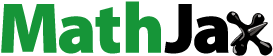
ABSTRACT
Palatable, unhealthy food stimuli can be devalued via Go/No-Go (GNG) training that consistently pairs such stimuli with motor inhibition. However, it remains unclear whether this devaluation is caused via learned associations with motor inhibition or via inferential learning based on the valence of emitted motor responses. The present research disentangles the effects of motor assignment and response valence in GNG training through task instructions. In two studies, chocolate stimuli were consistently paired with motor inhibition (“no-go”) or with motor excitation (“go”). Task instructions indicated that no-go responses were negatively valenced (“do not take”) and that go responses were positively valenced (“take”), or identified no-go responses as positively valenced (“keep”) and go as negatively valenced (“throw away”). The results show an effect of response valence on chocolate evaluations, but no effect of motor assignment: Chocolate stimuli were consistently devalued following pairings with a negatively valenced response, regardless of whether this response entailed motor inhibition or excitation. These findings align best with an inferential account of GNG training, suggesting that devaluation effects critically depend on inferential processes regarding motor response valence. GNG training procedures may, therefore, be optimised by disambiguating the valence of go and no-go motor responses prior to training.
Introduction
Overweight and obesity rates are alarmingly high and continue to rise rapidly (Finucane et al., Citation2011; World Health Organisation, Citation2017), placing individuals worldwide at risk of developing cardiovascular diseases, diabetes, musculoskeletal disorders and cancer (Lim et al., Citation2012). Though most people realise the risks associated with excess bodyweight, only a small proportion of individuals who attempt to lose weight are able to reduce their bodyweight over an extended period of time (Jeffery et al., Citation2000). In the interest of individual and public health, the current obesity epidemic calls for more effective interventions to reduce excessive bodyweight and related morbidity and mortality. Relatively recent efforts in this area have focused on motor response training, like Go/No-Go training (GNG), to change evaluations of unhealthy, high-calorie food and reduce overconsumption and overweight (Veling et al., Citation2017).
In GNG training procedures, food stimuli are presented alongside a go cue or a no-go cue (e.g. a frame presented around the stimulus in one of two colours). Participants are instructed to always execute a motor response toward stimuli that are presented with the go cue, and to withhold the motor response for stimuli that are presented with the no-go cue. During training, some food stimuli (e.g. high-caloric food products) are consistently presented with no-go cues, while other food stimuli (e.g. low-caloric food products) or non-food stimuli are consistently paired with go cues. Research has shown that consistently pairing food stimuli with no-go cues in GNG training leads to reduced intake of these foods relative to go associated foods (Chami et al., Citation2020; Houben & Jansen, Citation2011, Citation2015; Lawrence, Verbruggen, et al., Citation2015; Oomen et al., Citation2018; Veling et al., Citation2011; Veling et al., Citation2013; see also Aulbach et al., Citation2019; Jones et al., Citation2016; Wolz et al., Citation2020 for recent meta-analyses). Moreover, GNG training has been found to improve eating disorder symptomatology (Chami et al., Citation2020; Keeler et al., Citation2022) and facilitate weight loss (Lawrence, O'Sullivan, et al., Citation2015; Veling et al., Citation2014). Further, research has demonstrated that GNG training leads to more negative evaluations for no-go associated food stimuli as compared to goassociated stimuli (Adams et al., Citation2021; Chen et al., Citation2019; Chen, Veling, Dijksterhuis, et al., Citation2018; Houben & Giesen, Citation2018; Keeler et al., Citation2022; Lawrence, O'Sullivan, et al., Citation2015; Veling et al., Citation2013; Yang et al., Citation2019). As such, evidence suggests that GNG training may primarily affect eating behaviour by changing the underlying evaluations of no-go associated food stimuli (Johannes et al., Citation2021; Veling et al., Citation2013).
The reason for such a devaluation effect following GNG training is, however, still relatively unclear. While GNG training was originally believed to strengthen top-down inhibitory control, studies in the applied domain have failed to find consistent evidence for this idea (e.g. Aulbach et al., Citation2020; Carbine et al., Citation2021; Di Lemma & Field, Citation2017; Houben et al., Citation2012; Oomen et al., Citation2018; Poppelaars et al., Citation2018). Instead, GNG training is very easy to perform (e.g. Veling et al., Citation2011; Veling et al., Citation2014), without readily requiring acts of inhibitory control (Wessel, Citation2018). Consequently, it has been suggested that GNG training effects may instead rely on the formation of stimulus-action associations. According to these associative accounts, devaluation of no-go associated stimuli is caused by bidirectional associations between stimulus evaluations and motor action that is wired into motivational appetitive/aversive systems (Neumann et al., Citation2003; Verbruggen et al., Citation2014). On the one hand, positive, appetitive stimuli typically invigorate go or approach actions while negative, aversive stimuli trigger inhibition or avoidance (Chen & Bargh, Citation1999; Chiu et al., Citation2014). On the other hand, repeatedly pairing stimuli with go/approach or no-go/avoidance may cause these stimuli to acquire a positive or negative valence through associations with motivational appetitive/aversive systems (Neumann & Strack, Citation2000). Each time that a go/approach or no-go/avoidance response is executed towards a stimulus, the corresponding stimulus representation and motivational representation are both activated, thereby gradually strengthening the association between those representations (Neumann et al., Citation2003; Verbruggen et al., Citation2014). In line with this idea, research has shown that inaction (no-go) is inherently perceived as more negative compared to action (go) (McCulloch et al., Citation2012; Sunderrajan & Albarracín, Citation2021), suggesting that negative valence associated with no-go responses may indeed feed back on the evaluation of stimuli that are associated with inaction.
Such associative accounts, however, cannot readily explain why GNG training effects are typically stronger for highly valued stimuli as compared to low value stimuli (Chen et al., Citation2016; Veling et al., Citation2021), or why training appears more effective among individuals who are more hungry (Chen, Veling, de Vries, et al., Citation2018), have higher dietary restraint standards (Houben & Jansen, Citation2011; Lawrence, Verbruggen, et al., Citation2015; Veling et al., Citation2013), or have higher initial preferences for the trained foods (Forman et al., Citation2019; Veling et al., Citation2013). The Behaviour Stimulus Interaction theory (BSI; Veling et al., Citation2008) instead explains the devaluation that is observed following GNG training as a consequence of experienced response conflict. Specifically, appetitive stimuli elicit strong approach action tendencies that need to be inhibited when presented alongside a no-go cue. To resolve the ensuing response conflict and prevent continuous oscillation between approach and inhibition, the appetitive stimulus is devalued (Chen et al., Citation2016; Veling et al., Citation2008). As such, a stronger devaluation effect is expected when the experienced response conflict is stronger, as is the case for highly valued stimuli and among individuals who exhibit increased approach tendencies toward food stimuli due to hunger, food preferences or dietary restraint. However, BSI fails to clarify why response conflict would specifically result in devaluation rather than other forms of regulation strategies to resolve the experienced conflict.
While associative accounts and BSI place strong emphasis on the nature of the executed motor response in GNG training, the inferential account of motor response training instead stresses the importance of inferences that individuals make about the relation between stimulus and action as well as the affective value of those actions (Van Dessel et al., Citation2019). To be more precise, this account proposes that individuals infer the value of a stimulus through actions performed towards that stimulus. When a stimulus is paired with (in)action, inferences are drawn about the stimulus-action relation (e.g. “I did not respond to this object”). Based on subsequent inferences that are drawn about the relation between the performed action and valence (e.g. “not responding is negative”), individuals may construe an inference about the evaluative properties of the stimulus (e.g. “I did not respond to this object, so I probably do not like this object”). Importantly, this account predicts that any information that influences the detection of stimulus-action relations or action-evaluation links will influence the effect on stimulus evaluations (Van Dessel et al., Citation2019; Van Dessel, Eder, et al., Citation2018).
