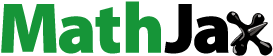
ABSTRACT
Purpose: First, testing an intervention of neuromodulation based on motor imagery and action observation as a promoter of motor adaptation of a complex motor task involving balance. Second, determining what prior balance factors can affect the motor adaptation task. Methods: A double-blind randomized controlled trial was performed. Forty-eight healthy subjects were recruited. The balance of all participants during gait and standing was assessed before adapting to the complex, multi-limb motor task of riding an inverse steering bicycle (ISB). Two interventions were carried out interleaved among trials of adaptation to the motor task: the experimental group (n = 24) was asked to perform neuromodulation (EN) by watching first-person ISB riding through immersive VR glasses and, simultaneously, mentally mimicking the movements. The control group (CG) was asked to watch a slideshow video of steady landscape images. Results: The results showed that the EN group did not improve the motor adaptation rate and induced higher adaptation times with respect to the CG. However, while the motor adaptation success showed a significant dependence on the prior proprioceptive participation in balance in the CG, the EN group did not present any relationship between the prior balance profile and motor adaptation outcome. Conclusions: Results point to a benefit of the visually guided neuromodulation for the motor adaptation of the subjects with low participation of proprioception in balance. Moreover, the results from the control group would allow to disclose prognostic factors about the success of the motor adaptation, and also prescription criteria for the proposed neuromodulation based on the balance profile.
Motor skill learning is defined as the ability to learn a newly acquired skill by executing it in a more graceful and accurate way due to practice. Fundamental Movement Skills (FMS) of development, such as riding a bicycle (Kavanagh et al., Citation2020), are mainly acquired through frequent exploratory practice (Seidler et al., Citation2012; Willingham, Citation1998). Explicit instruction and feedback from a trainer help to focus the attention of the practitioner in the very first steps (van Abswoude et al., Citation2019). After that, practice and experience are at the basis for this motor sequence learning (Kavanagh et al., Citation2020; Seidler et al., Citation2012).
The acquisition and long-term retention of motor skills plays a fundamental role in our daily life. We have opportunities to learn motor skills everyday, whether it is for pleasure or work or the need for relearning a skill we lost following an injury or for preventing possible future injuries. A total and accurate motor relearning of a lost skill can be unfeasible, especially after a neurological damage or severe trauma (Lee et al., Citation2015; Tan et al., Citation2018), due to physio-anatomical changes or chronic pain. In this case, the lost motor skills must be compensated, for functional purposes, not by motor relearning but through sensorimotor adaptation. Sensorimotor adaptation is the process by which one learns to perform a previously mastered motor task under different physical conditions or changes in the sensory incoming information or in output features (Seidler et al., Citation2006, Citation2012). Motor adaptation implies different cognitive demands and neuronal substrates than motor sequence learning (Seidler & Noll, Citation2008) but both processes can share neural substrates in different stages of them, according to the plasticity model described in (Doylon & Benali, Citation2005). Based on brain images studies, this model postulates that consolidated long-term hardwired motor sequence learning seems to involve the cortico-striatal brain system, while for long-term memory consolidating and maintaining of motor adaption, cortico-cerebellar system is essential. However, motor adaption, in the first fast learning phase, recruits the cortico-striatal and cortico-cerebellar circuits, which might lead to partially and temporally interfere and inhibit consolidated motor sequence patterns distributed in the cortico-striatal circuit in healthy people.
The traditional approach to motor rehabilitation and learning is focused on mobilizing and strengthening the peripheral system in order to improve functionality. However, motor adaptation is a rather a central nervous system process. Conventional training and rehabilitation methods present limitations when restoring motor function, especially after neurological damage, because they do not take into account the underlying brain processes. Therefore, it is necessary to apply new strategies in order to promote, maintain and rehabilitate functional capacity (Belda-Lois et al., Citation2011). These new strategies are based on neuronal plasticity. Neuronal plasticity is the ability of the nervous system to respond to intrinsic or extrinsic stimuli by reorganizing its structure, function, and connections. Modification of the central nervous system according to the principle of neuronal plasticity is carried out by enhancement or inhibition of neuronal activity, which produces functional changes in the short-term (minutes or days) and structural changes in the medium term (months) (Pfurtscheller et al., Citation2008).
