items before the target (lag 4) – which generally yields a smaller EIB effect than lag 2. Second, we simplified the design by using exclusively negative, and not positive, distractors. Supporting this decision, studies that have included positive distractors (e.g. Most et al., Citation2007; Olatunji et al., Citation2013; Singh & Sunny, Citation2017) tend to find similar EIB for positive as negative distractors. Third, because vertical stream studies suggested EIB predominantly occurs when the distractor and target appear in the same spatial location (Wang et al., Citation2012), we started by always presenting the distractor and target in the same stream as one another (Experiment 1a–1b). We later manipulated whether the distractor and target appeared in the same or opposite stream as each other (Experiment 2a–2b). Pre-registrations (https://osf.io/xzs9w/registrations/), data and supplementary material for all experiments are publicly available (https://osf.io/xzs9w/files/).
Experiment 1a
We used a 2 (distractor valence: negative, neutral) x 2 (distractor/target stream: left, right) x 2 (lag: 2, 4) within-subjects design. In Experiment 1a (pre-registration: http://osf.io/xb4fg/) and Experiment 1b, the distractor and target always appeared in the same stream. We predicted that participants would show better target detection for left than right stream targets and following neutral than negative distractors. But our primary hypothesis was that distractor valence (negative, neutral) and stream (left, right) would interact, such that there would be a stronger EIB effect for images presented in the left stream (i.e. images initially processed by the right-hemisphere) compared to images presented in the right stream (i.e. images initially processed by the left-hemisphere).
There is some evidence that EIB may be influenced by psychopathology, including anxiety (e.g. Haddara et al., Citation2019; Most et al., Citation2010) and Posttraumatic Stress Disorder (PTSD) symptoms (Olatunji et al., Citation2013), though other studies have failed to replicate relationships between EIB and trait variables (Moeck et al., Citation2022). We included measures of current depression, anxiety, stress and PTSD symptoms to determine whether they were associated with EIB (see supplementary material for measures and results: https://osf.io/fcwmr/), and found minimal evidence of any associations.
Method
Sample size justification. In several dual-stream EIB experiments, Most and Wang (Citation2011) found a large difference in target detection accuracy when the target was preceded by a negative versus a neutral distractor (ds = 0.7–1.3). Although this effect was large and reliable, we were interested in the difference in effect size depending on visual field. Our prior research (Moeck et al., Citation2020) showed small to medium-sized visual field differences for recognition memory of negative images (e.g. dz = 0.35). We therefore conducted a power analysis (G*Power) with this smaller effect size (dz = 0.35). We based our sample size on a t-test for visual field differences following negative distractors, rather than a three-way interaction between valence, visual field and lag, because at the time we estimated sample size (2018), G*Power could not estimate samples for designs with more than one within-subjects factor. We needed a sample size of at least 61 to maintain 85% power at alpha = .05. Due to always using a within-subjects design with the same number of factors, we aimed for 61 useable participants per experiment.
Participants. We recruited 65 right-handedFootnote2 university students with normal or corrected-to-normal vision. We excluded two participants based on their performance on the EIB task: one whose overall accuracy (collapsed across all trials) was 2SDs below the rest of the sample (M = 63.06, SD = 6.08) and one who had below chance accuracy on baseline trials, which have no distractors. We also excluded one participant who was not strongly right-handed, determined by scoring less than +8 Flinders Handedness Survey – where −8 to −10/+8 to +10 indicates someone is strongly left/right handed (FLANDERS; Nicholls et al., Citation2013). Our final sample of 62 participants ranged from 18 to 33 years old (M = 21.3, 95% CI [20.4, 22.3]), were predominantly women (77%; 23% men; 0% non-binary), and strongly right-handed (M = + 9.98, 95% CI [9.94, 10.00], range +9 to +10 on the FLANDERS). Participants provided written informed consent before the experiment, which was approved by the Flinders University Social and Behavioural Research Ethics Committee. Participants received course credit or $10.00AUD.
Materials
Apparatus. We used MATLAB and Psychophysics Toolbox extensions (Brainard, Citation1997; Pelli, Citation1997) to programme and present the experiments. We used a 20 × 13-inch monitor, with a refresh rate of 59.86 Hz and screen resolution of 1680 × 1050 pixels. We adapted the programme used by Zhao and Most (Citation2019) to incorporate a modified divided visual field paradigm: a central fixation cross appeared alone for 100 ms and was then accompanied by two RSVP image streams, one in each visual field (left and right). The medial edge of each image stream (320 × 240 pixels) was 125 pixels (2.5° visual angle) from the central fixation cross. We instructed participants to fixate on the cross during image presentation (Bourne, Citation2006). To avoid changes in visual angle, a chin rest ensured the participant’s head was in a fixed position throughout the experiment, 500 mm from the screen.
Images. There were 56 negative and 56 neutral distractor images sourced from the International Affective Picture System (Lang et al., Citation2005) and other public sources. These images matched Kennedy and Most (Citation2012), who previously gathered valence (from 1 = negative to 9 = positive) and arousal (from 1 = low to 9 = high) ratings from 12 pilot participants. The negative distractor images depicted threatening animals, medical trauma, or violence (valence: M = 1.73, SD = 0.54; arousal: M = 6.04, SD = 0.69); while the neutral distractor images depicted animals and people without obvious emotional content (valence: M = 4.99, SD = 0.45; arousal: M = 3.18, SD = 0.55). The negative and neutral distractor sets had the same number of images including people and animals. The targets were 122 landscape images rotated 90° clockwise (right) or 90° counterclockwise (left).Footnote3 We had an additional 253 filler items, comprising upright landscape and architectural images.
Procedure. Following consent, participants rated their depression, anxiety and stress symptoms (via the DASS-21; Lovibond & Lovibond, Citation1995) and answered questions assessing handedness (via the FLANDERS; Nicholls et al., Citation2013). They then completed the experiment individually at a computer, beginning with eight practice trials without distractors. The speed of the practice trials gradually increased from 200 to 100 ms per item. Following the practice trials, the researcher confirmed participants understood the task instructions and provided any clarifications. Participants then completed four experimental blocks, each lasting approximately 5-min. Participants took a self-timed break between blocks to reengage their attention.
shows two example trials. On each trial, participants saw a centrally located fixation cross alone (100 ms), and then accompanied by two RSVP streams, one in the left and one in the right visual field. Both streams comprised 17 items serially presented for 100 ms each (for 1700 ms total). One of the two streams always contained a rotated target image: participants had to indicate the target’s rotation (left, right) by pressing the left/right arrow key at the end of each trial. To maintain task motivation, a “bell” sounded on correct answers. We did not specify what hand or fingers participants should use to make their response, which triggered the start of the next trial. Thus, the inter-trial interval was determined by how long participants took to make their response (Experiment 1a: M = 560 ms, SD = 452 ms; Experiment 1b: M = 539 ms, SD = 637 ms).
Figure 1. Examples of 6 (out of 17) frames from a Lag 2 and Lag 4 trial in Experiment 1a–1b. Note. Each frame appeared for 100 ms. In the lag 2 example trial, a negative distractor appears two items before a left-side target (rotated to the right). In the lag 4 example trial, a neutral distractor appears four items before a right-side target (rotated to the left). For copyright reasons, the images included in this schematic are from the authors collections and were not used in the study.