The inferential account, thus, predicts that GNG devaluation effects will strongly depend on the inferences that participants make regarding the valence of the performed actions. Such inferences may reflect the intrinsic affective value of actions, but may also be based on any other type of information that is available, including, for instance, task instructions. Associative accounts and the BSI, in contrast, focus mostly on the intrinsic value of (in)action and would not readily predict effects of task instructions regarding action valence: No-go associated stimuli would be inferred to be negative to the degree that non-action is perceived as negative. Even though inaction may generally be perceived as more negative compared to action (McCulloch et al., Citation2012; Sunderrajan & Albarracín, Citation2021), this is likely not the same for everyone and will depend on individual learning histories. As there might exist individual differences in the way participants infer action valence, evaluative stimulus-action effects may differ between individuals if action valence is left unspecified (Van Dessel et al., Citation2019). Uncovering the exact mechanism of how GNG influences evaluations is therefore important as it may carry important implications for improving GNG training protocols to elicit stronger effects.
The aim of this research was to investigate whether GNG devaluation effects are determined by repeated pairings with motor inhibition and/or via response valence by manipulating how go and no-go responses are evaluatively framed. The present research comprises two studies that used a GNG protocol as used in previous research (e.g. Houben & Jansen, Citation2011, Citation2015): In two different training conditions, chocolate stimuli were either consistently paired with a no-go response (chocolate/no-go) or paired with a go response (chocolate/go). In addition, participants were assigned to one of two instruction conditions with different framing of go and no-go responses: In one condition, “go” was framed as “taking” (positive) while “no-go” was framed as “not taking” (negative), which is consistent with how responses are perceived to be (implicitly) framed in GNG training. In the other condition, “go” was instead framed as “throwing away” (negative) whereas no-go had a positive connotation, “keep”. As such, these studies offer simultaneous, but separate tests of associative accounts/BSI and the inferential account. Associative accounts and BSI, which emphasise the importance of pairing stimuli with motor inhibition, would predict stronger negative evaluations of chocolate in the conditions that pair chocolate with motor inhibition (no-go) relative to the conditions that pair chocolate with motor response (go), independently of task instructions that specify the valence of those responses. The inferential account, in contrast, would predict more negative evaluations for chocolate when paired with responses that are inferred to be negative as compared to conditions that paired chocolate with a positive response, independently of whether such responses entail motor inhibition or motor excitation. In theory, associative and inferential processes may also both be involved in GNG devaluation effects, which would be evidenced by main effects of both motor inhibition and response valence. Sample size in both studies was based on a power analysis that would allow 80% power to detect medium effects of = .06 at α = .05.
Study 1
Methods
Participants
Participants were undergraduate students from Maastricht University who participated in the study for course credit. In total, 113 students signed up to participate in the study, of which 104 completed the study. Three participants were excluded due to their performance on the GNG task: One participant was excluded because this participant only completed three trials of the GNG task due to a technical error, and two participants were excluded due to low accuracy (i.e. 2.5 SD below the sample mean). The final sample therefore consisted of 101 participants, of which 84 identified as female, 16 identified as male, and 1 identified as other/neither female or male. Participants’ age ranged from 18 to 30 years (M = 21.49, SD = 2.62).
Materials and measures
Go/No-Go (GNG) task
During the GNG task, participants were shown pictures on the computer screen and were instructed to press the space bar when the picture was presented within a blue frame (i.e. go cue) and to refrain from responding when the picture was presented within a grey frame (i.e. no-go cue). The stimuli were four pictures of chocolate (e.g. small pieces of chocolate, chocolate bar), four pictures of fruit (e.g. apple, orange), and eight food-related filler pictures consisting of four high-calorie food products (e.g. crisps, coated peanuts) and four low-calorie food products (e.g. tomatoes, carrots). During each trial, a picture was presented first, and a blue or grey frame appeared around the picture 100 ms after picture onset. The frame remained visible together with the picture for the remainder of the trial. Trial duration was 1000 ms. A green circle was displayed after a correct (non)response (500 ms), and a red cross after an incorrect (non)response (500 ms). The inter-trial interval was 500 ms.
The GNG task consisted of two blocks of 160 trials. During each block, all stimuli were presented ten times. Pictures from the chocolate category and the fruit category were consistently paired with the go or no-go cue in one of two motor assignment conditions: For half of the participants, chocolate-related pictures were consistently paired with the no-go cue, while pictures of fruit were consistently paired with the go cue. For the other half of the participants, the pairing was reversed so that chocolate-related pictures were always paired with the go cue, and pictures of fruit were always paired with the no-go cue. The eight filler pictures were presented with the go cue on half of the trials and with the no-go cue on the other half of the trails. Go and no-go trials were presented in random order. Participants were further randomly assigned to one of two instruction conditions to manipulate the valence of the motor responses: In one condition, participants were instructed that the blue frame (i.e. go cue) indicated that they should “take” the item by pressing the response key (i.e. go/positive) while the grey frame indicated that they should “not take” the item by not responding (i.e. no-go/negative). In the second condition, participants were instructed that the blue frame indicated that they should “throw away” the item by pressing the response key (i.e. go/negative) while the grey frame indicated that they should “keep” the item by not responding (i.e. no-go/positive).
Picture rating task
During the picture rating task, all pictures that were presented in the GNG task were presented on the computer screen one by one. In addition to the trained picturesFootnote1, the rating task also included 10 novel chocolate pictures that were not used during GNG training (e.g. chocolate in different shapes and products of processed chocolate like chocolate ice-cream and chocolate donuts) to test whether training effects would generalise to category exemplars that were not included in GNG training. All pictures were presented in random order. Participants provided an evaluation for each picture (0 = “not appealing at all”; 100 = “very appealing”) and rated their current desire for the pictured food (0 = “no desire at all”; 100 = “very strong desire”) on two 100-point visual analogue scales. Ratings were averaged into an overall evaluation score, separately for each food category (i.e. chocolate – trained, fruit – trained, and chocolate – untrained).
Craving
Participants indicated on two 100-point visual analogue scales how much hunger they experienced at that moment (0 = “not hungry”; 100 = “very hungry”), and how strongly they desired to eat something at that moment (0 = “no desire”; 100 = “strong desire”). Responses were averaged across the two questions into one overall craving score.
Procedure
The study was approved by the ethical review board of the Faculty of Psychology and Neuroscience, Maastricht. The study was performed entirely online. After giving consent, participants filled out their demographic information (age and gender identification). Next, participants rated their current hunger and craving levels on two visual analogue scales. Participants were then randomly assigned to one of four conditions (chocolate – go/positive: n = 22; chocolate – go/negative: n = 24; chocolate – no-go/positive: n = 27; chocolate – no-go/negative: n = 28), and performed the GNG task. After the GNG task, participants performed the picture rating task. Participants were thanked and fully debriefed at the end of the study and received a gift voucher or course credit as remuneration for their participation in the study.