A harmless and ecological way to promote neuroplasticity is to voluntarily modulate the neuronal activity through internal mechanisms of thought, generating an endogenous neuromodulation (EN). This term refers to a modulation method in which functional changes depend solely on information being provided to the brain on its own state of function. Currently, this approach is largely used in diverse neurofeedback applications from functional magnetic resonance imaging and electroencephalographic (EEG) activity (Chapin et al., Citation2012; deCharms et al., Citation2005; Dekker et al., Citation2014; Del Castillo et al., Citation2018; Tan et al., Citation2009). Moreover, observation and imagery can also modulate neural activity, especially in sensorimotor tasks (Ono et al., Citation2018; Orand et al., Citation2012), and promote imitation learning (Vogt & Thomaschke, Citation2007) and motor adaptation (Stöckel & Fries, Citation2013). In addition, action observation and imagery largely share brain activation areas with actual motor execution (Hardwick et al., Citation2018). Consequently, a visually induced endogenous neuromodulation strategy through motor imagery with immersive virtual reality was tested as a promoter of the sensorimotor adaptation process in the present study.
The motor task to be adapted was selected in order to maximize the utility of the results of the study for rehabilitation or enhancement purposes. In this regard, the selected motor task, riding a bicycle, is a common and complex task that involves all limbs and also balance as in gait. In addition, it is a task usually learned during early childhood, which guarantees a strongly consolidated motor pattern. In this study, as a result, the participants had to adapt to ride an inverse steering bicycle (ISB) (Briem et al., Citation2004).
The process of riding a bicycle is complex because it comprises a set of multiple tasks with sensory, cognitive, and motor domains according to Zeuwts et al. (Citation2017). It also involves balance (Cain et al., Citation2016), usually defined as the ability of the body to maintain its center of gravity vertically over the base of support through a combination of information coming from visual, vestibular and somatosensory structures (Cullen, Citation2012; Nashner, Citation1997). All tasks requiring balance need the engagement of these structures (Ertl et al., Citation2017; Medendorp & Selen, Citation2017). The ability to maintain and control balance is usually recognized as a fundamental motor component, but the relationship between balance ability and gross motor skill learning is less clear (Payne & Isaacs, Citation2020). Moreover, action observation itself, as provided in the visually induced neuromodulation intervention here, might influence and also modulate balance (Gatti et al., Citation2019). This is the main reason why the balance factor was also controlled in the present study.
Therefore, the present paper describes a study with two main objectives. First, testing an intervention of neuromodulation based on action observation as a promoter of motor adaptation of a complex motor task. Second, determining whether prior balance factors can affect the motor adaptation task. Outcomes from the present study would be useful to establish balance-based prescription criteria for motor adaptation-based training and rehabilitation.
Materials and methods
Participants
Volunteers were recruited via an advertisement and those who met inclusion criteria were included in the study. Inclusion criteria included aged between 18 and 29; able to ride a regular bicycle and to have an adequate balance score. The balance factor was measured by the Standard Balance Deficit Test and scored by the Risk-of-Falling indicator defined in (Rossi-Izquierdo et al., Citation2013), described in more detail in the section devoted to Measurements. Exclusion criteria included any type of diagnosed pathology or disease, or self-reported pain; having unusual bedtimes (between 3:00 a.m. and 6:00 p.m.) and/or extreme chronotype (score ≤20 or ≥80 in the Morningness-Eveningness Questionnaire-MEQ); engaging in professional bicycling regularly (more than once per week for a period longer than 3 h). High-level sportsmen in any other discipline were also automatically excluded from the study (Category 3 of the International Physical Activity Questionnaire—IPAQ). All subjects were informed in detail about the project and gave their written informed consent before the inclusion in the study. Finally, the study involved 48 healthy subjects (22 males and 26 females) aged between 19 and 29 years.