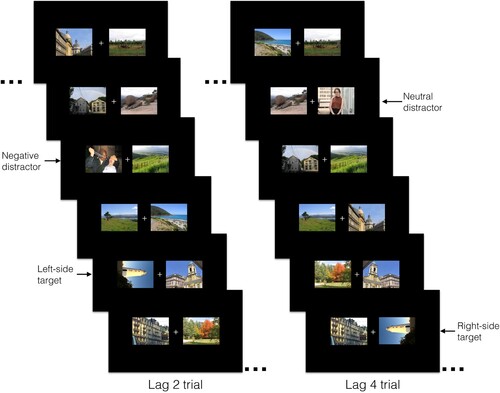
Our interest was in measuring whether distractor type (negative, neutral) changed whether participants were aware of the target image – and therefore correctly identified which way the image was rotated. Awareness of stimuli is better captured by accuracy than response time (Goodhew & Edwards, Citation2022). Hence, consistent with prior studies using EIB and attentional blink tasks, we focused on the accuracy with which participants detected the rotation of the target image, not how fast participants made these judgments. To prevent participants sacrificing accuracy for speed, we did not ask them to respond as quickly as possible. Although we did not analyse response time data, the raw data is publicly available (https://osf.io/xzs9w/files/).
The distractor appeared either two (lag 2) or four (lag 4) items before the target – see . The distractor and target were never the first or the last item in the stream: the distractor appeared in serial position 3–7, and the target appeared in serial position 5–11, depending on the trial and the lag. Therefore, of the 17 image pairs presented on each trial, one contained the target, one could contain a distractor (negative, neutral), and 15 were fillers. The target and distractor images were not paired, but rather randomly selected (at equal frequency) on each trial. There were 352 trials in total (four blocks of 88 trials). Each block included 32 trials with negative distractors (16 per lag), 32 trials with neutral distractors (16 per lag) and 24 trials without distractors – termed baseline trials. Baseline trials (that had 16 fillers, 1 distractor) allowed us to compare accuracy in the left vs. right stream on trials with vs. without distractors. There were fewer baseline trials (in total) than distractor trials, because trials without distractors have no lag properties. In each block, the target (and distractor) appeared an equal number of times in the left and right stream. After the experiment, participants rated their PTSD symptoms (via the PCL-5; Weathers et al., Citation2013). Debriefing procedures concluded the 35-min session.
Results and discussion
We first ran a 2 (distractor presence: present, absent) x 2 (target stream: left, right) repeated measures ANOVA. Although not pre-registered, this analysis allowed us to determine whether participants showed better target detection in the left (than right) stream overall, and whether this leftward advantage occurred both on trials where distractors were present and when they were absent (i.e. baseline trials). Participants were more accurate on baseline than distractor trials; a main effect of distractor presence, F(1, 61) = 48.13, p < .001, ŋp2 = .44. As hypothesised, participants were more accurate for left than right stream targets, demonstrated by a main effect of target stream, F(1, 61) = 18.63, p < .001, ŋp2 = .23. This leftward advantage occurred on distractor and baseline trials (see ), demonstrated by no significant interaction between distractor presence and target stream, F(1, 61) = 0.29, p = .594, ŋp2 = .005.
Table 1. Descriptive statistics (M[SD]) for target detection accuracy depending on distractor presence and target stream: Experiments 1a and 1b.
To test our remaining hypotheses, we ran a 2 (distractor/target stream: left, right) x 2 (distractor valence: negative, neutral) x 2 (lag: 2, 4) repeated-measures ANOVA on distractor trials only (see ). As predicted, participants were less accurate (%) in detecting the rotation of the target image when it was preceded by a negative distractor (M = 60.06, SD = 6.54) than a neutral distractor (M = 63.81, SD = 6.23), demonstrating EIB, F(1, 61) = 18.59, p < .001, ŋp2 = .23. Participants were more accurate at lag 4 (M = 63.87, SD = 6.81) than lag 2 (M = 59.99, SD = 5.44), F(1, 61) = 26.06, p < .001, ŋp2 = .30. Replicating the aforementioned leftward advantage in target detection, there was a main effect of distractor/target stream, F(1, 61) = 22.82, p < .001, ŋp2 = .27. There was no interaction between distractor/target stream and lag (F(1, 61) = 0.08, p = .782, ŋp2 = .001), suggesting there were no visual field differences in how long the distractors disrupted target detection. There was also no interaction between valence and lag (F(1, 61) = 0.02, p = .887, ŋp2 = .000), suggesting horizontal streams give rise to a similar sized EIB effect at lags 2 and 4. Finally, counter to our primary hypothesis, there was a similar-sized EIB effect in the left and right stream demonstrated by no interaction between distractor/target stream and valence (F(1, 61) = .001, p = .977, ŋp2 = .000) and no three-way interaction between valence, lag and distractor/target stream (F(1, 61) = 0.23, p = .636, ŋp2 = .004). Therefore, although participants were more accurate when the distractor and target appeared in the left than the right stream, the relative difference in accuracy following negative vs. neutral distractors was similar in both streams.
Figure 2. Mean accuracy for correctly detecting the target rotation depending on distractor valence, lag, and the distractor/target stream in Experiment 1a. Note. Error bars represent 95% within-subject confidence intervals (Masson & Loftus, Citation2003). Dotted line represents mean baseline accuracy (i.e. on trials without distractors) and shaded area represents 95% confidence interval around baseline accuracy.
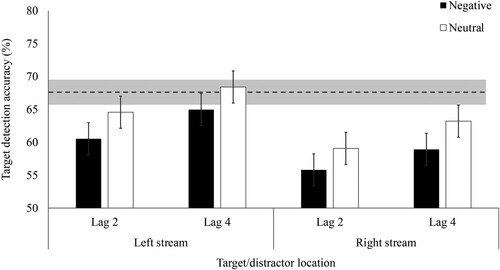
Experiment 1b
To establish converging evidence for the results of Experiment 1a, we ran a direct replication – Experiment 1b (pre-registration: https://osf.io/um95t).
Method
Participants. We collected data from 65 participants but excluded four; one who was not strongly right-handed, two whose overall accuracy was 2SDs below the rest of the sample ([M = 63.61, SD = 6.56] collapsed across all trials), and one who had below chance accuracy on baseline trials. Our final sample of 61 participants ranged from 18 to 51 years old (M = 23.1, 95% CI [21.3, 25.0]), were predominantly women (72%; 26% men; 1% non-binary), strongly right-handed (M = + 9.92, 95% CI [+9.82, + 10.00], range = + 8 to +10 on the FLANDERS), and had normal or corrected-to-normal vision.
Results and discussion
Our analyses matched Experiment 1a. We first ran a 2 (distractor presence: present, absent) x 2 (target stream: left, right) repeated measures ANOVA to confirm we replicated the leftward advantage from Experiment 1a. We did; participants showed a leftward advantage on baseline and distractor trials (see ), shown by a main effect of target stream (F(1, 60) = 14.36, p < .001, ŋp2 = .19), a main effect of distractor presence (F(1, 60) = 49.53, p < .001, ŋp2 = .45), and no interaction between distractor presence and target stream (F(1, 60) = 0.32, p = .576, ŋp2 = .005).
We then ran a 2 (distractor/target stream: left, right) x 2 (distractor valence: negative, neutral) x 2 (lag: 2, 4) repeated measures ANOVA (see ). As in Experiment 1a, participants exhibited EIB: they were less accurate (%) following negative (M = 60.55, SD = 6.33) than neutral (M = 65.57, SD = 6.84) distractors, F(1, 60) = 38.53, p < .001, ŋp2 = .39. Participants were less accurate overall at lag 2 (M = 61.37, SD = 5.51) than lag 4 (M = 64.75, SD = 7.36), F(1, 60) = 19.63, p < .001, ŋp2 = .25. Finally, participants were more accurate when the distractor and target appeared in the left than the right stream, F(1, 60) = 16.58, p < .001, ŋp2 = .22. Also similar to Experiment 1a, there was no three-way interaction between valence, lag and distractor/target stream (F(1, 60) = .56, p = .455, ŋp2 = .009), nor two way interactions between distractor/target stream and lag (F(1, 60) = .94, p = .336, ŋp2 = .015) or between valence and lag (F(1, 60) = .08, p = .780, ŋp2 = .001).