Results
Baseline and GNG task performance
Baseline craving and accuracy during the GNG task were both analysed using 2 × 2 ANOVA with response valence (positive versus negative) and motor assignment (go versus no-go) as between-subjects factors. On average, participants did not show strong food cravings at baseline (M = 33.93, SD = 24.78) with no significant differences between the valence and motor assignment conditions (all F ≤ 1.46, all p ≥ .23). Participants showed high accuracy during the GNG task (M = .99, SD = .02) with no significant differences between the conditions (all F ≤ .41, all p ≥ .52).
Picture evaluations
The effect of response valence and motor assignment on evaluations of trained food stimuli was examined via mixed 2 × 2 x 2 ANOVA with type of stimulus (chocolate versus fruit) as within-subjects factor, and with response valence and motor assignment as between-subjects factors. The results showed a significant main effect of stimulus type, F(1, 97) = 40.03, p < .001, = .29, in addition to a significant interaction between stimulus type and valence, F(1, 97) = 4.15, p = .04,
= .04. None of the other main or interaction effects were significant (all F ≤ 1.64, all p ≥ .20). Follow-up analyses showed no significant effect of response valence on fruit evaluations, F(1, 99) = .28, p = .60. For chocolate stimuli, the results showed lower evaluations when chocolate was paired with a negative response than when chocolate was paired with a positive response (see ), though this difference was not statistically significant, F(1, 99) = 3.48, p = .07,
= .03. In addition to trained chocolate stimuli, participants also rated novel, untrained chocolate pictures. Evaluations for these untrained stimuli were analysed in a similar way using 2 × 2 ANOVA with response valence and motor assignment as between-subjects factors. The pattern of findings was highly similar to the findings for trained chocolate stimuli: Results showed no significant effects involving motor assignment (motor: F(1, 97) = 1.78, p = .19; valence*motor: F(1, 97) = .18, p = .67). Only the main effect of response valence was statistically significant, F(1, 97) = 5.43, p = .02,
= .05, indicating that chocolate evaluations were lower when chocolate was paired with a negative response than when chocolate was paired with a positive response, irrespective of motor assignment (see ).
Figure 1. Mean food evaluations with standard errors for trained chocolate stimuli (choco-T), trained fruit stimuli (fruit-T) and novel untrained chocolate stimuli (choco-UNT). Evaluations are displayed per response valence condition (positive versus negative valence) and motor assignment condition (no-go versus go).
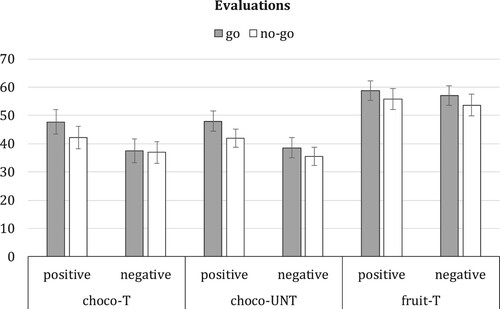
Discussion
In study 1, the independent contribution of response valence and motor assignment to the GNG devaluation effect was examined by changing the evaluative framing of go and no-go motor responses through task instructions. Results showed no effect of motor assignment on chocolate ratings, such that pairing chocolate with no-go did not lead to significantly lower ratings as compared to pairing chocolate with go. Instead, chocolate ratings were lower when chocolate had been paired with a negatively valenced response than when paired with a positively valenced response, irrespective of whether that response entailed motor inhibition or motor excitation. While the pattern was the same for trained and untrained chocolate pictures, the effect of response valence was only significant in the evaluations of novel, untrained chocolate pictures. For fruit stimuli, there was no evidence for an effect of motor assignment or response valence on stimulus evaluations.
Thus, the findings for chocolate stimuli seem more in line with an inferential account, with evaluations reflecting the valence of the responses associated with the stimuli during training. However, effects were generally small, which could be due to individual differences in food evaluations prior to training. As study 1 did not include a pretest, it is unclear whether pre-existing differences in food evaluations between conditions may have influenced the results, nor was it possible to test whether evaluations indeed changed over time. Moreover, the present findings could reflect a devaluation in stimuli associated with a negative response, as well as a valuation effect for stimuli associated with a positive response (similar to the previously described go-valuation effect; Chen et al., Citation2016; Chen, Veling, de Vries, et al., Citation2018; Chen, Veling, Dijksterhuis, et al., Citation2018). In a second, pre-registered study (https://aspredicted.org/9ep5k.pdf), the effect of response valence and motor inhibition on food evaluations was examined using a similar design and procedure with the addition of a pretest. Compared to the procedure of study 1, study 2 included the following changes: (1) To better differentiate between devaluation and valuation effects, study 2 included baseline measures of food evaluations that allowed assessment of change over time; (2) In addition to trained stimuli, ratings were also collected for novel, untrained chocolate and fruit stimuli; (3) Filler stimuli were no longer included in the training; and (4) Dietary restraint was measured as a potential moderator of GNG training effects.
Study 2
Methods
Participants
Participants were undergraduate students from Maastricht University who participated in the study for course credit. A total of 115 students signed up to participate in the study, of which 107 completed the study. Six participants were excluded due to their performance on the GNG task: Three participants never responded during the GNG while three additional participants were excluded due to low accuracy on the GNG task (i.e. 2.5 SD below the sample mean). The final sample consisted of 101 participants, who all identified as female. Participants’ age ranged from 18 to 31 years (M = 23.12, SD = 2.74).
Materials and measures
Go/No-Go (GNG) task
The GNG task was the same as used in study 1 but without the inclusion of filler pictures. The GNG task consisted of 2 blocks of 80 trials during which four chocolate pictures and four fruit pictures were presented 10 times. As before, pictures from the chocolate category and the fruit category were consistently paired with the go or no-go cue and this pairing was counterbalanced across participants. Participants were randomly assigned to one of two instruction conditions: Participants were either instructed that the blue frame (i.e. go cue) indicated a positive response (i.e. “take”) while the grey frame (i.e. no-go cue) indicated a negative response (i.e. “do not take”), or they were instructed that the blue frame signalled a negative response (i.e. go; “throw away”) whereas the grey frame signalled a positive response (i.e. no-go: “keep”).
Picture rating task
Similar to study 1, participants rated all pictures that were presented during the GNG task on two 100-point visual analogue scales (Evaluation: 0 = “not appealing at all”; 100 = “very appealing”; Desire: 0 = “no desire at all”; 100 = “very strong desire”). In addition, participants also rated four novel, untrained chocolate pictures (e.g. chocolate in different shapes and products of processed chocolate like chocolate ice-cream and chocolate donuts) and four novel, untrained fruit pictures (e.g. different types of fruit, including pears and strawberries). All pictures were presented in random order. Ratings were averaged into one evaluation score, separately for each food category (i.e. chocolate versus fruit).
Craving
As in study 1, participants rated their current hunger level (0 = “not hungry”; 100 = “very hungry”), and their current desire to eat something (0 = “no desire”; 100 = “strong desire”) on two 100-point visual analogue scales. Responses were averaged across the two questions into one craving score.
Dietary restraint
Dietary restraint was measured using the revised Restraint Scale (RS; Herman & P., Citation1980). The RS is a self-report questionnaire consisting of 10 items that measure dieting concern/intentions and weight fluctuations. Higher scores indicate an increased intention to restrict food intake.
