ABSTRACT
Purpose: In many sports situations, two or more players need to coordinate their actions to make sure that one of them intercepts a ball or opponent. We considered how two soccer players head back a thrown ball. Two accounts for the joint decision making by both players were considered. These two accounts not only differ in their theoretical basis but also have vastly different implications for training practice. In a first account, players know their areas of responsibility for interception, and combine this with their prediction of the ball's landing location. In a second account, the coordination emerges from the unfolding dynamics of the system of informationally connected players and ball. According to this second account, especially for balls aimed in between the two players, both of the players may start moving and one player sees that the ball will be interceptable for the other player, and subsequently yields the interception. Methods: We instrumented soccer players and the ball with Kinexon sensors and had pairs of players head back the thrown ball. Results: In line with the second account, the results showed a fair number of instances where the player who intercepted the ball had to move the longest distance. Furthermore, considerable movement by both players was not an exception. Conclusion: The results can be taken as a first step towards an understanding of joint coordination as an emergent phenomenon.
KEYWORDS:
Team sports involve coordination among the team members. This coordination can take on many faces (cf. Cannon-Bowers & Bowers, Citation2006). Here, we focus on a situation that can be found in a number of team sports: the coordination between two (or more) players in intercepting an approaching ball. This is the situation of two volleyball players having to make sure that one of them passes the serve to the setter (e.g., Paulo et al., Citation2018), the two players in doubles tennis returning the ball (e.g., Lausic et al., Citation2014), baseball outfielders in their attempt to make a catch, or soccer defenders blocking the ball to defend their penalty box. These situations boil down to two (or more) individuals having to arrive at a decisionFootnote1 on who will intercept the ball, making sure that they will not collide (e.g., see, Boden et al., Citation2004) and possibly fail.
When considering how two players can solve the task of joint interception, two accounts have been put forward (e.g., Benerink et al., Citation2016, Citation2018; Van Opstal et al., Citation2018). In the first account, the two players have a shared understanding of the division of space, and use this to determine who will take which ball. For instance, in receiving a serve in volleyball, players may work with tactical plans as to which serve would be taken by whom. Paulo et al. (Citation2018) proposed that such division of labor could well be based on, so-called, Voronoi areas (e.g., Fonseca et al., Citation2012),Footnote2 indicating a boundary halfway in between the two players: players would be responsible for balls arriving closest to them. In the Paulo et al. study, the vast majority of serve receptions adhered to this principle, which, thus, seemed to be a good principle underlying the joint decision-making. The concept of Voronoi areas (or, relatedly, dominant regions, in Taki & Hasegawa, Citation2000) has been applied in other sports as well, such as soccer (e.g., Caetano et al., Citation2021; Gudmundsson & Wolle, Citation2014; Kim, Citation2004; Rein et al., Citation2017; Taki & Hasegawa, Citation2000), futsal (Fonseca et al., Citation2012), basketball (Lopes et al., Citation2015), and handball (Taki & Hasegawa, Citation2000), although not yet in the context of such decision-making.
The shared understanding of the division of space by two (or more) players to base their decision on of how to jointly deal with an approaching ball assumes an explicit role for the boundary between areas to be responsible for by the players: balls arriving at my side of the boundary will be mine, and balls arriving at your side of the boundary will be yours to handle. Apart from the assumption that the players know this boundary, there is also the assumption that players are able to predict where balls are headed. This might be more difficult for players than intuitively expected, perhaps well illustrated by the struggles that goalkeepers sometimes have especially with balls approaching along curved trajectories (e.g., Craig et al., Citation2011, Citation2006, Citation2009; Dessing & Craig, Citation2010). An alternative account for the boundaries that can be observed in joint interception is that they emerge from the unfolding dynamics of the system of players and balls coupled to each other informationally (e.g., Benerink et al., Citation2016, Citation2018; Van Opstal et al., Citation2018). In this account, for balls arriving in between two players, both players may start moving. Both players use prospective information to control their locomotion for interception (e.g., Bootsma et al., Citation2016) and information specifying interceptability for themselves and for the other. In the model that Benerink et al. (Citation2016) developed as a proof of concept, at some point in time, one player sees that the ball will be interceptable for the other, after which they abort their movement, preventing collision and allowing the other to successfully intercept the ball. The boundary, from this perspective, is not an explicit boundary used by the players, but rather an implicit boundary that can be established by researchers post-hoc. The decision-making, in this approach, is more so a matter of continuous coordination than a discrete event (cf. Raab & Araújo, Citation2019).