Figure 3. Mean accuracy for correctly detecting the target rotation depending on distractor valence, lag, and the distractor/target stream in Experiment 1b. Note. Error bars represent 95% within-subject confidence intervals (Masson & Loftus, Citation2003). Dotted line represents mean baseline accuracy (i.e. on trials without distractors) and shaded area represents 95% confidence interval around baseline accuracy.
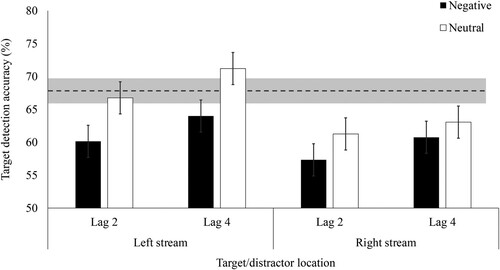
However, counter to Experiment 1a, we found the hypothesised interaction between distractor/target stream and valence, F(1, 60) = 6.43, p = .014, ŋp2 = .10. Post hoc tests with Holm correctionFootnote4 suggested a larger EIB effect in the left stream (negative: M = 62.07, SD = 8.34, neutral: M = 68.98, SD = 8.58, t(60) = −6.28, p < .001, dz = 0.80) than the right stream (negative: M = 59.04, SD = 8.01, neutral: M = 62.17, SD = 9.45, t(60) = −2.84, p = .016, dz = 0.36). However, we only found visual field differences following neutral (t(60) = 4.80, p < .001, dz = 0.61) and not negative (t(60) = 2.13, p = .072, dz = 0.27) distractors. In other words, negative distractors disrupted target detection to a similar extent in both streams.
In Experiments 1a and 1b, EIB occurred in both the left and the right visual fields – participants were consistently less accurate in detecting the target’s rotation following negative than neutral distractors. Although overall target detection was better at lag 4 than lag 2, a similar sized EIB effect occurred at both lags. This result is inconsistent with other studies (e.g. Kennedy & Most, Citation2015) that have reported a stronger EIB effect at lag 2 than lag 4. In line with a leftward attention bias, participants were more accurate in detecting the rotation of left than right stream targets both when distractors were present and when they were absent. Taken alongside existing work (Mulckhuyse et al., Citation2017; Nicholls et al., Citation2017), this finding suggests greater activation in right- than left-hemisphere regions for visuospatial attention (Karolis et al., Citation2019) leads to superior detection of targets when they appear in the left, than the right, visual field.
We found mixed support for our main hypothesis that EIB would be larger in the left than the right visual field. This hypothesis was not supported in Experiment 1a but was partially supported in Experiment 1b. Although the difference in accuracy following negative relative to neutral distractors was larger in the left stream (dz = 0.80) than the right stream (dz = 0.36) in Experiment 1b, post hoc tests revealed accuracy following negative distractors was similar in the left and right streams. In other words, it was superior target detection following neutral distractors that appeared to drive the larger EIB effect in the left stream. These findings provide preliminary evidence that emotional distractors disrupt target detection to a similar extent in the left and the right visual field.
However, always having the distractor and target appear in the same stream as one another is a sub-optimal test of whether emotional processing asymmetries affect EIB: if participants noticed this relationship, they could have simply used the distractor as a cue to the target’s location (e.g. Mackintosh, Citation1975). Our Experiment 1b data suggests some participants noticed this relationship – when there were four items between the distractor and target (i.e. lag 4), target detection in the left stream was better following neutral distractors than on no distractor trials (t(60) = −2.81, p = .007). To address this methodological limitation, we ran Experiments 2a (and 2b) in which the distractor and target could appear in the same or the opposite stream as each other. In addition to providing a more valid test of whether EIB is exacerbated in the left than the right visual field, these experiments allowed us to determine whether EIB is spatially localised with horizontally aligned streams, as it is with vertical streams (e.g. Most & Wang, Citation2011).
Experiment 2a
In Experiment 2a (and 2b) the distractor appeared in either the same stream as the target (i.e. both in the left visual field or both in the right visual field) or the opposite stream (e.g. distractor in the left and target in the right visual field, or vice versa). We used a 2 (target stream: left, right) x 2 (distractor stream: left, right) x 2 (distractor valence: negative, neutral) repeated measures design, replacing the lag manipulation with the distractor stream manipulation (i.e. varying the distractor-target relationship). In these experiments, the distractor only ever occurred two items before the target (lag 2). Using only one type of lag allowed us to keep the same number of within-subject factors as Experiment 1a–1b, and maximise the number of trials in each cell. We chose lag 2, rather than lag 4, because EIB is highly reliable at lag 2 (Onie & Most, Citation2017) and to be consistent with vertical dual-stream EIB studies (Kennedy et al., Citation2018; Most & Wang, Citation2011; Wang & Most, Citation2016). Notably, the degree of EIB at lag 2 is similar when other lags are present (e.g. Kennedy & Most, Citation2015) or absent (e.g. Most et al., Citation2010) in the design.
One aim of Experiments 2a–2b was to establish if EIB is spatially localised when the dual-streams are aligned horizontally, as has been found with vertically aligned streams (e.g. Kennedy et al., Citation2018; Most & Wang, Citation2011; Wang & Most, Citation2016). Findings from attentional blink studies provide grounds to expect EIB might not be spatially localised with horizontal streams. Unlike existing dual-stream EIB experiments, dual-stream attention blink experiments often include horizontally aligned streams (Asanowicz et al., Citation2013; Holländer et al., Citation2005; Śmigasiewicz et al., Citation2010; Verleger et al., Citation2009). In a dual-stream attentional blink paradigm, participants have to report two targets (e.g. target 1 [T1]: a different coloured letter, target 2 [T2]: a number) presented among streams located in the left and right visual field (Holländer et al., Citation2005). While there are generally no visual field differences in detecting T1, accuracy in identifying T2 is 20–30% better when it appears in the left than the right visual field (see Verleger & Śmigasiewicz, Citation2015 for review). This left side T2 advantage is larger when the two targets appear in opposite visual fields compared to the same visual field – i.e. the effect is not spatially localised. The left side advantage for identifying T2, particularly when T1 appears in the right visual field, is attributed to a right-hemisphere advantage in directing attention to salient events (e.g. Smigasiewicz et al., Citation2014) – termed attentional orienting. Due to this orienting asymmetry, the “default” setting for detecting salient visual stimuli in rapidly presented streams is toward the left side of space (Verleger & Śmigasiewicz, Citation2015). Thus, people can shift attention from the right to the left faster than from the left to the right visual field (Moscovitch, Citation1986), resulting in a strong and reliable left side advantage with simultaneous monitoring of dual-streams. This left side advantage is particularly likely when target location is uncertain and both visual fields monitored simultaneously (e.g. Corbetta et al., Citation2008).
Both attentional blink and EIB paradigms require monitoring rapid streams that contain two critical stimuli (T1 and T2 in attentional blink, the distractor and target in EIB). But there are differences between the paradigms, such as T1 being task relevant vs. the distractor being task irrelevant, using simple (e.g. letters and numbers) vs. more complex (e.g. images) stimuli, and the absence vs. presence of emotion, respectively. Therefore, although attentional blink findings led us to expect a larger leftward advantage in target detection when the distractor appeared in the opposite location to the target (i.e. in the right stream) than when it appeared in the same location (i.e. also in the left stream), we predicted that distractor valence would alter this effect. This prediction would be supported by a three-way interaction between target stream, distractor stream and distractor valence. Specifically, we expected no difference in target detection by valence (i.e. no EIB) on trials when the distractor appeared in the right and the target appeared in the left stream. By contrast, we expected to see EIB on trials when the distractor appeared in the left and the target appeared in the right stream.
Method
Participants. In Experiment 2a (pre-registration: https://osf.io/4a6fg/), we recruited 66 university students but excluded five; three who were not sufficiently right-handed (two were excluded before starting the EIB task) and two whose accuracy was below chance. The remaining 61 participants were aged between 18 and 42 years (M = 21.0, 95% CI [20.1, 21.8]), had normal or corrected-to-normal vision, were strongly right-handed (M = + 9.68, 95% CI [+9.85, + 10.00], range = + 8.00 to +10.00 on the FLANDERS), and were predominantly women (85%; 15% men; 0% non-binary).