Procedure
The study was approved by the ethical review board of the Faculty of Psychology and Neuroscience, Maastricht and performed entirely online. After providing consent, participants filled out their demographic information (age and gender identification). Next, participants rated their current hunger and craving levels and they performed the picture rating task for the first time. Participants were then randomly assigned to one of four conditions (chocolate – go/positive: n = 25; chocolate – no-go/negative: n = 27; chocolate – go/negative: n = 29; chocolate – no-go/positive: n = 20), and performed the GNG task. Afterwards, participants performed the picture rating task for a second time. At the end of the study, participants were thanked and fully debriefed. Participants received a gift voucher or course credit as remuneration for participating in the study.
Results
Baseline and GNG task performance
Baseline differences between conditions in craving and dietary restraint as well as accuracy during the GNG task were analysed using 2 × 2 ANOVA with response valence (positive versus negative) and motor assignment (go versus no-go) as between-subjects factors. There were no significant differences in food cravings at baseline (M = 39.53, SD = 26.85) between the valence and motor assignment conditions (all F ≤ .77, all p ≥ .38). Dietary restraint was significantly lower in the conditions that paired chocolate with a no-go motor response (M = 13.62, SD = 4.71) than in conditions that paired chocolate with go (M = 16.66, SD = 5.64), F(1, 97) = 8.62, p < .01, = .08.Footnote2 There was no significant effect of response valence, F(1, 97) = 2.36, p = .13, or motor*valence on dietary restraint, F(1, 97) = .05, p = .83. Participants showed high accuracy during the GNG task (M = .97, SD = .05) with no significant differences between the conditions (all F ≤ 1.77, all p ≥ .19).
Picture evaluations
The effect of response valence and motor assignment on food evaluations was examined via 2 × 2 x 2 × 2 ANOVA with time (pretest versus posttest) and type of stimulus (chocolate versus fruit) as within-subjects factors, and with response valence and motor assignment as between-subjects factors. Results showed a significant time*type*valence interaction, F(1, 97) = 4.63, p = .03, = .05. None of the other main or interaction effects were statistically significant (all F ≤ 2.32, all p ≥ .13). Follow-up analyses showed no significant change over time in fruit evaluations following pairings with positive valence, F(1, 55) = .42, p = .52, or following pairings with negative valence, F(1, 44) = 1.80, p = .19 (see ). Chocolate evaluations also did not change significantly over time when chocolate was paired with positive valence, F(1, 44) = .12, p = .73. However, when chocolate was paired with a negatively valenced response, chocolate evaluations significantly decreased from pretest to posttest, F(1, 55) = 7.97, p = .01,
= .13 (see ). The same analysis was also performed separately for trained and untrained stimuli to investigate whether training effects generalised from the trained stimuli to novel, untrained stimuli that belonged to the same food category. Results were highly similar for trained and untrained stimuli, though the time*type*valence interaction did not reach statistical significance for trained, F(1, 97) = 3.69, p = .06, or for untrained stimuli, F(1, 97) = 3.68, p = .06.
Figure 2. Mean difference scores (posttest - pretest) for chocolate and fruit evaluations with standard errors. Difference scores are shown separately per response valence condition (positive versus negative valence) and motor assignment condition (no-go versus go). Negative scores indicate reduced food evaluations at posttest compared to pretest; positive scores indicate increased food evaluations at posttest compared to pretest.
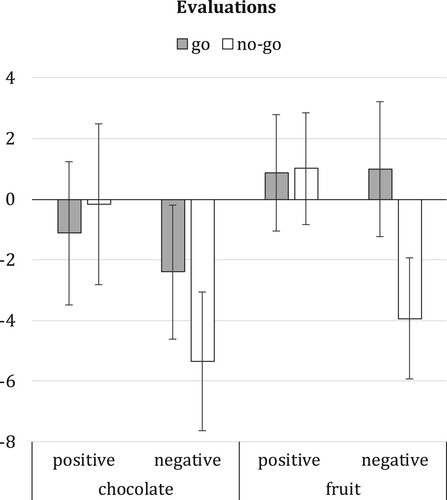
Dietary restraint
Exploratory analyses were performed to examine whether dietary restraint acted as a moderator in the effects of training on food evaluations. For simplicity, evaluation difference scores (posttest – pretest) were analysed using ANOVA with type of stimulus as within-subjects factor, response valence and motor assignment as between-subjects factors, and standardised dietary restraint scores as a continuous covariate2. Results showed a significant main effect of restraint, F(1, 93) = 4.84, p = .03, = .05, and a significant interaction between type and valence, F(1, 93) = 4.53, p = .04,
= .05, qualified by a significant type*valence*restraint interaction, F(1, 93) = 7.93, p = .01,
= .08. None of the other main or interaction effects reached statistical significance (all F ≤ 2.40, all p ≥ .13). Follow-up analyses showed no significant effects involving valence and restraint for fruit stimuli (all F ≤ 1.99, all p ≥ .16). For chocolate stimuli, the main effect of restraint was significant, F(1, 97) = 5.51, p = .02,
= .05, but not the main effect of valence, F(1, 97) = 2.96, p = .09. Importantly, the restraint*valence interaction was significant, F(1, 97) = 4.34, p = .04,
= .04, indicating a significant effect of valence at high levels of dietary restraint (+1 SD), F(1, 97) = 6.89, p = .01,
= .07, but not at low levels of dietary restraint (−1 SD), F(1, 97) = .10, p = .76. Among high restrained participants, pairing chocolate with negative valence resulted in reduced chocolate evaluations (M = −3.55, SE = 1.99), while pairing chocolate with positive valence resulted in increased chocolate evaluations (M = 5.44, SE = 2.79). For low restrained participants, chocolate evaluations were lower at posttest than at pretest, following pairings with negative valence (M = −4.18, SE = 2.24) as well as pairings with positive valence (M = −5.19, SE = 2.35).
Discussion
Similar to the results of study 1, the findings from study 2 show an effect of response valence, but not motor assignment, on evaluations of chocolate pictures. Chocolate pictures were devalued after being paired with a negatively valenced response and did not change after being paired with a positively valenced response. This devaluation effect was found irrespective of whether the negatively valenced response entailed motor excitation (go) or motor inhibition (no-go). As in study 1, this devaluation effect was similar for both trained and novel, untrained chocolate stimuli. Furthermore, the devaluation effect was only observed for chocolate stimuli and not for fruit stimuli.
General discussion
A fairly robust finding in GNG research is that pairing stimuli with a no-go motor response leads to a devaluation of those stimuli, suggesting that GNG training may primarily affect eating behaviour by changing underlying food evaluations (Veling et al., Citation2017). The aim of the present research was to examine whether no-go associated stimuli are devalued due to repeated pairings with motor inhibition or because of the valence of the motor responses that are emitted toward those stimuli. In two studies, the evaluative framing of go and no-go motor responses was manipulated via task instructions to separate the effects of motor assignment and response valence on stimulus evaluations. The findings show an effect of response valence, but not of motor assignment, on evaluations: In study 1, evaluations of chocolate stimuli were more negative following repeated pairings with a negative response than when paired with a positive response, regardless of whether this negative response was operationalised as motor inhibition (no-go) or motor excitation (go). In study 2, this effect was replicated but now including a baseline measure of evaluations. Chocolate stimuli were devalued following repeated pairings with a negatively valenced response. When chocolate was paired with a positively framed response, no change in evaluations was observed.