In summary, we contrasted an approach in which players have explicit knowledge of boundaries between reception areas as well as the ability to predict the future landing location of the ball with an approach in which these two qualities are not assumed and in which coordination emerges from the informational coupling of the two players. Both accounts have been contrasted in the previous studies by Benerink and colleagues (Benerink et al., Citation2016, Citation2018; Van Opstal et al., Citation2018), which considered lateral interception on a shared computer screen. The two players each controlled a paddle that could move along a horizontal interception axis near the bottom of the computer screen. Their task was that one of them intercepted a ball that moved down in a straight line under an angle with the vertical, at a constant speed. Also, the two players needed to make sure not to collide with each other’s paddle. The information underlying the coordination was proposed to be in the evolution of the angles between the lines between the ball and the player and the interception axis (which was the horizontal in their doubles-pong task). A rate of change of zero of one player’s angle meant that the other player would yield interception (for details, see, Benerink et al., Citation2016, Citation2018; Van Opstal et al., Citation2018). In other words, whereas one account assumes that players know boundaries and landing locations, the alternative account assumes that coordination emerges from the use of directly available information. The distinction between the two accounts is a theoretical distinction (e.g., see also, Zhao & Warren, Citation2015), but at the same time, the distinction has practical implications: Training the coordination should focus on developing knowing boundaries and predicting trajectories versus attending to the proper information for yielding interception.
As mentioned before, the studies on joint interception that contrasted the two accounts that we introduced before, until now, have all used computer tasks, in which players controlled virtual paddles to intercept a virtual ball (Benerink et al., Citation2016, Citation2018; Van Opstal et al., Citation2018; for a study using a similar task and setup, see; Faure et al., Citation2019). The present study is the first attempt to test the idea of an emerging boundary in an experiment involving the interception of balls in a real-world sports context. To this end, we will have soccer players head soccer balls, while both the players and the ball will be instrumented with position sensors. Although we will not restrict the players to lateral movement, which makes that we cannot test the informational variable that we discussed before directly, showing that interception is not always realized by the player who turned out to be closest to the eventual interception location will provide credibility for the account of emergent coordination.
Methods
Participants
Sixteen participants (14 males, 2 females) were recruited for the study. The average age was 24.3 years, with a range of 17–28 years of age and the average years of experience playing competitive soccer at 14.1 years with a range of 1–23 years. This study was approved by the Ethics Board of the German Sports University. All COVID-19 safety protocols were followed during the experiment.
Materials
The experiment was performed on an athletic field with artificial grass turf at the German Sports University. A local-positioning tracking system (Kinexon GmbH, Munich, Germany) was used for the primary data collection. Through 16 Wi-Fi connected radio transmitters placed around the field, the system tracked the positions of small devices that were secured to each player and the ball, at 25 Hz.
Procedure
After filling out an informed consent form, the participants were given 10 minutes to perform a physical and mental warm up of their choice. Next, they joined in an individual session and a dyadic session, in this order. They were instructed on the task to intercept the thrown ball with a header back toward the thrower, to avoid collision with the other player, and avoid collision with the edges of the field. Once the interception occurred, they were to hold their position as the thrower retrieved the ball and await the next trial. The field was separated into five equally sized zones with a designated thrower standing in the middle of one long side of the field (see ). The thrower’s job was to throw a ball with a high arc toward each of the five zones in a random but equally distributed order. That is, each zone would have the same number of balls thrown toward it during the condition trials, but the order of throws was selected at random by the thrower. After being fitted with a tracking sensor, the participants performed five practice trials to familiarize themselves with the task and ensure that the instructions were followed.
Figure 1. Illustration of the experimental set up and the variables used in the analyses. The red and blue dots indicate the initial positions of both players in the doubles condition. The black dot indicates the standard position of the ball thrower. The ball is headed back at the position marked by the green dot, by the player whose initial position is connected to this green dot by a solid line. The position of the non-intercepting player at the moment of interception is indicated by the white dot. For a description of the variables used in the analyses, please see the text.