Randomization and blinding
Immediately after verifying that the participants met the criteria necessary to be included in the trial, the participants were randomly assigned in a counter-balanced fashion to either an EN intervention or the control group using a computer-generated random-sequence table with a two-balanced block design (GraphPad Software, Inc, San Diego, CA). The randomization list was generated and guarded by a member of the research team, who did not participate in the evaluation of subjects. The masking procedure was carried out by a blinded researcher. In addition, participants were strongly asked not to comment to other participants on the intervention received. This process results in two equally sized (n = 24) intervention groups: the neuromodulation group (EN) and the control group (CG).
Measurements
During the pre-training evaluation, the participant’s vestibular balance was measured with the Vertiguard System.
Participants were evaluated with the Vertiguard® device (Vesticure GmbH, Germany) while they performed the Standard Balance Deficit Test (SBDT), consisting of 14 different stance and gait conditions (Rossi-Izquierdo et al., Citation2013), which are shown in . This system was fixed on a belt at the center of body mass located around the waist and was composed of a battery driven main unit and four vibration stimulators at the front, back, right and left sides of the waist adjustable in every individual subject (Rossi-Izquierdo et al., Citation2013). This device recorded trunk sway in the pitch (anterior-posterior) and roll (lateral) planes. Body sway was measured, in terms of average of the absolute angular velocity (degrees/second), by gyroscopes at the waist while the participants were asked to carry out the SBDT. Despite only healthy participants are tested in the present study, it intends for the results to be extrapolated to rehabilitation scenarios. In this sense, the measurement of the absolute average angular sway velocity could be an advantage for the analysis of aggravating patients because they often show a slow high angle sway (Basta et al., Citation2013), but one or more momentary wide-amplitude sway excursions during the measurement period also could influence the median strongly if they occur with a high velocity (possible sway pattern in patients with vestibular disorders). The Vertiguard® device performs an automatic self-calibration before each measurement and then compensates the sensor drift continuously (Basta et al., Citation2013). Since human balance is affected and controlled by three systems (vision, vestibular and proprioception), the Vertiguard® software also provides an estimation of the degree of participation or contribution to the total balance for each of them. The SBDT score, or risk of falling indicator, provides a measurement of balance, calculated by the formula:
Table 1. Tasks of the standard balance deficit test (SBDT) (V: Visual, P: Proprioception, VB: Vestibular).
with p = pitch sway/normal value in %, r = roll sway/normal value in %, n = number of tasks in SBDT. This norm is based on 246 healthy volunteers (137 females, 109 males) (source: Vesticure GmbH, Pforzheim, Germany). Each one of the 14 tasks of the SBDT contributes a certain percentage of visual, proprioceptive and/or vestibular stimulation to the SBDT score. In , the participation of the three systems for each task of the test is indicated (Basta et al., Citation2005). Together with trunk sway, these degrees of participation were also considered in the balance assessment of participants.
Protocol and interventions
First, a pre-evaluation was performed by the application of the vestibular test. After this balance evaluation, the subject started to work out on a real ISB. The ISB used was a common gearless city bike with the distinctive feature of turning the front wheel in the opposite direction to the handlebars. For each participant, the height of the bike seat was set to the height of the participant’s iliac crests. Moreover, the distance between the front tip of the bike seat and the middle of handlebars was set to the distance between the fingertip of the middle finger and the elbow in the right upper limb of each participant. Adapting the protocol timing used in Hoedlmoser et al. (Citation2015), the maximum training time was 60 min, divided into four sessions each of 15 min of riding the ISB interleaved with periods of 15 min of VR (EN group) or slideshow video (control group) intervention between them (a maximum total time of 105 min for each participant). If the participant succeeded to ride the ISB before the end of the maximum training time, the experiment was stopped. The experimental process is depicted in . A participant was considered successful in riding the ISB if she or he rode it straight for a minimum of 10 m, determined by two red parallel lines painted on the ground, without putting feet on the ground. This achievement was defined as a successful motor adaptation of body balance and upper limb control.