Procedure. Our procedure matched Experiments 1a and 1b (DASS-21 and FLANDERS, the experiment, then the PCL-5), except for the changes to distractor location and lag. The distractor appeared in the same stream as the target on half the trials and in the opposite stream as the target on the other half, in a randomised order. In each block, the target and distractor appeared an equal number of times in the left and right visual field. The inter-trial interval – determined by the time it took participants to respond – was similar to Experiments 1a–1b (Experiment 2a: M = 563 ms, SD = 498; Experiment 2b: 538 ms, SD = 850 ms).
Results and discussion
We first tested whether participants showed superior target detection when the target appeared in the left than the right stream using the same 2 (distractor presence: present, absent) x 2 (target stream: left, right) repeated measures ANOVA as Experiments 1a and 1b (see ). Consistent with Experiments 1a and 1b, participants were more accurate on baseline than distractor trials; a main effect of distractor presence, F(1, 60) = 49.71, p < .001, ŋp2 = .11, and showed an overall leftward advantage in target detection accuracy; a main effect of target stream, F(1, 60) = 7.44, p = .008, ŋp2 = .11. However, contrary to Experiments 1a and 1b we found a significant distractor x target stream interaction, F(1, 60) = 9.60, p = .003, ŋp2 = .14. A post hoc test with Holm correction showed no difference in target detection accuracy in the left and the right stream when distractors were present (t(60) = 1.06, p = .47, dz = 0.49) but a leftward advantage when distractors were absent (t(60) = 3.82, p < .001, dz = 0.14). The leftward advantage on baseline trials replicates Experiment 1a and 1b.
Table 2. Descriptive statistics (M(SD)) for target detection accuracy depending on distractor presence and target stream: Experiments 2a and 2b.
We next ran the main 2 (target stream: left, right) x 2 (distractor stream: left, right) x 2 (distractor valence: negative, neutral) repeated measures ANOVA (see ). Participants showed EIB; they were less accurate (%) following negative (M = 59.34, SD = 6.28) than neutral (M = 63.93, SD = 6.89) distractors, a main effect of distractor valence, F(1, 60) = 26.50, p < .001, ŋp2 = .31. Accuracy was similar when the distractor appeared in the left (M = 61.03, SD = 6.33) or the right (M = 62.23, SD = 6.27) stream, as reflected by no main effect of distractor stream, F(1, 60) = 1.50, p = .108, ŋp2 = .04. Inconsistent with Experiments 1a and 1b and our hypothesis, there was no main effect of target stream, F(1, 60) = 1.50, p = .225, ŋp2 = .02.
Figure 4. Mean accuracy for correctly detecting the target rotation depending on distractor valence, distractor stream, and target location in Experiment 2a. Note. Error bars represent 95% within-subject confidence intervals (Masson & Loftus, Citation2003). Dotted line represents mean baseline accuracy (i.e. on trials without distractors) and shaded area represents 95% confidence interval around baseline accuracy.
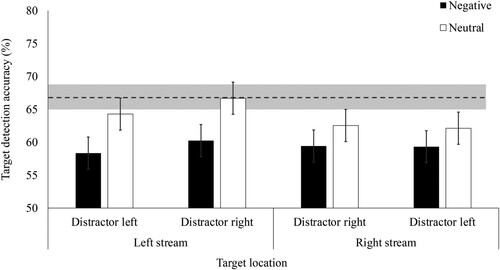
Contrary to EIB being spatially localised, accuracy was similarly impaired following negative relative to neutral distractors, regardless of whether the distractor and target appeared in the same (negative = 58.89, SD = 7.35, neutral = 63.43, SD = 7.64; t(60) = 4.11, p < .001, dz = 0.53, 95% CI [0.26, 0.79]) or opposite (negative = 59.79, SD = 8.12, neutral = 64.42, SD = 8.27; t(60) = 3.91, p < .001, dz = 0.50, 95% CI [0.23, 0.77]) stream. Counter to our hypotheses, we found no three-way interaction between target stream, valence, and distractor stream (F(1, 60) = .005, p = .944, ŋ2 = .000). There was also no interaction between distractor stream and valence (F(1, 60) = .10, p = .750, ŋp2 = .002) or distractor and target stream (F(1, 60) = 1.33, p = .253, ŋp2 = .22). There was a marginal but non-significant interaction between target stream and valence, F(1, 60) = 4.00, p = .050, ŋp2 = .06, which we sought to replicate in Experiment 2b.
Experiment 2b
Experiment 2b directly replicated Experiment 2a (pre-registration: http://osf.io/586jx).
Method
Participants. We recruited 68 participants but excluded seven; three who were not strongly right-handed (who did not begin the EIB task), two whose overall accuracy was more than 2SD lower than the sample mean (M = 61.16, SD = 6.38 collapsed across all trials), and two who had below chance accuracy on baseline trials. The remaining 61 participants were 18–38 years of age (M = 21.67, 95% CI [20.46, 22.88]), strongly right-handed (M = + 9.86, 95% CI [+9.76, + 9.97], range = + 8.00 to +10.00 on the FLANDERS) and predominantly women (80%; 20% men; 0% non-binary).
Results and discussion
Our analyses matched Experiment 2a. We first ran a 2 (distractor presence: present, absent) x 2 (target stream: left, right) repeated measures ANOVA (see ). Consistent with Experiment 2a, we found main effects of distractor presence (F(1, 60) = 51.74, p < .001, ŋp2 = .46) and target stream (F(1, 60) = 18.33, p < .001, ŋp2 = .23), and a significant distractor presence x target stream interaction, F(1, 60) = 7.55, p = .008, ŋp2 = .11. However, inconsistent with Experiment 2a, the leftward advantage in target detection accuracy occurred on trials both with (t(60) = 5.02, p < .001, dz = 0.64) and without distractors (t(60) = 2.83, p = .01, dz = 0.36). The leftward advantage was larger on baseline than distractor trials.
We next ran a 2 (target stream: left, right) x 2 (distractor stream: left, right) x 2 (distractor valence: negative, neutral) repeated measures ANOVA (see ). Consistent with Experiment 2a, participants were less accurate (%) following negative (M = 58.71, SD = 5.48) than neutral (M = 62.26, SD = 6.40) distractors; a main effect of valence demonstrating EIB, F(1, 60) = 24.75, p < .001, ŋp2 = .29. Target detection accuracy was similar when the distractor appeared in the left (M = 60.02, SD = 5.58) and the right (M = 60.95, SD = 6.67) stream; there was no main effect of distractor stream, F(1, 60) = 1.32, p = .255, ŋp2 = .02. Inconsistent with Experiment 2a, but consistent with Experiment 1a and 1b, we found the aforementioned leftward advantage demonstrated by a main effect of target stream, F(1, 60) = 5.15, p = .027, ŋp2 = .08.
Figure 5. Mean accuracy for correctly detecting the target rotation depending on distractor valence, distractor stream, and target location in Experiment 2b. Note. Error bars represent 95% within-subject confidence intervals (Masson & Loftus, Citation2003). Dotted line represents mean baseline accuracy (i.e. on trials without distractors) and shaded area represents 95% confidence interval around baseline accuracy.