Theoretical implications
Several accounts have been proposed to explain why GNG training leads to stimulus devaluation. Associative accounts and BSI focus mostly on the intrinsic value of the motor responses that are repeatedly paired with stimuli during training. According to associative accounts, stimuli that are repeatedly paired with motor inhibition (no-go) are devalued via associative links between motor inhibition responses and a negative, aversive motivational system that feed back on stimulus evaluations (Neumann et al., Citation2003; Verbruggen et al., Citation2014). BSI proposes that appetitive or rewarding stimuli automatically trigger an approach tendency (via an appetitive motivational system), which needs to be inhibited when the stimuli are paired with a no-go motor inhibition response. The resulting conflict between the approach tendency and the task requirement of motor inhibition is then assumed to be resolved by devaluing the stimuli (Veling et al., Citation2008; Veling et al., Citation2017). Across two studies, the pattern of findings, however, suggests that devaluation effects critically depend on the evaluative framing of motor responses: A devaluation effect was observed for stimuli that were paired with a negatively valenced response, regardless of the type of action (go or no-go) that was performed.
The present findings are therefore more consistent with the inferential account of motor training, which proposes that participants draw inferences about the valence of a stimulus through actions performed towards that stimulus (Van Dessel et al., Citation2019). Repeated no-go actions in response to a stimulus lead to inferential learning about the evaluative properties of that stimulus based on the inferred relation between action and stimulus and the valence of the action. Importantly, inferences about the valence of responses can be based on any information that is available (e.g. task instructions) and is not confined to intrinsic motor response valence. Unlike associative accounts and BSI, the inferential account thus assigns a critical role to task instructions that influence the inferences formed about the valence of emitted motor responses. When stimuli are repeatedly paired with a motor response that is inferred to be negative (e.g. because of task instructions), participants are more likely to infer that their evaluation of those stimuli is also negative. The present findings are also consistent with the common-coding account of motor response training (Eder & Klauer, Citation2009) that bears similarity to the inferential account. The common-coding account proposes that repeated action in response to a stimulus leads to the formation of links between the stimulus representation and the affective code that is part of the response representation, biasing stimulus evaluations toward the valence of the response (Eder & Klauer, Citation2007, Citation2009). Such affective codes can be based on the intrinsic valence of responses, but may also be influenced by task instructions.
Together, the present findings suggest that GNG training is effective at changing stimulus evaluations because it facilitates the formation of inferences regarding stimulus valence based on the valence of performed actions. Importantly, BSI and associative accounts do not necessarily exclude the potential influence of task instructions, as associative processes and inferential learning may jointly influence GNG effects. However, the current findings do argue against a strong associative account of GNG devaluation effects in terms of motor inhibition, by showing that inferential processes influence stimulus evaluations in GNG. However, it should be noted that the GNG training with verbal instructions as used in the present research differs from the original implementation that does not include such verbal instructions. While it is possible that adding verbal labels to the motor responses may have invited processes that are otherwise not activated during GNG training, the present findings do argue against a strict explanation of GNG training in terms of motor action alone. These findings may be reconciled with BSI by allowing more room for propositional information in the creation and resolution of response conflict. Pairing appetitive stimuli with any type of response that is inferred to be negative based on propositional information may potentially lead to response conflict. The resolution of this response conflict could then similarly involve an inference-based process.
The current findings also suggest that GNG training may be more similar to other motor response training procedures than previously thought: Any motor response that is paired with a stimulus may result in stimulus devaluation to the extent that it is inferred to be negative. As such, it is interesting to note that Approach-Avoidance training (AAT), a motor response training procedure that involves pairing stimuli with avoidance or approach responses, has similarly been proposed to crucially depend on inferential learning (Van Dessel, Eder, et al., Citation2018). Moreover, a recent study found GNG and AAT training to be equally effective in changing stimulus evaluations (Veling et al., Citation2021). While it is possible that the verbal instructions used in the current research may have caused the GNG training to be even more similar to AAT training, the available evidence tentatively suggests that there might be no qualitative difference between training to avoid certain food stimuli and training to not respond to food stimuli. Future research that aims to determine which theoretical accounts best explain GNG training effects should therefore also focus on the commonalities and possible differences in the mechanisms involved in these procedurally similar motor response training tasks.
Practical implications
Importantly, inferences about response valence (and consequently stimulus evaluations) can be based on any information that is readily available during training. In absence of specific task instructions, as is typically the case in GNG training studies, participants may be inclined to infer that a no-go response is more negative as compared to a go response (McCulloch et al., Citation2012; Sunderrajan & Albarracín, Citation2021). As such, these findings are in line with previous research showing devaluation of no-go associated food stimuli relative to go-associated stimuli (e.g. Adams et al., Citation2021; Chen et al., Citation2019; Chen, Veling, Dijksterhuis, et al., Citation2018; Houben & Giesen, Citation2018; Keeler et al., Citation2022; Lawrence, O'Sullivan, et al., Citation2015; Veling et al., Citation2013; Yang et al., Citation2019). In the absence of specific task instructions, inferences regarding response valence, however, will strongly depend on one’s individual learning experiences that relate specific actions to valence (Van Dessel et al., Citation2019). While some individuals may easily infer that inaction is negative, others may be more inclined to retrieve information that action/approach can also have a negative valence. Such individual differences may influence GNG training effects, especially when task instructions do not disambiguate the valence of motor responses. Such individual differences may at least partly explain why some studies obtained no effects on stimulus evaluations following GNG training (e.g. Adams et al., Citation2017; Bowley et al., Citation2013; Liu et al., Citation2022; Tzavella et al., Citation2021).
To maximise the impact of GNG training, task instructions should, thus, specify the meaning or valence of go versus no-go responses. In addition, GNG training procedures could also be improved by including consequences of stimulus-action relations (e.g. negative consequences when approaching and positive consequences when avoiding unhealthy food) to further facilitate the generation of relevant inferences regarding response and stimulus valence (Van Dessel et al., Citation2019; Van Dessel, Hughes, et al., Citation2018). If GNG training indeed critically depends on inferential processing, one could additionally question whether similar training effects may be achieved by merely instructing participants about stimulus-action contingencies through verbal instruction, without actually having them perform these actions. To date, no research has investigated this possibility in relation to GNG training. Research on AAT, however, has shown effects of mere instruction about the stimulus-action relationship (Van Dessel et al., Citation2015; Van Dessel et al., Citation2016) as well as effects of simple observation of the stimulus-action relationship (Van Dessel, Eder, et al., Citation2018) on evaluations for novel, unvalenced stimuli. However, for valenced, well-known stimuli, mere instructions about stimulus-action contingencies were not potent enough to induce evaluation change (Van Dessel et al., Citation2015). Whether devaluation effects can be demonstrated when participants are merely instructed about go and no-go responses requires further investigation, though at least one study has shown that mere observation of GNG training does not lead to reliable changes in evaluations for palatable food stimuli (Chen et al., Citation2016). Overall, these findings suggest that, at least for well-known, valenced stimuli, mere instruction and observation about stimulus-action contingencies may not be potent enough to induce changes in evaluations and that actually performing actions in relation to such stimuli may be required to lend more power to the newly learned propositions.