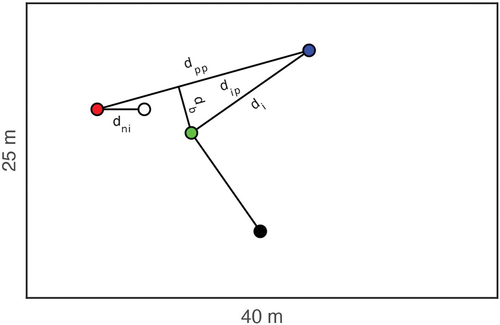
As mentioned before, participants went through two conditions of the experiment on the same day. The first (individual) condition involved 25 trials with the participant alone and starting in the center of the field. The second (dyadic) condition involved two sets of 25 trials with a 5-minute break in between, involving two participants on the field together, each participant starting each set of 25 trials in a predefined position (see ).
Analysis
To detect successful headers, we used two criteria. First, the minimum distance between the ball and one of the players needed to be less than 1 m. Second, the ball needed to have undergone a significant change in direction. To meet this second criterion, the angle between the lines fitted through the projections of the ball positions onto the playing surface before and after the point of minimum distance between the ball and the player needed to be larger than 45 degrees. If both criteria were met, the successful interception was attributed to the player with the smallest minimum distance to the ball.
illustrates the situation in the dyadic condition. For each trial, we determined the initial position of each player (the red and blue dots) and the interception position (the green dot). The interception position was the player’s position at the moment of the minimum distance between the ball and the player. Starting with these positions, we used several variables to characterize the situation We computed the distance between the initial positions of both players (dpp). Next, we determined the projection of the interception position onto the line through both initial positions of the two players, and computed the distance between this projection and the initial position of the intercepting player (dip). The ratio rip (dip divided by dpp) was taken as an indicator of the coordination between the two players. As can be seen in , values of rip < 0 mean that the ball did not arrive in between the two players and was intercepted by the player who was initially closest to the interception location. In case 0 < rip < 1, the ball did arrive in between the two players’ initial positions. The value of rip = 0.5 demarcates the situations in which the ball is being headed back by the player initially closest to the interception location and initially farthest from the interception location (rip > 0.5). Finally, a value of rip > 1 indicates that the player who successfully heads back the ball must have moved beyond the other player for this successful interception.
Figure 2. The implications of rip values, as used in this study. The red and blue dots indicate the initial positions of both players in the dyadic condition. The ball is headed back at the position marked by the green dot, by the player whose initial position is connected to this green dot by a solid line. For rip values larger than 0.5, the intercepting player was farther away from the interception location than the non-intercepting player.
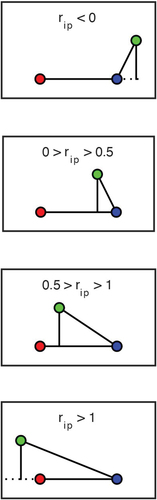
The final variables that we computed were the distance between the interception position and its projection onto the line connecting both initial positions of the players (db) and the distances that both players moved: the distance moved by the intercepting player (di) was defined as the distance between their initial position and the interception location, whereas the distance moved by the non-intercepting player (dni) was defined as the distance between their initial position and their position at the moment of interception by the other player.
Results and discussion
In the present contribution, we will focus on the dyadic condition, in which the two players were present. One out of the 400 trials turned out to be unavailable for further analysis because of technical issues during the experiment itself. The remaining 399 trials resulted in 368 successful interceptions. details the number of interceptions and misses for each of the eight pairs of players.
Table 1. Number of interceptions, misses, and total of trials for each of the eight pairs of players.
The main question in the experiment was how the two players dealt with their task of heading back the ball to the thrower. Did they work with zones of responsibility? Or, alternatively, did we see signs of coordination and an emergent division of labor? The first variable that we considered was rip. As explained before, rip values between 0 and 1 indicated that the projected interception location would lie in between the initial positions of the two players whereas for rip values smaller than 0, this was not the case and the interception location would be closest to the initial position of the players who made the actual interception. As can be seen in , a total of 234 out of the 399 trials belonged to the latter category. That is to say, in these 234 trials, the ball was headed back to the thrower by the player who was clearly closest to the interception location.