The Endogenous Neuromodulation (EN) intervention or the control intervention was performed between the ISB training sessions in a separate room that was blinded to the other investigators in charge of the evaluations. Participants in the EN group performed a visually guided motor imagery neuromodulation: they were asked to imagine themselves as simultaneously performing the movements shown in a prerecorded first-person video of fluent ISB riding played in immersive virtual reality goggles. Immersive virtual reality has been evidenced as promoting transferable and generalized learning gains with respect to computer screen display (Pagé et al., Citation2019). The control group was asked to passively watch a video in virtual reality goggles showing steady landscapes.
Data analysis
All data analyses were performed using SPSS for Windows, version 21.0 (SPSS Inc, Chicago, IL, USA). The statistical analyses were conducted at a 95% confidence level; a p-value of less than .05 was considered statistically significant. We expressed our results as means and standard deviations (SD). All statistical tests were selected during the experimental design.
A multivariate one-way ANOVA was applied to test the differences in the descriptive and balance-related variables between groups. The difference in categorical variables, such as sex, was tested by the chi-squared test. To test the significance of the differences in adaptation results (adaptation rate, i.e. percentage of participants who successfully adapted the ISB, and time to adapt, i.e. effective training time until the 10-m test was passed) between groups, a univariate one-way ANCOVA test was applied, taken all descriptive and balance-related variables as covariates. Finally, a multivariate one-way ANOVA was also applied to test the differences in descriptive and balance-related variables between ISB riding success and sex groups. We also report effect size, as partial eta squared, and observed power for significant differences.
Results
Descriptive and balance-related variables
There were no statistically significant differences in age, body mass index, and gender between the control (CG) and experimental (EN) groups ().
Table 2. Descriptive and balance data of the two study groups. BMI: Body Mass Index (kg/m2); lat: Angular velocity in the lateral plane (degrees/sec); front: Angular velocity in the frontal plane (degrees/sec).
Among all the measures in all tasks and planes, the only significant difference found between groups among the balance-related variables was the average angular velocity in the lateral plane when the participants were standing on one leg on a foam support surface (“SOEOF lat,” , row 16). The analysis showed that the control group presented a higher average velocity than the experimental group in the balance task just mentioned (“SOEOF lat”).
Task achievement
Regarding the number of subjects that succeeded to achieve the adaptation to the ISB, there was no statistically significant difference between groups. Among those who did learn the ISB, the time to learn was significantly higher (around 14 min longer) in the EN group than in the control group (see ).
Table 3. Inverse steering bicycle (ISB) riding adaptation results for the two study groups. min: minutes.
We analyzed the differences between the participants who succeeded to adapt to the ISB riding and the ones who did not within each of the two groups separately. In the control group, the successful participants presented a significantly higher participation of the proprioceptive component in balance (36.00%±27.78 vs 9.38%±21.75; F(1,22) = 6.029, p = .024, η2p = .241, observed power 1-β = .644), as well as a significantly lower age (21.98 ± 1.17 vs 24.54 ± 3.17; F(1,22) = 4.748, p = .042, η2p = .200, observed power 1-β = .543) than the unsuccessful participants. However, in the EN group, no significant difference in the descriptive or balance-related variables was found between successful and unsuccessful participants. So, results in the control group suggest that the ISB adaptation success positively depends on the participation of the proprioceptive component in balance, while the success of the participants from the EN group does not seem rely on descriptive or balance-related variables. Therefore, we hypothesize that neuromodulation through the first-person ISB riding video reduces the role of the balance variables since successful and no successful participants belonging to the EN group present indiscriminate high or low participation of the proprioceptive component in balance.
The ISB motor adaptation results also showed that most participants who adapted to ride the ISB were males in both groups (10 males and 2 females in the CG group, 9 males and 3 females in the EN group). This aspect is discussed in more detail below.
Sex differences
The statistical analysis of the differences in all the participants of the study grouped by sex revealed several significant differences (see ).