Limitations and questions for future research
In both studies, similar devaluation effects were observed for trained and untrained, novel chocolate stimuli, suggesting that repeated pairings of stimuli with negative response valence may generalise to other untrained exemplars that belong to the same food category. Some previous studies have similarly reported generalisation of GNG training effects towards other, novel exemplars that belonged to the same category as trained stimuli (e.g. Adams et al., Citation2017; Folkvord et al., Citation2016), though generalisation is not always found (e.g. Adams et al., Citation2021). As generalisation generally takes place between stimuli that resemble each other either perceptually and/or conceptually (Osherson et al., Citation1990; Sloman, Citation1993), generalisation is likely stronger for novel stimuli that share more perceptual and/or conceptual features with the trained stimuli. Future research could include measures of perceived perceptual and conceptual similarity between trained and untrained stimuli to further examine when and how training effects generalise beyond the training context. In addition, this research only focused on food evaluations but not on actual food intake. It is therefore unclear if and how observed changes in evaluations would translate into behaviour change. Though there is some evidence to suggest that changes in evaluations may underlie GNG training effects on behaviour (Johannes et al., Citation2021), more research is needed to clarify the exact mechanisms through which GNG training impacts food intake.
Importantly, devaluation effects were only found for chocolate stimuli while no clear effects of training were observed for fruit stimuli. The lack of training effects with respect to fruit stimuli could relate to the previously described findings that GNG training effects are generally stronger for high value stimuli as compared to stimuli of low value (Chen et al., Citation2016; Veling et al., Citation2008; Veling et al., Citation2021; but see Chen, Veling, Dijksterhuis, et al., Citation2018). However, based on the evaluation scores at pretest in study 2, there was no evidence to suggest that chocolate stimuli were liked more as compared to fruit stimuli in the present research (chocolate: M = 53.21, SD = 19.86; fruit: M = 53.32, SD = 17.39). Importantly, at least one other study has similarly found reliable devaluation effects only when unhealthy stimuli were paired with no-go responses and healthy, fruit stimuli with go responses, but not in the reversed motor assignment condition (i.e. unhealthy/go versus healthy/no-go; Serfas et al., Citation2017). It was argued that consistently pairing unhealthy snacks with no-go responses and fruit with go responses may be more meaningful because the no-go associated stimuli belong to a category for which devaluation is normative and desired as compared to healthy food for which devaluation may be less meaningful and inconsistent with health goals (Serfas et al., Citation2017).
Consistent with this idea, dietary restraint was found to significantly moderate the effect of training on chocolate evaluations. More specifically, the effect of evaluative framing on chocolate evaluations was only significant at high levels of dietary restraint, but not at low levels of dietary restraint. Previous research has similarly found stronger GNG training effect among individuals with high dietary restraint (Houben & Jansen, Citation2011; Lawrence, Verbruggen, et al., Citation2015; Veling et al., Citation2011). Together these findings thus seem to fit the argumentation that GNG training effects may be stronger when in line with normative goals (Serfas et al., Citation2017). Hence, one would anticipate a more pronounced devaluation of chocolate (but not of fruit) at higher levels of dietary restraint. However, it should be noted that the present findings suggested a similar devaluation following negative response pairings with chocolate both at low and high levels of restraint. The difference instead seems to be caused by differential responding to positive response pairings with chocolate, which resulted in increased evaluations among individuals with high dietary restraint and devaluation at low levels of restraint. As higher levels of dietary restraint were overall associated with lower chocolate evaluations at baseline (r = -.21, p = .04), pairings of chocolate with positive valence may have resulted in a stronger discrepancy among individuals high in dietary restraint, fostering stronger effects of the evaluative framing, as compared to individuals low in dietary restraint.
The observation that GNG training effects appear stronger in restrained eaters (Houben & Jansen, Citation2011; Lawrence, Verbruggen, et al., Citation2015; Veling et al., Citation2011), and when initial stimulus value is higher (Chen et al., Citation2016; Veling et al., Citation2008; Veling et al., Citation2021) could also fit with an inferential account of GNG training: A stronger discrepancy between (high) valued stimuli and an incompatible, valenced action may result in stronger inferences about the evaluation of the stimuli (especially when there is no other valid reason available for the action; Van Dessel et al., Citation2019). Moreover, inferences about stimulus evaluations do not only depend on the inferred stimulus-action and action-valence relationships, but also critically depend on current goals and willingness to integrate this information into inferences about stimulus valence (Van Dessel et al., Citation2019). It would therefore be interesting for future research to examine how individual differences in motivation, goals and initial stimulus value affect the inferential processes involved in GNG training.
Conclusions
The goal of the present research was to disentangle the effects of motor assignment and response valence in producing the stimulus devaluation effect that is commonly observed following GNG training. In two studies, chocolate stimuli were devalued following repeated pairings with negatively valenced motor responses, irrespective of whether these responses entailed motor inhibition or motor excitation. Hence, the current findings align best with an inferential account of GNG training effects that is based on propositional learning. Stimuli are devalued when they are repeatedly paired with motor responses that are inferred or perceived to be negative. Therefore, to maximise the effects of GNG training, task instructions should disambiguate the valence of go and no-go responses.
Disclosure statement
No potential conflict of interest was reported by the author(s).
Data availability
All data are available at https://doi.org/10.34894/UYSS04.
Notes
1 Filler stimuli were also presented during the picture rating task. However, these ratings were not further analysed as fillers were not consistently paired with positive or negative response valence or with motor excitation versus motor inhibition (i.e., go versus no-go).
2 Fourteen participants did not fill out the Restraint Scale. Missing data for the total score on the Restraint Scale were imputed with the sample mean. When the analyses were performed with list wise deletion (n = 87), the pattern of results remained the same.