Table 2. Number of successful interceptions as a function of rip.
In 129 out of the 399 trials, rip values were in between 0 and 1, and rip values larger than 1 were seen in 3 trials (). In 22 out of the total of these 132 trials, rip values were larger than 0.5, indicating that the player who finally headed back the ball to the thrower was initially farthest away from the interception location. gives a few examples of trials in which the latter was the case. gives a clear example of a trial in which the ball trajectory is about perpendicular to the line connecting the initial positions of the players. The ball ends up closer to the left player’s initial position (the red dot). Both players show clear movement (the line connecting the red and white dots for the left player and the line connecting the blue and green dots for the right player), and in the end, the right player, who was farthest away from the interception position, makes the interception. A similar case can be seen in , now for a ball that is being intercepted before it reaches the line connecting both players’ initial positions. These are clear cases of pictures of the two players that fit an account in which the players did not work with fixed interception areas but rather coordinated their actions while moving to make the interception happen. Both players show considerable movement in the direction of the interception location, which might be taken to indicate that they did not (were not able to) predict this position early during ball flight. Why, in the end, the player who had to run the longest distance turned out to be the one to head back the ball cannot be deduced from these graphs. In their task in which only lateral movement was possible, Benerink and colleagues (Citation2016, Citation2018; Van Opstal et al., Citation2018) suggested that one of the players saw that the other one would be on route to make the interception (perhaps moving faster). The same could be at the basis of the coordination in the joint interception of soccer balls, in the present study.
Figure 3. Four trials with 0.5 < rip < 1, in which both players moved. The red and blue dots indicate the initial positions of both players in the dyadic condition. The black dot indicates the standard position of the ball thrower. The ball is headed back at the position marked by the green dot, by the player whose initial position is connected to this green dot by a solid line. The position of the non-intercepting player at the moment of interception is indicated by the white dot.
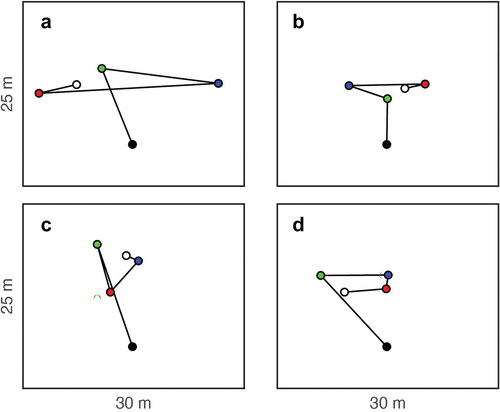
also gives a situation that can be well understood from an emergent-patterns perspective. In this case, the interception location (the green dot) turns out to be closer to the right player ‘s initial position (the blue dot) than to the left player’s initial position (the red dot). The right player did start moving, but it was the left player who made the interception. If both players would have been able to predict the interception location from looking at the first part of the ball trajectory, it would have been more economical for the left player to have left it to the right player. Still, it makes sense that it was the left player who headed back the ball. The ball was coming in his direction, so he went for it. This fits with the account in which the unfolding of the dynamics of the informationally coupled system determines the outcome. More specifically, the information that players might use to know about interceptability of balls for the other is probably rooted in the information needed for the control of locomotion to intercept. The use of this type of information has been proposed to cause the, so-called, angle-of-approach effect, the effect that the kinematics of locomotion are not only determined by the time and distance to be covered but also by the angle under which the ball approaches the interception location (e.g., Bootsma et al., Citation2016).
The situation depicted in also shows an interesting case. Also in this trial, the player who headed back the ball was initially farthest away from the interception location. Furthermore, both players clearly ran to intercept the ball. It was definitely not the case that they knew where exactly it would be going, so both went for the ball. Of course, this fits the way that players always want to make the extra effort to make sure that the team succeeds. Just running to be the backup for your teammate or to increase chances of success is not uncommon in team sports. Similar situations can be seen in the trials in which the ri values were larger than 1 (). All these situations may also be taken to illustrate the unpredictability of ball trajectories as experienced by players. This unpredictability might be illustrated as well by the occasions in which one of the two players did not even move at all, while the player whose initial position was farthest away from the interception location went for a successful header ().