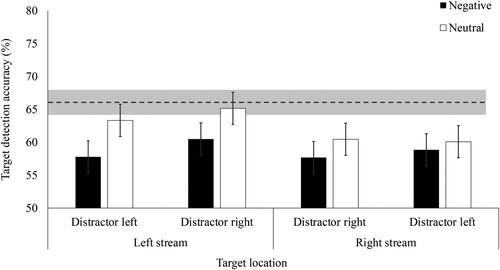
Consistent with Experiment 2a, accuracy was worse following negative than neutral distractors when the distractor and target appeared in the same (negative: M = 57.74, SD = 6.50, neutral: M = 61.89, SD = 7.40; t(60) = 3.68, p < .001, dz = 0.47, 95% CI [0.20, 0.73]) and the opposite (negative: M = 59.68, SD = 7.69, neutral: M = 62.63, SD = 7.91; t(60) = 2.71, p = .009, dz = 0.35, 95% CI [0.09, 0.60]) stream. The lack of three-way interaction between distractor stream, target stream and valence (F(1, 60) = .50, p = .480, ŋp2 = .008) replicates the non-spatially localised EIB effect from Experiment 2a. Also similar to Experiment 2a, there were no two-way interactions between distractor stream and valence (F(1, 60) = .05, p = .833, ŋp2 = .001) or distractor and target stream (F(1, 60) = 3.34, p = .072, ŋp2 = .05). We found an interaction between target stream and valence, supporting the marginal interaction between these two variables from Experiment 2a, F(1, 60) = 4.76, p = .033, ŋp2 = .07. Post hoc tests with Holm correction revealed that EIB occurred in the left (negative: M = 59.14, SD = 7.36; neutral: M = 64.24, SD = 8.66, t(60) = 5.06, p < .001, dz = 0.65), but not the right (negative: M = 58.27, SD = 7.69; neutral: M = 60.27, SD = 7.33, t(60) = 1.99, p = .148, dz = 0.25) stream. However, this difference was driven by worse accuracy for right than left stream targets following neutral (t(60) = 3.10, p = .01, dz = 0.40) distractors. There was no visual field difference in target detection following negative distractors (t(60) = .68, p = .763, dz = 0.09); a pattern consistent with Experiment 1b.
In Experiment 2b, the impairment following negative relative to neutral distractors was larger for left than right stream targets, demonstrated by the target stream-valence interaction (which was marginal in Experiment 2a). One explanation for this effect is that the tendency to attend more to the left increases people’s susceptibility to all distractors presented on the left. This explanation is supported by prior work. For example, participants’ ability to report a single red target letter presented amongst 20 different coloured letters in a central stream is impaired more by distractors that appeared to the left than the right of the central stream (at lag 2; Du & Abrams, Citation2010). Further, in a vertical dual-stream EIB task participants showed worse target detection accuracy when the distractors were located in the stream to which they fixated (Kennedy et al., Citation2018). We know – from the leftward advantage on baseline trials – that participants were attending more to the left than the right stream overall. But if this explanation were correct, then people should have been more impaired by negative distractors when they appeared on the left than the right, which was not the case.
Rather, the detection of left stream targets was similarly disrupted by negative distractors when those distractors appeared in the left or the right stream. To confirm this explanation, we ran two exploratory Bayesian paired samples t-tests on the Experiment 2b data using the default Cauchy prior (Rouder et al., Citation2009). We interpret the results of these t-tests according to Wetzels et al. (Citation2011), who describe Bayes factor categories ranging from no to decisive evidence for the null/alternative hypotheses. We first compared left-right (target) stream accuracy following neutral distractors and found BF10 = 13.14, indicating strong evidence for the alternative hypothesis of a visual field difference (Wetzels et al., Citation2011). We then compared left-right (target) stream accuracy following negative distractors and found BF10 = 0.17, indicating substantial support for the null hypothesis of no visual field difference (Wetzels et al., Citation2011). Therefore, the most plausible explanation for the target stream-valence interaction is a consistent impairment in target detection following negative distractors in both streams, coupled with a leftward advantage following neutral distractors.
General discussion
Task irrelevant emotional distractors preferentially engage people’s attention, impairing their ability to detect task-relevant neutral targets. This effect is known as emotion-induced blindness (EIB). Here, we wondered whether the extent to which emotional distractors disrupt attention depends on which visual field the distractors and targets appear in. We thought it might, based on visuospatial attention asymmetries and proposed emotional processing asymmetries. We found that visual field differences in EIB depended on whether the target and distractor had a predictable (Experiments 1a–1b) or unpredictable (Experiments 2a–2b) relationship. When the distractors and targets always appeared in the same visual field as one another – a predictable relationship – we found a similar degree of EIB in the left and right visual field. When the distractors and targets could appear in the same or opposite visual field as one another – an unpredictable relationship – we found stronger EIB in the left than the right visual field. However, this effect was driven by better left-side target detection following neutral distractors, coupled with similarly impaired target detection following negative distractors. Together, these findings suggest emotional stimuli disrupt target detection regardless of which visual field the emotional stimuli, or the neutral targets, appear.
These findings extend understanding of the circumstances in which hemispheric asymmetries in visuospatial attention affect behaviour. Based on right-hemisphere asymmetries in visuospatial attention (e.g. Karolis et al., Citation2019), we predicted better detection of left than right visual field targets. In line with this prediction, participants were consistently better at detecting targets that appeared in the left than the right visual field. This leftward advantage was particularly reliable on trials without distractors and following neutral distractors. The consistent leftward advantage on trials without distractors fits with people being better at finding targets that appear on the left than the right side of a display (Mulckhuyse et al., Citation2017; Nicholls et al., Citation2017). The similarly consistent leftward advantage on neutral distractor trials fits with people being more accurate in detecting the second target when it appears in the left than the right visual field in attentional blink (e.g. Asanowicz et al., Citation2012). Therefore, right-hemisphere asymmetries in visuospatial attention lead to a leftward advantage in stimuli detection, even when these stimuli rapidly appear.
Our findings suggest emotional stimuli disrupt this leftward advantage. On negative distractor trials in Experiments 2a and 2b, participants were no better at detecting targets that appeared in the left or the right visual field. This finding aligns with people showing similar steady-state visual evoked potential amplitude – a neural marker of attention allocation – when emotional distractors appear in the left or right visual field, regardless of which visual field they are attending (Bekhtereva et al., Citation2021). Together with Bekhetereva et al.’s EEG evidence, our behavioural evidence supports the view that emotional stimuli disrupt spatial attention. However, our findings suggest this disruption only occurs when the emotional and target stimuli have an unpredictable relationship. In Experiments 1a and 1b, when the distractor-target relationship was predictable, participants showed a leftward advantage on both neutral and negative distractor trials. With a predictable distractor-target relationship, the distractor might have inadvertently cued the target location, particularly on lag 4 trials where there was 400 ms between the distractor and target. This cueing effect could have allowed participants to partially overcome the impairment caused by negative distractors, maintaining the leftward advantage.
Recall our secondary aim to advance understanding of emotional processing asymmetries. Broadly speaking, our findings suggest emotional processing asymmetries have limited influence on the processing of task-irrelevant emotional stimuli. This finding counters traditional models of emotional processing asymmetries, such as the valence (Davidson & Fox, Citation1982) and right-hemisphere (Gainotti, Citation1972) hypotheses. According to these traditional models, because of right-hemisphere specialisation in processing negative emotion, we should have seen a visual field difference on negative distractor trials. Although we observed a greater impairment following negative relative to neutral distractors in the left than the right stream in Experiments 2a–2b – i.e. a larger EIB effect in the left stream – this effect was driven by the leftward advantage following neutral distractors, coupled with consistent (worse) accuracy in both visual fields following negative distractors. Therefore, our findings do not support the relatively clean emotional processing asymmetries proposed by traditional models, which have also not been supported by research showing no left visual field advantage in detecting emotional stimuli (Carretié et al., Citation2019). The fact that distractor-target relationship affected whether or not participants showed a leftward advantage following negative distractors supports the criticism of traditional models for not taking into account cognition–emotion interactions (Grimshaw & Carmel, Citation2014; Ocklenburg et al., Citation2017).