References
- Adams, R. C., Button, K. S., Hickey, L., Morrison, S., Smith, A., Bolus, W., Coombs, E., Randolph, S., Hunt, R., & Kim, D. (2021). Food-related inhibitory control training reduces food liking but not snacking frequency or weight in a large healthy adult sample. Appetite, 167, 105601. https://doi.org/10.1016/j.appet.2021.105601
- Adams, R. C., Lawrence, N. S., Verbruggen, F., & Chambers, C. D. (2017). Training response inhibition to reduce food consumption: Mechanisms, stimulus specificity and appropriate training protocols. Appetite, 109, 11–23. https://doi.org/10.1016/j.appet.2016.11.014
- Aulbach, M. B., Harjunen, V. J., Spape, M., Knittle, K., Haukkala, A., & Ravaja, N. (2020). No evidence of calorie-related modulation of N2 in food-related Go/No-Go training: A preregistered ERP study. Psychophysiology, 57(4), e13518. https://doi.org/10.1111/psyp.13518
- Aulbach, M. B., Knittle, K., & Haukkala, A. (2019). Implicit process interventions in eating behaviour: A meta-analysis examining mediators and moderators. Health Psychology Review, 13(2), 179–208. https://doi.org/10.1080/17437199.2019.1571933
- Bowley, C., Faricy, C., Hegarty, B., Johnstone, S. J., Smith, J. L., Kelly, P. J., & Rushby, J. A. (2013). The effects of inhibitory control training on alcohol consumption, implicit alcohol-related cognitions and brain electrical activity. International Journal of Psychophysiology, 89(3), 342–348. https://doi.org/10.1016/j.ijpsycho.2013.04.011
- Carbine, K. A., Muir, A. M., Allen, W. D., LeCheminant, J. D., Baldwin, S. A., Jensen, C. D., Kirwan, C. B., & Larson, M. J. (2021). Does inhibitory control training reduce weight and caloric intake in adults with overweight and obesity? A pre-registered, randomized controlled event-related potential (ERP) study. Behaviour Research and Therapy, 136, 103784. https://doi.org/10.1016/j.brat.2020.103784
- Chami, R., Cardi, V., Lawrence, N., MacDonald, P., Rowlands, K., Hodsoll, J., & Treasure, J. (2020). Targeting binge eating in bulimia nervosa and binge eating disorder using inhibitory control training and implementation intentions: A feasibility trial. Psychological Medicine, 52(5), 874–883. https://doi.org/10.1017/S0033291720002494
- Chen, M., & Bargh, J. A. (1999). Consequences of automatic evaluation: Immediate behavioral predispositions to approach or avoid the stimulus. Personality and Social Psychology Bulletin, 25(2), 215–224. https://doi.org/10.1177/0146167299025002007
- Chen, Z., Holland, R. W., Quandt, J., Dijksterhuis, A., & Veling, H. (2019). When mere action versus inaction leads to robust preference change. Journal of Personality and Social Psychology, 117(4), 721. https://doi.org/10.1037/pspa0000158
- Chen, Z., Veling, H., de Vries, S. P., Bijvank, B. O., Janssen, I., Dijksterhuis, A., & Holland, R. W. (2018). Go/no-go training changes food evaluation in both morbidly obese and normal-weight individuals. Journal of Consulting and Clinical Psychology, 86(12), 980. https://doi.org/10.1037/ccp0000320
- Chen, Z., Veling, H., Dijksterhuis, A., & Holland, R. W. (2016). How does not responding to appetitive stimuli cause devaluation: Evaluative conditioning or response inhibition? Journal of Experimental Psychology: General, 145(12), 1687. https://doi.org/10.1037/xge0000236
- Chen, Z., Veling, H., Dijksterhuis, A., & Holland, R. W. (2018). Do impulsive individuals benefit more from food go/no-go training? Testing the role of inhibition capacity in the no-go devaluation effect. Appetite, 124, 99–110. https://doi.org/10.1016/j.appet.2017.04.024
- Chiu, Y.-C., Cools, R., & Aron, A. R. (2014). Opposing effects of appetitive and aversive cues on go/no-go behavior and motor excitability. Journal of Cognitive Neuroscience, 26(8), 1851–1860. https://doi.org/10.1162/jocn_a_00585
- Di Lemma, L. C., & Field, M. (2017). Cue avoidance training and inhibitory control training for the reduction of alcohol consumption: A comparison of effectiveness and investigation of their mechanisms of action. Psychopharmacology, 234(16), 2489–2498. https://doi.org/10.1007/s00213-017-4639-0
- Eder, A. B., & Klauer, K. C. (2007). Common valence coding in action and evaluation: Affective blindness towards response-compatible stimuli. Cognition and Emotion, 21(6), 1297–1322. https://doi.org/10.1080/02699930701438277
- Eder, A. B., & Klauer, K. C. (2009). A common-coding account of the bidirectional evaluation–behavior link. Journal of Experimental Psychology: General, 138(2), 218. https://doi.org/10.1037/a0015220
- Finucane, M. M., Stevens, G. A., Cowan, M. J., Danaei, G., Lin, J. K., Paciorek, C. J., Singh, G. M., Gutierrez, H. R., Lu, Y., & Bahalim, A. N. (2011). National, regional, and global trends in body-mass index since 1980: Systematic analysis of health examination surveys and epidemiological studies with 960 country-years and 9· 1 million participants. The Lancet, 377(9765), 557–567. https://doi.org/10.1016/S0140-6736(10)62037-5
- Folkvord, F., Veling, H., & Hoeken, H. (2016). Targeting implicit approach reactions to snack food in children: Effects on intake. Health Psychology, 35(8), 919–922. https://doi.org/10.1037/hea0000365
- Forman, E. M., Manasse, S. M., Dallal, D. H., Crochiere, R., Loyka, C. M., Butryn, M. L., Juarascio, A. S., & Houben, K. (2019). Computerized neurocognitive training for improving dietary health and facilitating weight loss. Journal of Behavioral Medicine, 42(6), 1029–1040. https://doi.org/10.1007/s10865-019-00024-5
- Herman, C. P., & P., P. J. (1980). Restrained eating. In A. J. Stunkard (Ed.), Obesity (pp. 208–225). Saunders.
- Houben, K., & Giesen, J. C. (2018). Will work less for food: Go/No-Go training decreases the reinforcing value of high-caloric food. Appetite, 130, 79–83. https://doi.org/10.1016/j.appet.2018.08.002
- Houben, K., Havermans, R. C., Nederkoorn, C., & Jansen, A. (2012). Beer à No-Go: Learning to stop responding to alcohol cues reduces alcohol intake via reduced affective associations rather than increased response inhibition. Addiction, 107(7), 1280–1287. https://doi.org/10.1111/j.1360-0443.2012.03827.x
- Houben, K., & Jansen, A. (2011). Training inhibitory control. A recipe for resisting sweet temptations. Appetite, 56(2), 345–349. https://doi.org/10.1016/j.appet.2010.12.017
- Houben, K., & Jansen, A. (2015). Chocolate equals stop. Chocolate-specific inhibition training reduces chocolate intake and go associations with chocolate. Appetite, 87, 318–323. https://doi.org/10.1016/j.appet.2015.01.005
- Jeffery, R. W., Epstein, L. H., Wilson, G. T., Drewnowski, A., Stunkard, A. J., & Wing, R. R. (2000). Long-term maintenance of weight loss: Current status. Health Psychology, 19(1S), 5. https://doi.org/10.1037/0278-6133.19.Suppl1.5
- Johannes, N., Buijzen, M., & Veling, H. (2021). Beyond inhibitory control training: Inactions and actions influence smartphone app use through changes in explicit liking. Journal of Experimental Psychology: General, 150(3), 431. https://doi.org/10.1037/xge0000888
- Jones, A., Di Lemma, L. C., Robinson, E., Christiansen, P., Nolan, S., Tudur-Smith, C., & Field, M. (2016). Inhibitory control training for appetitive behaviour change: A meta-analytic investigation of mechanisms of action and moderators of effectiveness. Appetite, 97, 16–28. https://doi.org/10.1016/j.appet.2015.11.013
- Keeler, J. L., Chami, R., Cardi, V., Hodsoll, J., Bonin, E., MacDonald, P., Treasure, J., & Lawrence, N. (2022). App-based food-specific inhibitory control training as an adjunct to treatment as usual in binge-type eating disorders: A feasibility trial. Appetite, 168, 105788. https://doi.org/10.1016/j.appet.2021.105788
- Lawrence, N. S., O'Sullivan, J., Parslow, D., Javaid, M., Adams, R. C., Chambers, C. D., Kos, K., & Verbruggen, F. (2015). Training response inhibition to food is associated with weight loss and reduced energy intake. Appetite, 95, 17–28. https://doi.org/10.1016/j.appet.2015.06.009
- Lawrence, N. S., Verbruggen, F., Morrison, S., Adams, R. C., & Chambers, C. D. (2015). Stopping to food can reduce intake. Effects of stimulus-specificity and individual differences in dietary restraint. Appetite, 85, 91–103. https://doi.org/10.1016/j.appet.2014.11.006
- Lim, S. S., Vos, T., Flaxman, A. D., Danaei, G., Shibuya, K., Adair-Rohani, H., AlMazroa, M. A., Amann, M., Anderson, H. R., & Andrews, K. G. (2012). A comparative risk assessment of burden of disease and injury attributable to 67 risk factors and risk factor clusters in 21 regions, 1990–2010: A systematic analysis for the Global Burden of Disease Study 2010. The Lancet, 380(9859), 2224–2260. https://doi.org/10.1016/S0140-6736(12)61766-8
- Liu, H., Holland, R. W., Blechert, J., Quandt, J., & Veling, H. (2022). Devaluation of NoGo stimuli is both robust and fragile. Cognition and Emotion, 36(5), 876–893. https://doi.org/10.1080/02699931.2022.2067132.