Figure 4. Two trials with rip > 1. The red and blue dots indicate the initial positions of both players in the dyadic condition. The black dot indicates the standard position of the ball thrower. The ball is headed back at the position marked by the green dot, by the player whose initial position is connected to this green dot by a solid line. The position of the non-intercepting player at the moment of interception is indicated by the white dot.
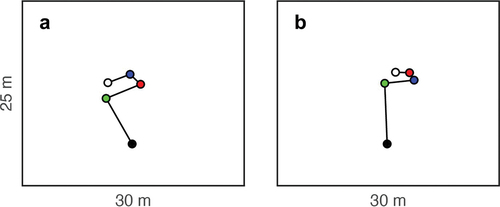
Figure 5. Four trials with 0.5 < rip < 1, in which only the intercepting player moved. The red and blue dots indicate the initial positions of both players in the dyadic condition. The black dot indicates the standard position of the ball thrower. The ball is headed back at the position marked by the green dot, by the player whose initial position is connected to this green dot by a solid line. The position of the non-intercepting player at the moment of interception is indicated by the white dot.
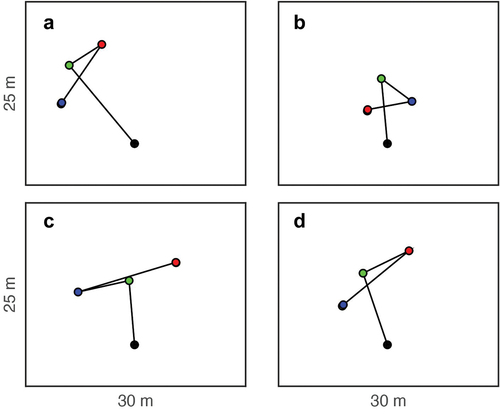
gives the distances between the players’ initial positions (dpp), the distance between the initial position of the intercepting player and the interception position (i.e., the distance that this player moved to get at the interception location: di) and the distance between the initial position of the non-intercepting player and their position at the moment of interception position (i.e., the distance that the non-intercepting player moved: dni), all as function of rip (only for the trials with 0 < rip < 1). These results illustrate the wide variation in the distance between both players’ initial positions. shows something like a linear increase in the distance moved by the intercepting player with rip for rip values smaller than 0.5, but no clear relation for rip values larger than 0.5. Finally, demonstrates that the distances moved by the non-intercepting player ranged from hardly moving at all (cf. ) to considerable movement (cf. ).
Figure 6. The distance between the players’ initial positions (panel a: dpp), the distance moved the intercepting player (panel b: di), and the distance moved by the non-intercepting player (panel c: dni) as a function of rip, for all trials with 0 < rip < 1.
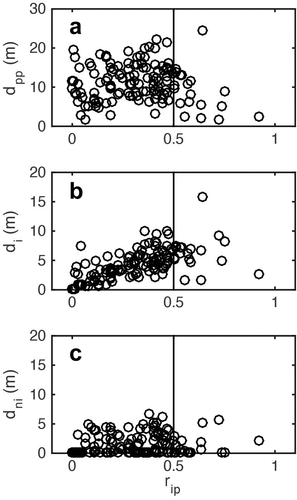
Taken together, what does this all mean? The main question that we try to address in the present contribution is how two players, be it soccer players, volleyball players, baseball players, or what have you, coordinate their actions to make sure that one of them intercepts an approaching ball. We sketched two accounts: one in which the two players make decisions based on a shared understanding of a division in zones of responsibility (e.g., Paulo et al., Citation2018) and another in which players are continuously coupled informationally with each other and with the ball, such that the division of labor emerges from the unfolding dynamics. The latter was proposed and tested in studies in which participants played doubles pong on a large, shared computer screen (Benerink et al., Citation2016, Citation2018; Van Opstal et al., Citation2018). Here, we made an attempt to bring this paradigm to a sports situation, with participants heading real balls. The disadvantage of this type of studies, obviously, is the lesser amount of control, leading to more variability in ball trajectories and player positions, for instance. Still, the data give us indications that players might not be working with predesignated zones, each covered by one of the players. First, when we consider the numbers in , when focusing on the trials in which rip was larger than 0, in 22 of the 132 trials (16.7%) the player who was initially farthest away from the interception location eventually was the one actually heading the ball back to the thrower. When we would only consider rip values in between 0.25 and 0.75, the percentage would be 21.5% (17 out of 79 trials). Although one could imagine many other reasons for these percentages, one conclusion might be that players are not well able to predict the interception location being in their zone. Furthermore, in these cases, often, both players moved considerable distances, which also seems uneconomical and unnecessary if players would know which ball would be for whom. And even when players remained at their initial position, we found plenty of examples of the player needing to move the longest distance to be the one making the interception. Is this proof? No, it is not. However, these results might be taken to entertain the idea that emergent coordination might actually be at play in these situations. A follow-up on this first, admittedly, small study seems worth the effort.