Taken alongside other research, our findings suggest the influence of emotional processing asymmetries on behaviour may depend on whether emotion is relevant to the task (Grimshaw & Carmel, Citation2014). As demonstrated in these experiments using an EIB paradigm, and by Ocklenburg et al. (Citation2017) using a lateralised No-Go/Go task, when distractors are task irrelevant, there seems to be minimal influence of emotional processing asymmetries on behavioural responses. When distractors are task relevant, however, emotional processing asymmetries may affect behavioural responses (e.g. Mulckhuyse et al., Citation2017). As Ocklenburg et al. (Citation2017) echo, this potential interaction between task relevance and hemispheric processes may be crucial in predicting if and how hemispheric asymmetries influence behaviour. In line with this view, emotional processing asymmetries appear to influence responses on behavioural tasks that require explicit judgments of the emotional stimuli, such as recognising emotional vs. neutral scenes (Moeck et al., Citation2020) and discriminating whether a peripherally presented scene is emotional or not (Calvo et al., Citation2015). Future research could examine the role of task relevance by using a horizontal EIB paradigm but varying whether the distractors are task relevant or not, for example by testing (or not testing) participant’s memory for the distractor images at the end of each block of trials (as in Kennedy et al., Citation2018).
These findings extend understanding of EIB, because in EIB tasks, researchers have previously used only a single central stream or two vertically-aligned streams. We found that with horizontally-aligned streams, EIB emerged both in the right and left visual fields and at both lag 2 and lag 4 (Experiment 1a–1b). This consistency across visual fields and lags underscores the robustness of EIB, an effect that is not restricted to central or vertically placed streams. EIB occurred regardless of whether the distractor and target appeared in the same or the opposite stream as one another (Experiment 2a–2b), suggesting that with horizontal streams, EIB may not be spatially localised. Work by Proud et al. (Citation2020) found no spatial localisation with vertical dual-streams. Thus, the view that EIB only occurs when the emotional and neutral stimuli appear in the same location as one another (as summarised by Wang et al., Citation2012) should be further tested.
These experiments have limitations. First, like most dual-stream EIB studies (except Proud et al., Citation2020), we only used negative and not positive distractors. Using only negative distractors limits the conclusions that can be drawn for emotional processing theories that distinguish between positive and negative emotion (Davidson & Fox, Citation1982) or between approach and avoidance motivations (Harmon-Jones, Citation2003). Second, we relied on recruiting strong right-handers to increase the likelihood that participants had greater right than left-hemisphere activation for visuospatial attention (e.g. Zago et al., Citation2017). However, eye-dominance may be a better predictor than handedness of hemispheric biases in visuospatial attention (Schintu et al., Citation2020). Finally, we did not eye-track our participants, meaning we cannot confirm that participants maintained central fixation. However, instructing participants to maintain central fixation is sufficient in the majority of healthy participants (e.g. Moscovitch, Citation1986) and similar left-right differences are found in the attentional blink when fixation is vs. is not controlled (Verleger et al., Citation2009).
Emotional stimuli alert us to potential environmental threats, increasing our vigilance and capacity for survival. But our reflexive response to emotion comes at a cost; it robustly impairs our ability to notice potentially important, but neutral, environmental stimuli. These experiments demonstrate that this impairment occurs in the right and left visual field and appears not to be spatially localised across a horizontal field of view. When the locations of emotional and neutral (target) stimuli are uncertain, as they often are in everyday life, people are no better at recovering from emotional distraction in the left or the right visual field.
Acknowledgments
Experiments conceptualised by EKM with assistance from MKTT, SBM and NAT. Experiments programmed by JLZ. Data collected and analysed by EKM. Manuscript draft written by EKM with critical revisions from JLZ, SBM and MKTT. All authors approved the final version of the manuscript.
Disclosure statement
No potential conflict of interest was reported by the author(s).
Additional information
Funding
Notes
1 These leftward attention bias effects are reduced, but not reversed, in people who read right-to-left (e.g. Śmigasiewicz et al., Citation2010).
2 We recruited only right-handers, to ensure participants had leftward visuospatial attention asymmetries (e.g. Zago et al., Citation2017).
3 Exploratory analyses confirmed the congruence/incongruence between the left/right rotation of the target and the visual field in which it appeared did not affect the results.
4 We used Holm correction because it has a lower Type II error rate, and the same Type I error rate, as Bonferroni correction (Navarro et al., Citation2019).
References
- Anderson, A. K. (2005). Affective influences on the attentional dynamics supporting awareness. Journal of Experimental Psycholology: General, 134(2), 258–281. https://doi.org/10.1037/0096-3445.134.2.258
- Asanowicz, D., Marzecová, A., Jaśkowski, P., & Wolski, P. (2012). Hemispheric asymmetry in the efficiency of attentional networks. Brain and Cognition, 79(2), 117–128. https://doi.org/10.1016/j.bandc.2012.02.014
- Asanowicz, D., Śmigasiewicz, K., & Verleger, R. (2013). Differences between visual hemifields in identifying rapidly presented target stimuli: Letters and digits, faces, and shapes. Frontiers in Psychology, 4, 1-13. https://doi.org/10.3389/fpsyg.2013.00452
- Bekhtereva, V., Craddock, M., & Müller, M. M. (2021). Emotional content overrides spatial attention. Psychophysiology, 58(8), 1–14. https://doi.org/10.1111/psyp.13847.
- Bourne, V. J. (2006). The divided visual field paradigm: Methodological considerations. Laterality: Asymmetries of Body, Brain and Cognition, 11(4), 373–393. https://doi.org/10.1080/13576500600633982
- Bowers, D., & Heilman, K. M. (1980). Pseudoneglect: Effects of hemispace on a tactile line bisection task. Neuropsychologia, 18(4–5), 491–498. https://doi.org/10.1016/0028-3932(80)90151-7
- Brainard, D. H. (1997). The psychophysics toolbox. Spatial Vision, 10(4), 433–436. https://doi.org/10.1163/156856897X00357
- Calvo, M. G., Rodríguez-Chinea, S., & Fernández-Martín, A. (2015). Lateralized discrimination of emotional scenes in peripheral vision. Experimental Brain Research, 233(3), 997–1006. https://doi.org/10.1007/s00221-014-4174-8
- Carretié, L. (2014). Exogenous (automatic) attention to emotional stimuli: A review. Cognitive, Affective, & Behavioral Neuroscience, 14(4), 1228–1258. https://doi.org/10.3758/s13415-014-0270-2
- Carretié, L., Méndez-Bértolo, C., Bódalo, C., Hernández-Lorca, M., Fernández-Folgueiras, U., Fondevila, S., & Giménez-Fernández, T. (2019). Retinotopy of emotion: Perception of negatively valenced stimuli presented at different spatial locations as revealed by event-related potentials. Human Brain Mapping, 41(7), 1711–1724. https://doi.org/10.1002/hbm.24904
- Ciesielski, B. G., Armstrong, T., Zald, D. H., & Olatunji, B. O. (2010). Emotion modulation of visual attention: Categorical and temporal characteristics. PLoS ONE, 5(11), 11. https://doi.org/10.1371/journal.pone.0013860
- Corbetta, M., Patel, G., & Shulman, G. L. (2008). The reorienting system of the human brain: From environment to theory of mind. Neuron, 58(3), 306–324. https://doi.org/10.1016/j.neuron.2008.04.017
- Davidson, R. J., & Fox, N. A. (1982). Asymmetrical brain activity discriminates between positive and negative affective stimuli in human infants. Science, 218(4578), 1235–1237. JSTOR. https://doi.org/10.1126/science.7146906
- de Schotten, M. T., Dell’Acqua, F., Forkel, S., Vergani, F., Murphy, D. G. M., & Catani, M. (2011). A lateralized brain network for visuospatial attention. Nature Neuroscience, 14(10), 1245–1246. https://doi.org/10.1038/nn.2905
- Du, F., & Abrams, R. A. (2010). Visual field asymmetry in attentional capture. Brain and Cognition, 72(2), 310–316. https://doi.org/10.1016/j.bandc.2009.10.006
- Gainotti, G. (1972). Emotional behavior and hemispheric side of the lesion. Cortex, 8(1), 41–55. https://doi.org/10.1016/S0010-9452(72)80026-1
- Goodhew, S. C., & Edwards, M. (2022). Don’t look now! Emotion-induced blindness: The interplay between emotion and attention. Attention, Perception, & Psychophysics, 84(8), 2741–2761. https://doi.org/10.3758/s13414-022-02525-z
- Grimshaw, G. M., & Carmel, D. (2014). An asymmetric inhibition model of hemispheric differences in emotional processing. Frontiers in Psychology, 5, 1–7. https://doi.org/10.3389/fpsyg.2014.00489.