- McCulloch, K. C., Li, H., Hong, S., & Albarracin, D. (2012). Naïve definitions of action and inaction: The continuum, spread, and valence of behaviors. European Journal of Social Psychology, 42(2), 227–234. https://doi.org/10.1002/ejsp.860
- Neumann, R., Förster, J., & Strack, F. (2003). Motor compatibility: The bidirectional link between behavior and evaluation. In J. Musch, & K. C. Klauer (Eds.), The psychology of evaluation: Affective processes in cognition and emotion (pp. 371–391). Lawrence Erlbaum Associates Publishers.
- Neumann, R., & Strack, F. (2000). Approach and avoidance: The influence of proprioceptive and exteroceptive cues on encoding of affective information. Journal of Personality and Social Psychology, 79(1), 39. https://doi.org/10.1037/0022-3514.79.1.39
- Oomen, D., Grol, M., Spronk, D., Booth, C., & Fox, E. (2018). Beating uncontrolled eating: Training inhibitory control to reduce food intake and food cue sensitivity. Appetite, 131, 73–83. https://doi.org/10.1016/j.appet.2018.09.007
- Osherson, D. N., Smith, E. E., Wilkie, O., Lopez, A., & Shafir, E. (1990). Category-based induction. Psychological Review, 97(2), 185–200. https://doi.org/10.1037/0033-295X.97.2.185
- Poppelaars, A., Scholten, H., Granic, I., Veling, H., Johnson-Glenberg, M. C., & Luijten, M. (2018). When winning is losing: A randomized controlled trial testing a video game to train food-specific inhibitory control. Appetite, 129, 143–154. https://doi.org/10.1016/j.appet.2018.06.039
- Serfas, B. G., Florack, A., Büttner, O. B., & Voegeding, T. (2017). What does it take for sour grapes to remain sour? Persistent effects of behavioral inhibition in go/no-go tasks on the evaluation of appetitive stimuli. Motivation Science, 3(1), 1. https://doi.org/10.1037/mot0000051
- Sloman, S. A. (1993). Feature-based induction. Cognitive Psychology, 25(2), 231–280. https://doi.org/10.1006/cogp.1993.1006
- Sunderrajan, A., & Albarracín, D. (2021). Are actions better than inactions? Positivity, outcome, and intentionality biases in judgments of action and inaction. Journal of Experimental Social Psychology, 94, 104105. https://doi.org/10.1016/j.jesp.2021.104105
- Tzavella, L., Lawrence, N. S., Button, K. S., Hart, E. A., Holmes, N. M., Houghton, K., Badkar, N., Macey, E., Braggins, A.-J., & Murray, F. C. (2021). Effects of go/no-go training on food-related action tendencies, liking and choice. Royal Society Open Science, 8(8), 210666. https://doi.org/10.1098/rsos.210666
- Van Dessel, P., De Houwer, J., Gast, A., & Smith, C. T. (2015). Instruction-based approach-avoidance effects. Experimental Psychology, 62(3), 161–169. https://doi.org/10.1027/1618-3169/a000282
- Van Dessel, P., De Houwer, J., Gast, A., Smith, C. T., & De Schryver, M. (2016). Instructing implicit processes: When instructions to approach or avoid influence implicit but not explicit evaluation. Journal of Experimental Social Psychology, 63, 1–9. https://doi.org/10.1016/j.jesp.2015.11.002
- Van Dessel, P., Eder, A. B., & Hughes, S. (2018). Mechanisms underlying effects of approach-avoidance training on stimulus evaluation. Journal of Experimental Psychology: Learning, Memory, and Cognition, 44(8), 1224. https://doi.org/10.1037/xlm0000514
- Van Dessel, P., Hughes, S., & De Houwer, J. (2018). Consequence-based approach-avoidance training: A new and improved method for changing behavior. Psychological Science, 29(12), 1899–1910. https://doi.org/10.1177/0956797618796478
- Van Dessel, P., Hughes, S., & De Houwer, J. (2019). How do actions influence attitudes? An inferential account of the impact of action performance on stimulus evaluation. Personality and Social Psychology Review, 23(3), 267–284. https://doi.org/10.1177/1088868318795730
- Veling, H., Aarts, H., & Papies, E. K. (2011). Using stop signals to inhibit chronic dieters’ responses toward palatable foods. Behaviour Research and Therapy, 49(11), 771–780. https://doi.org/10.1016/j.brat.2011.08.005
- Veling, H., Aarts, H., & Stroebe, W. (2013). Stop signals decrease choices for palatable foods through decreased food evaluation. Frontiers in Psychology, 4, 875. https://doi.org/10.3389/fpsyg.2013.00875
- Veling, H., Holland, R. W., & van Knippenberg, A. (2008). When approach motivation and behavioral inhibition collide: Behavior regulation through stimulus devaluation. Journal of Experimental Social Psychology, 44(4), 1013–1019. https://doi.org/10.1016/j.jesp.2008.03.004
- Veling, H., Lawrence, N. S., Chen, Z., van Koningsbruggen, G. M., & Holland, R. W. (2017). What is trained during food go/no-go training? A review focusing on mechanisms and a research agenda. Current Addiction Reports, 4(1), 35–41. https://doi.org/10.1007/s40429-017-0131-5
- Veling, H., van Koningsbruggen, G., Aarts, H., & Stroebe, W. (2014). Targeting impulsive processes of eating behavior via the internet. Effects on body weight. Appetite, 78, 102–109. https://doi.org/10.1016/j.appet.2014.03.014
- Veling, H., Verpaalen, I. A., Liu, H., Mosannenzadeh, F., Becker, D., & Holland, R. W. (2021). How can food choice best be trained? Approach-avoidance versus go/no-go training. Appetite, 163, 105226. https://doi.org/10.1016/j.appet.2021.105226
- Verbruggen, F., Best, M., Bowditch, W. A., Stevens, T., & McLaren, I. P. (2014). The inhibitory control reflex. Neuropsychologia, 65, 263–278. https://doi.org/10.1016/j.neuropsychologia.2014.08.014
- Wessel, J. R. (2018). Prepotent motor activity and inhibitory control demands in different variants of the go/no-go paradigm. Psychophysiology, 55(3), e12871. https://doi.org/10.1111/psyp.12871
- Wolz, I., Nannt, J., & Svaldi, J. (2020). Laboratory-based interventions targeting food craving: A systematic review and meta-analysis. Obesity Reviews, 21(5), e12996. https://doi.org/10.1111/obr.12996
- World Health Organisation. (2017). Global health observatory - Overweight and obesity. WHO. http://www.who.int/gho/ncd/risk_factors/overweight/en/.
- Yang, Y., Shields, G. S., Wu, Q., Liu, Y., Chen, H., & Guo, C. (2019). Cognitive training on eating behaviour and weight loss: A meta-analysis and systematic review. Obesity Reviews, 20(11), 1628–1641. https://doi.org/10.1111/obr.12916