Conclusion
This research examined two players, a ball, and a thrower as a complex system to analyze how online-based action control influences the emergence of coordinative behaviors between two players in soccer. Our data suggest that coordinative behaviors between players in intercepting a ball are emergent based on the visual information of the environment and the movements of the other player rather than based on a heuristic prediction of an interception point. However, more research with a larger sample size and refinements to the experimental design are required in order to explore this concept further and come to a firm conclusion. The potential implications of this research could include adopting new training strategies that focus more on training action behaviors based on environmental affordances that make judgment and decision-making behaviors more accurate and effective within a fast-paced environment like a soccer game. While this research is still limited, it lays the foundation for further exploration within real-world sport settings.
IRB approval
This study was approved by the ethics commission of the German Sports University.
Acknowledgments
We would like to thank Maxmillian Kemp for assistance with and operating the Kinexon equipment and Muaz Sözen for help with recruiting participants and experiment logistics.
Disclosure statement
No potential conflict of interest was reported by the author(s).
Additional information
Funding
Notes
1 The concept of decision can have many definitions. For instance, decisions can be seen as choices between alternatives or, alternatively, as transitions in the course of action (for a more elaborate discussion, see, Raab & Araújo, Citation2019).
2 In previous literature, what we call Voronoi areas are often referred to as Voronoi diagrams. To emphasize their quality of bounded areas, we will use the phrasing of Voronoi areas.
References
- Benerink, N. H., Zaal, F. T. J. M., Casanova, R., Bonnardel, N., & Bootsma, R. J. (2016). Playing ‘pong’ together: Emergent coordination in a doubles interception task. Frontiers in Psychology, 7, Article 1910. https://doi.org/10.3389/fpsyg.2016.01910
- Benerink, N. H., Zaal, F. T. J. M., Casanova, R., Bonnardel, N., & Bootsma, R. J. (2018). Division of labor as an emergent phenomenon of social coordination: The example of playing doubles-pong. Human Movement Science, 57, 134–148. https://doi.org/10.1016/j.humov.2017.11.012
- Boden, B. P., Tacchetti, R., & Mueller, F. O. (2004). Catastrophic injuries in high school and college baseball players. The American Journal of Sports Medicine, 32(5), 1189–1196. https://doi.org/10.1177/0363546503262161
- Bootsma, R. J., Ledouit, S., Casanova, R., & Zaal, F. T. J. M. (2016). Fractional-order information in the visual control of lateral locomotor interception. Journal of Experimental Psychology: …, 42(4), 517–529. https://doi.org/10.1037/xhp0000162
- Caetano, F. G., Junior, S. B., Torres, R. D. S., Cunha, S. A., Ruffino, P. R. C., Martins, L. E. B., & Moura, F. A. (2021). Football player dominant region determined by a novel model based on instantaneous kinematics variables. Scientific Reports, 11(1), 18209. https://doi.org/10.1038/s41598-021-97537-4
- Cannon‐Bowers, J. A., & Bowers, C. (2006). Applying work team results to sports teams: Opportunities and cautions. International Journal of Sport and Exercise Psychology, 4(4), 447–462. https://doi.org/10.1080/1612197X.2006.9671807