- Guilbert, D., Most, S. B., & Curby, K. M. (2019). Real world familiarity does not reduce susceptibility to emotional disruption of perception: Evidence from two temporal attention tasks. Cognition and Emotion, 34(3), 450–461. https://doi.org/10.1080/02699931.2019.1637333.
- Haddara, N., Ravid, J., Miller, E. L., O’Hagan, M., Caracciolo, C., & Miskovic, V. (2019). Anxious anticipation prolongs emotional interference for rapid visual detection. Emotion, 19(7), 1127–1137. https://doi.org/10.1037/emo0000521
- Harmon-Jones, E. (2003). Clarifying the emotive functions of asymmetrical frontal cortical activity. Psychophysiology, 40(6), 838–848. https://doi.org/10.1111/1469-8986.00121
- Holländer, A., Corballis, M. C., & Hamm, J. P. (2005). Visual-field asymmetry in dual-stream RSVP. Neuropsychologia, 43(1), 35–40. https://doi.org/10.1016/j.neuropsychologia.2004.06.006
- Jewell, G., & McCourt, M. E. (2000). Pseudoneglect: A review and meta-analysis of performance factors in line bisection tasks. Neuropsychologia, 38(1), 93–110. https://doi.org/10.1016/S0028-3932(99)00045-7
- Karolis, V. R., Corbetta, M., & Thiebaut de Schotten, M. (2019). The architecture of functional lateralisation and its relationship to callosal connectivity in the human brain. Nature Communications, 10(1), 1. https://doi.org/10.1038/s41467-019-09344-1
- Kennedy, B. L., & Most, S. B. (2012). Perceptual, not memorial, disruption underlies emotion-induced blindness. Emotion, 12(2), 199–202. https://doi.org/10.1037/a0026380
- Kennedy, B. L., & Most, S. B. (2015). The rapid perceptual impact of emotional distractors. PLoS ONE, 10(6), 6. https://doi.org/10.1371/journal.pone.0129320
- Kennedy, B. L., Pearson, D., Sutton, D. J., Beesley, T., & Most, S. B. (2018). Spatiotemporal competition and task-relevance shape the spatial distribution of emotional interference during rapid visual processing: Evidence from gaze-contingent eye-tracking. Attention, Perception, & Psychophysics, 80(2), 426–438. https://doi.org/10.3758/s13414-017-1448-9
- Lang, P. J., Bradley, M. M., & Cuthbert, B. N. (2005). International Affective Picture System: Technical report A-8. University of Florida.
- Lovibond, P. F., & Lovibond, S. H. (1995). The structure of negative emotional states: Comparison of the depression anxiety stress scales (DASS) with the Beck depression and anxiety inventories. Behaviour Research and Therapy, 33(3), 335–343. https://doi.org/10.1016/0005-7967(94)00075-U
- Mackintosh, N. J. (1975). A theory of attention: Variations in the associability of stimuli with reinforcement. Psychological Review, 82(4), 276–298. https://doi.org/10.1037/h0076778
- MacLeod, C., Mathews, A., & Tata, P. (1986). Attentional bias in emotional disorders. Journal of Abnormal Psychology, 95(1), 15–20. https://doi.org/10.1037/0021-843X.95.1.15
- Masson, M. E. J., & Loftus, G. R. (2003). Using confidence intervals for graphically based data interpretation. Canadian Journal of Experimental Psychology/Revue Canadienne de Psychologie Expérimentale, 57(3), 203–220. https://doi.org/10.1037/h0087426
- Mattingley, J. B., Berberovic, N., Corben, L., Slavin, M. J., Nicholls, M. E. R., & Bradshaw, J. L. (2004). The greyscales task: A perceptual measure of attentional bias following unilateral hemispheric damage. Neuropsychologia, 42(3), 387–394. https://doi.org/10.1016/j.neuropsychologia.2003.07.007
- Mavratzakis, A., Herbert, C., & Walla, P. (2016). Emotional facial expressions evoke faster orienting responses, but weaker emotional responses at neural and behavioural levels compared to scenes: A simultaneous EEG and facial EMG study. NeuroImage, 124(Pt A), 931–946. https://doi.org/10.1016/j.neuroimage.2015.09.065
- McHugo, M., Olatunji, B. O., & Zald, D. H. (2013). The emotional attentional blink: What we know so far. Frontiers in Human Neuroscience, 7, 1–9. https://doi.org/10.3389/fnhum.2013.00151.
- Moeck, E. K., Mortlock, J., Onie, S., Most, S. B., & Koval, P. (2022). Blinded by and stuck in negative emotions: Is psychological inflexibility across different domains related?. Affective Science, 3(4), 836–848. http://dx.doi.org/10.1007/s42761-022-00145-2
- Moeck, E. K., Thomas, N. A., & Takarangi, M. K. T. (2020). Lateralized processing of emotional images: A left hemisphere memory deficit. Emotion, 20(2), 236–247. https://doi.org/10.1037/emo0000554
- Moscovitch, M. (1986). Afferent and efferent models of visual perceptual asymmetries: Theoretical and empirical implications. Neuropsychologia, 24(1), 91–114. https://doi.org/10.1016/0028-3932(86)90044-8
- Most, S. B., Chun, M. M., Widders, D. M., & Zald, D. H. (2005). Attentional rubbernecking: Cognitive control and personality in emotion-induced blindness. Psychonomic Bulletin & Review, 12(4), 654–661. https://doi.org/10.3758/BF03196754
- Most, S. B., Laurenceau, J.-P., Graber, E., Belcher, A., & Smith, C. V. (2010). Blind jealousy? Romantic insecurity increases emotion-induced failures of visual perception. Emotion, 10(2), 250–256. https://doi.org/10.1037/a0019007
- Most, S. B., Smith, S. D., Cooter, A. B., Levy, B. N., & Zald, D. H. (2007). The naked truth: Positive, arousing distractors impair rapid target perception. Cognition & Emotion, 21(5), 964–981. https://doi.org/10.1080/02699930600959340
- Most, S. B., & Wang, L. (2011). Dissociating spatial attention and awareness in emotion-induced blindness. Psychological Science, 22(3), 300–305. https://doi.org/10.1177/0956797610397665
- Mulckhuyse, M., Engelmann, J. B., Schutter, D. J. L. G., & Roelofs, K. (2017). Right posterior parietal cortex is involved in disengaging from threat: A 1-Hz rTMS study. Social Cognitive and Affective Neuroscience, 12(11), 1814–1822. https://doi.org/10.1093/scan/nsx111
- Navarro, D. J., Foxcroft, D. R., & Faulkenberry, T. J. (2019). Learning Statistics with JASP. http://www.learnstatswithjasp.com.
- Nicholls, M. E. R., Hobson, A., Petty, J., Churches, O., & Thomas, N. A. (2017). The effect of cerebral asymmetries and eye scanning on pseudoneglect for a visual search task. Brain and Cognition, 111, 134–143. https://doi.org/10.1016/j.bandc.2016.11.006
- Nicholls, M. E. R., Thomas, N. A., Loetscher, T., & Grimshaw, G. M. (2013). The flinders handedness survey (FLANDERS): A brief measure of skilled hand preference. Cortex, 49(10), 2914–2926. https://doi.org/10.1016/j.cortex.2013.02.002
- Ocklenburg, S., & Güntürkün, O. (2018). The lateralized brain. Elsevier. https://doi.org/10.1016/B978-0-12-803452-1.00008-4.