Table 4. Statistically significant differences in descriptive and balance variables of the subjects in the study split by sex. BMI: Body Mass Index (kg/m2); lat: Angular velocity in the lateral plane (degrees/sec); front: Angular velocity in the frontal plane (degrees/sec).
The results in show a significantly higher Body Mass Index (BMI) in males. In addition, male participants also presented a significantly higher participation of the proprioceptive component in balance (43.75%) than females (16.88%). Finally, female participants showed a significantly higher average angular velocity in the lateral plane during walking 3 m either rotating the head or with eyes closed, and a significantly lower risk of falling.
Discussion
According to the results, the visually induced motor imagery neuromodulation, in the way that is applied in the present study, led to similar adaptation rates to those from the control intervention. This is in accordance with evidence stating that declarative knowledge about the task, as the visually induced neuromodulation intervention, did not predict its successful learning (van Abswoude et al., Citation2019). In addition, our results are also in agreement with the evidence that explicit instructions about the task to learn, as the visually induced neuromodulation also provides, could have a negative influence on learning depending on working memory factors (Buszard et al., Citation2017), which were not controlled in the study. Besides the type of model used for action observation, with a video where an experimented and skilled rider does the ISB task, might have also led to those results (Kawasaki et al., Citation2018).
In addition, our results also suggest that adapting to a complex motor task might imply cognitive fatigue, since the additional focused action observation and motor imagery yielded higher adaptation time than the control intervention. Prior studies have shown that following mental fatigue, subjects are still able to perform automated tasks although performance in complex tasks deteriorates (Pageaux et al., Citation2015). However, future studies should analyze the effect of cognitive fatigue during the adaptation to the type of complex motor task used in the present study.
Besides, the proportion of male participants that succeeded in the ISB riding adaptation was significantly higher than the proportion of female participants. The analysis of sex differences suggests that this might be due to the higher participation of the proprioceptive component in the balance of male participants in this study sample. Moreover, the same difference in proprioceptive participation was found between the successful and unsuccessful participants in the control group. In this sense, body self-awareness is likely a determinant factor to adapt the proposed ISB motor task, pointing to a higher impact of the proprioceptive-based balance on the motor adaptation than the visual- and vestibular components. Nevertheless, the proprioceptive component difference (and any other) between successful and unsuccessful participants was not observed in the EN group, suggesting that the visually guided motor imagery neuromodulation might make the success in ISB motor adaptation independent on the balance profiles of subjects. In this group, a plausible explanation about the ratio of successful males (9) to females (2) obtained could be based on the fact that men have a greater ability to perform motor imagery, as evidenced in Paris-Alemany et al., Citation2019.
Limitations
One of the main limitations of the study is the sample size. The comparison of the two intervention groups is statistically sufficient. However, the statistical power of the comparisons of subgroups within groups is reduced.
Besides, the physical and cognitive fatigue of the participants, which could have probably affected their performance and results according to the line of previously published studies, was not controlled in the present work. Fatigue should have been ideally assessed at every intervention time between the practice of the experimental session. However, on the one hand, cognitive fatigue is usually assessed by cognitive demanding tests that might have interfered with either the neuromodulation intervention or the slideshow video intervention. Physical fatigue, on the other hand, is usually measured by subjective questionnaires, which are gross-grained and consequently not suitable for our study. A finer alternative is the estimation of the physical fatigue from EMG, ECG, respiration and oxygen saturation sensors that, if available, would have required additional instrumentation and more time from the participants.
Another limitation to consider is the duration of our study. If we had made a longer follow up of the participants, we might have observed: 1) whether long-lasting changes in the procedural memory, which is involved in the process of skill adaptation, had taken place, and 2) once the ISB skill is learned, validate whether the regular cycling skill might get temporally inhibited (Doylon & Benali, Citation2005), and additional practice time had been needed to recover it, that is, how the two motor skills, both regular and adapted, might coexist and interfere. In this sense, we did not measure all the attempts and time until participants return to recover the ability to ride on the regular bicycle. Our hypothesis is that this issue could show relevant information about the individual process about learning and forgetting new complex motor tasks. Moreover, the visually guided motor imagery neuromodulation might also have an effect on this rollback process.