- Craig, C. M., Bastin, J., & Montagne, G. (2011). How information guides movement: Intercepting curved free kicks in soccer. Human Movement Science, 30(5), 931–941. https://doi.org/10.1016/j.humov.2010.08.007
- Craig, C. M., Berton, E., Rao, G., Fernandez, L., & Bootsma, R. J. (2006). Judging where a ball will go: The case of curved free kicks in football. Die Naturwissenschaften, 93(2), 97–101. https://doi.org/10.1007/s00114-005-0071-0
- Craig, C. M., Goulon, C., Berton, E., Rao, G., Fernandez, L., & Bootsma, R. J. (2009). Optic variables used to judge future ball arrival position in expert and novice soccer players. Attention, Perception & Psychophysics, 71(3), 515–522. https://doi.org/10.3758/APP.71.3.515
- Dessing, J. C., & Craig, C. M. (2010). Bending it like Beckham: How to visually fool the goalkeeper. PLoS ONE, 5(10), e13161. https://doi.org/10.1371/journal.pone.0013161
- Faure, C., Limballe, A., Sorel, A., Perrin, T., Bideau, B., & Kulpa, R. (2019). Dyadic interference leads to area of uncertainty during face-to-face cooperative interception task. Frontiers in ICT, 6, Article 20. https://doi.org/10.3389/fict.2019.00020
- Fonseca, S., Milho, J., Travassos, B., & Araújo, D. (2012). Spatial dynamics of team sports exposed by Voronoi diagrams. Human Movement Science, 31(6), 1652–1659. https://doi.org/10.1016/j.humov.2012.04.006
- Gudmundsson, J., & Wolle, T. (2014). Football analysis using spatio-temporal tools. Computers, Environment and Urban Systems, 47(C), 16–27. https://doi.org/10.1016/j.compenvurbsys.2013.09.004
- Kim, S. (2004). Voronoi analysis of a soccer game. Nonlinear Analysis: Modelling and Control, 9(3), 233–240. https://doi.org/10.15388/NA.2004.9.3.15154
- Lausic, D., Razon, S., & Tenenbaum, G. (2014). Nonverbal sensitivity, verbal communication, and team coordination in tennis doubles. International Journal of Sport and Exercise Psychology, 13(4), 398–414. https://doi.org/10.1080/1612197x.2014.993681
- Lopes, A., Fonseca, S., Lese, R., & Baca, A. (2015). Using Voronoi diagrams to describe tactical behaviour in invasive team sports: An application in basketball. Cuadernos de Psicología Del Deporte, 15(1), 123–130. https://doi.org/10.4321/S1578-84232015000100012
- Paulo, A., Zaal, F. T. J. M., Seifert, L., Fonseca, S., & Araújo, D. (2018). Predicting volleyball serve-reception at group level. Journal of Sports Sciences, 36(22), 2621–2630. https://doi.org/10.1080/02640414.2018.1473098
- Raab, M., & Araújo, D. (2019). Embodied cognition with and without mental representations: The case of embodied choices in sports. Frontiers in Psychology, 10, Article 1825. https://doi.org/10.3389/fpsyg.2019.01825
- Rein, R., Raabe, D., & Memmert, D. (2017). “Which pass is better?” Novel approaches to assess passing effectiveness in elite soccer. Human Movement Science, 55, 172–181. https://doi.org/10.1016/j.humov.2017.07.010
- Taki, T., & Hasegawa, J. (2000). Quantitative measurement of teamwork in ball games using dominant region. International Archives of Photogrammetry and Remote Sensing, XXXIII(Suppl. B5), 125–131. https://www.isprs.org/proceedings/XXXIII/congress/part5/125_XXXIII-part5s.pdf
- Van Opstal, A. A. M. (Daphne), Benerink, N. H., Zaal, F. T. J. M., Casanova, R., & Bootsma, R. J. (2018). Information-based social coordination between players of different skill in doubles pong. Frontiers in Psychology, 9, 312–314. https://doi.org/10.3389/fpsyg.2018.01731
- Zhao, H., & Warren, W. H. (2015). On-line and model-based approaches to the visual control of action. Vision Research, 110(PB), 190–202. https://doi.org/10.1016/j.visres.2014.10.008