- Ocklenburg, S., Peterburs, J., Mertzen, J., Schmitz, J., Güntürkün, O., & Grimshaw, G. (2017). Effects of emotional valence on hemispheric asymmetries in response inhibition. Symmetry, 9(8), 145. https://doi.org/10.3390/sym9080145
- Olatunji, B. O., Armstrong, T., McHugo, M., & Zald, D. H. (2013). Heightened attentional capture by threat in veterans with PTSD. Journal of Abnormal Psychology, 122(2), 397–405. https://doi.org/10.1037/a0030440
- Onie, S., MacLeod, C., & Most, S. B. (2022). Gone for good: Lack of priming suggests early perceptual interference in emotion-induced blindness with negative stimuli. Emotion, Advance online publication. https://doi.org/10.1037/emo0001170.
- Onie, S., & Most, S. B. (2017). Two roads diverged: Distinct mechanisms of attentional bias differentially predict negative affect and persistent negative thought. Emotion, 17(5), 884–894. https://doi.org/10.1037/emo0000280
- Palomero-Gallagher, N., & Amunts, K. (2021). A short review on emotion processing: A lateralized network of neuronal networks. Brain Structure and Function, 227, 673–684. https://doi.org/10.1007/s00429-021-02331-7.
- Pelli, D. G. (1997). The VideoToolbox software for visual psychophysics: Transforming numbers into movies. Spatial Vision, 10(4), 437–442. https://doi.org/10.1163/156856897X00366
- Prete, G., Capotosto, P., Zappasodi, F., & Tommasi, L. (2018). Contrasting hemispheric asymmetries for emotional processing from event-related potentials and behavioral responses. Neuropsychology, 32(3), 317–328. https://doi.org/10.1037/neu0000443
- Prete, G., Marzoli, D., Brancucci, A., Fabri, M., Foschi, N., & Tommasi, L. (2014). The processing of chimeric and dichotic emotional stimuli by connected and disconnected cerebral hemispheres. Behavioural Brain Research, 271, 354–364. https://doi.org/10.1016/j.bbr.2014.06.034
- Proud, M., Goodhew, S. C., & Edwards, M. (2020). A vigilance avoidance account of spatial selectivity in dual-stream emotion induced blindness. Psychonomic Bulletin & Review, 27(2), 322–329. https://doi.org/10.3758/s13423-019-01690-x
- Rouder, J. N., Speckman, P. L., Sun, D., Morey, R. D., & Iverson, G. (2009). Bayesian t tests for accepting and rejecting the null hypothesis. Psychonomic Bulletin & Review, 16(2), 225–237. https://doi.org/10.3758/PBR.16.2.225
- Schintu, S., Chaumillon, R., Guillaume, A., Salemme, R., Reilly, K. T., Pisella, L., & Farne, A. (2020). Eye dominance modulates visuospatial attention. Neuropsychologia, 141, 1–6. https://doi.org/10.1016/j.neuropsychologia.2019.107314
- Shobe, E. R. (2014). Independent and collaborative contributions of the cerebral hemispheres to emotional processing. Frontiers in Human Neuroscience, 8, 1–19. https://doi.org/10.3389/fnhum.2014.00230.
- Simon, J. R., & Wolf, J. D. (1963). Choice reaction time as a function of angular stimulus-response correspondence and age. Ergonomics, 6(1), 99–105. https://doi.org/10.1080/00140136308930679
- Singh, D., & Sunny, M. M. (2017). Emotion induced blindness is more sensitive to changes in arousal as compared to valence of the emotional distractor. Frontiers in Psychology, 8, 1–8.https://doi.org/10.3389/fpsyg.2017.01381.
- Smigasiewicz, K., Asanowicz, D., Westphal, N., & Verleger, R. (2014). Bias for the left visual field in rapid serial visual presentation: Effects of additional salient cues suggest a critical role of attention. Journal of Cognitive Neuroscience, 27(2), 1–14. https://doi.org/10.1162/jocn_a_00714.
- Śmigasiewicz, K., Shalgi, S., Hsieh, S., Möller, F., Jaffe, S., Chang, C.-C., & Verleger, R. (2010). Left visual-field advantage in the dual-stream RSVP task and Reading-direction: A study in three nations. Neuropsychologia, 48(10), 2852–2860. https://doi.org/10.1016/j.neuropsychologia.2010.05.027
- Smith, S. D., Most, S. B., Newsome, L. A., & Zald, D. H. (2006). An emotion-induced attentional blink elicited by aversively conditioned stimuli. Emotion, 6(3), 523–527. https://doi.org/10.1037/1528-3542.6.3.523
- Thom, N., Knight, J., Dishman, R., Sabatinelli, D., Johnson, D. C., & Clementz, B. (2014). Emotional scenes elicit more pronounced self-reported emotional experience and greater EPN and LPP modulation when compared to emotional faces. Cognitive, Affective, & Behavioral Neuroscience, 14(2), 849–860. https://doi.org/10.3758/s13415-013-0225-z
- Verleger, R., & Śmigasiewicz, K. (2015). Consciousness wanted, attention found: Reasons for the advantage of the left visual field in identifying T2 among rapidly presented series. Consciousness and Cognition, 35, 260–273. https://doi.org/10.1016/j.concog.2015.02.013
- Verleger, R., Sprenger, A., Gebauer, S., Fritzmannova, M., Friedrich, M., Kraft, S., & Jaśkowski, P. (2009). On why left events are the right ones: Neural mechanisms underlying the left-hemifield advantage in rapid serial visual presentation. Journal of Cognitive Neuroscience, 21(3), 474��488. https://doi.org/10.1162/jocn.2009.21038
- Wang, L., Kennedy, B. L., & Most, S. B. (2012). When emotion blinds: A spatiotemporal competition account of emotion-induced blindness. Frontiers in Psychology, 3, 1–6. https://doi.org/10.3389/fpsyg.2012.00438.
- Wang, L., & Most, S. B. (2016). The cost of seeing the meaning: Conceptual processing of distractors triggers localized target suppression. Visual Cognition, 24(9–10), 473–486. https://doi.org/10.1080/13506285.2017.1321076
- Weathers, F. W., Litz, B. T., Keane, T. M., Palmieri, P. A., Marx, B. P., & Schnurr, P. P. (2013). The PTSD Checklist for DSM-5 (PCL-5). www.ptsd.va.gov.
- Wetzels, R., Matzke, D., Lee, M. D., Rouder, J. N., Iverson, G. J., & Wagenmakers, E.-J. (2011). Statistical evidence in experimental psychology: An empirical comparison using 855 t tests. Perspectives on Psychological Science, 6(3), 291–298. https://doi.org/10.1177/1745691611406923
- Zago, L., Petit, L., Jobard, G., Hay, J., Mazoyer, B., Tzourio-Mazoyer, N., Karnath, H.-O., & Mellet, E. (2017). Pseudoneglect in line bisection judgement is associated with a modulation of right hemispheric spatial attention dominance in right-handers. Neuropsychologia, 94, 75–83. https://doi.org/10.1016/j.neuropsychologia.2016.11.024
- Zago, L., Petit, L., Mellet, E., Jobard, G., Crivello, F., Joliot, M., Mazoyer, B., & Tzourio-Mazoyer, N. (2016). The association between hemispheric specialization for language production and for spatial attention depends on left-hand preference strength. Neuropsychologia, 93, 394–406. https://doi.org/10.1016/j.neuropsychologia.2015.11.018
- Zhao, J. L., & Most, S. B. (2019). Manipulations of distractor frequency do not mitigate emotion-induced blindness. Cognition and Emotion, 33(3), 442–451. https://doi.org/10.1080/02699931.2018.1459490