Finally, the 10-m test to determine when a participant succeeded in the motor adaptation task might not be completely accurate. It can be assured that the participants who passed the test actually adapted to the task (no false positive). However, other participants who partially completed it or even got close to passing it were considered as unsuccessful. We could have taken some measures from motion sensors during the test as assessment values. However, they would be affected by physical fatigue and muscle tone, whose development over the experiment is difficult to control. Therefore, we decided to apply a binary assessment test avoiding the false positive samples.
Interpretation of the results and future studies should keep in consideration these limitations.
Conclusion
The present study investigated the efficacy of a visually guided motor imagery neuromodulation intervention in the adaptation of a complex motor task like riding an inverse steering-wheel bicycle. As it was applied in the study, the visually guided neuromodulation resulted in a similar rate of adaptation success than the control intervention. Moreover, the visually guided motor imagery neuromodulation intervention produced a higher adaptation time, likely due to the induction of cognitive fatigue. Without any intervention (control), the results suggested that the motor adaptation success depended on the percentage of participation of proprioception in the balance of the participants, which was higher in male participants who, in turn, presented a higher rate of adaptation success. In this sense, these results point to a higher impact of body self-awareness than visual or vestibular information on the ISB motor adaptation task. Nevertheless, we postulate that further investigation would be needed to know a finer-grained contribution from each one of the three balance factors to motor adaptation of ISB. On the contrary, the visually induced motor imagery neuromodulation showed no dependence of the motor adaptation success on the prior balance profile of the participants. This points to a possible benefit of the visually guided neuromodulation for the motor adaptation of the subjects with a low participation of their proprioception component in balance.
In this sense, the results of the present study help for reaching prognostic criteria of the motor adaptation success when balance is involved, and also prescription criteria about the application of the proposed visually guided motor imagery neuromodulation based on the balance profile of subjects, thus improving and optimizing the motor adaptation training or rehabilitation of those subjects less susceptible of success a priori.
Ethical statement
The study was conducted at the Centro Superior de Estudios Universitarios La Salle (CSEULS). All experimental procedures were ratified by the La Salle University Ethics Committee in accordance with the Helsinki Declaration (CSEULS-PI-121/2016). The trial was registered with the United States National Institutes of Health Clinical Trials Registry, with the registration number NCT02999516.
Acknowledgments
We would like to thank all volunteers that have actively participated in the study.
Disclosure statement
No potential conflict of interest was reported by the author(s).
Additional information
Funding
References
- Basta, D., Rossi-Izquierdo, M., Soto-Varela, A., & Ernst, A. (2013). Mobile posturography: Posturographic analysis of daily-life mobility. Otology & Neurotology, 34(2), 288–297. https://doi.org/10.1097/MAO.0b013e318277a29b
- Basta, D., Todt, I., Scherer, H., Clarke, A., & Ernst, A. (2005). Postural control in otolith disorders. Human Movement Science, 24(2), 268–279. https://doi.org/10.1016/j.humov.2005.04.002
- Belda-Lois, J. M., Mena-Del Horno, S., Bermejo-Bosch, I., Moreno, J. C., Pons, J. L., Farina, D., & Rea, M. (2011). Rehabilitation of gait after stroke: A review towards a top-down approach. Journal of Neuroengineering and Rehabilitation, 8(1), 1–19. https://doi.org/10.1186/1743-0003-8-66
- Briem, V., Radeborg, K., Salo, I., & Bengtsson, H. (2004). Developmental aspects of children’s behavior and safety while cycling. Journal of Pediatric Psychology, 29(5), 369–377. https://doi.org/10.1093/jpepsy/jsh040
- Buszard, T., Farrow, D., Verswijveren, S. J. J. M., Reis, M., Williams, J., Polman, R., & Masters, R. S. W. (2017). Working memory capacity limits motor learning when implementing multiple instructions. Frontiers in Psychology, 8, 1350. https://doi.org/10.3389/fpsyg.2017.01350
- Cain, S. M., Ashton-Miller, J. A., Perkins, N. C., & Haddad, J. M. (2016). On the skill of balancing while riding a bicycle. PLoS ONE, 11(2), e0149340. https://doi.org/10.1371/journal.pone.0149340
- Chapin, H., Bagarinao, E., & Mackey, S. (2012). Real-time fMRI applied to pain management. Neuroscience Letters, 520(2), 174–181. https://doi.org/10.1016/j.neulet.2012.02.076
- Cullen, K. E. (2012). The vestibular system: Multimodal integration and encoding of self-motion for motor control. Trends in Neurosciences, 35(3), 185–196. https://doi.org/10.1016/j.tins.2011.12.001
- deCharms, R. C., Maeda, F., Glover, G. H., Ludlow, D., Pauly, J. M., Soneji, D., & Mackey, S. C. (2005). Control over brain activation and pain learned by using real-time functional MRI. Proceedings of the National Academy of Sciences USA, 102(51), 18626–18631. https://doi.org/10.1073/pnas.0505210102
- Dekker, M. K. J., Van den Berg, B. R., Denissen, A. D. J. M., Sitskoorn, M. M., & Van Boxtel, G. J. M. (2014). Feasibility of eyes open alpha power training for mental enhancement in elite gymnasts. Journal of Sports Sciences, 32(16), 1550–1560. https://doi.org/10.1080/02640414.2014.906044
- Del Castillo, M. D., Serrano, J. I., Lerma, S., Martínez, I., & Rocon, E. (2018). Evaluación Neurofisiológica del Entrenamiento de la Imaginación Motora con Realidad Virtual en Pacientes Pediátricos con Parálisis Cerebral. Revista Iberoamericana de Automática e Informática industrial, 15(2), 174–179. https://doi.org/10.4995/riai.2017.8819
- Doylon, J., & Benali, H. (2005). Reorganization and plasticity in the adult brain during learning of motor skills. Current Opinion in Neurobiology, 15(2), 161–167. https://doi.org/10.1016/j.conb.2005.03.004
- Ertl, M., Moser, M., Boegle, R., Conrad, J., Zu Eulenburg, P., & Dietrich, M. (2017). The cortical spatiotemporal correlate of otolith stimulation: Vestibular evoked potentials by body translations. NeuroImage, 155, 50–59. https://doi.org/10.1016/j.neuroimage.2017.02.044
- Gatti, R., Sarasso, E., Pelachin, M., Agosta, F., Filippi, M., & Tettamanti, A. (2019). Can action observation modulate balance performance in healthy subjects? Archives of Physiotherapy, 9(1), 1. https://doi.org/10.1186/s40945-018-0053-0
- Hardwick, R. M., Caspers, S., Eickhoff, S. B., & Swinnen, S. P. (2018). Neural correlates of action: Comparing meta-analyses of imagery, observation, and execution. Neuroscience & Biobehavioral Reviews, 94, 31–44. https://doi.org/10.1016/j.neubiorev.2018.08.003
- Hoedlmoser, K., Birklbauer, J., Schabus, M., Eibenberger, P., Rigler, S., & Mueller, E. (2015). The impact of diurnal sleep on the consolidation of a complex gross motor adaptation task. Journal of Sleep Research, 24(1), 100–109. https://doi.org/10.1111/jsr.12207
- Kavanagh, J. A., Issartel, J., & Moran, K. (2020). Quantifying cycling as a foundational movement skill in early childhood. Journal of Science & Medicine in Sport, 23(2), 171–175. https://doi.org/10.1016/j.jsams.2019.08.020
- Kawasaki, T., Tozawa, R., & Aramaki, H. (2018). Effectiveness of using an unskilled model in action observation combined with motor imagery training for early motor learning in elderly people: A preliminary study. Somatosensory & Motor Research, 35(3–4), 204–211. https://doi.org/10.1080/08990220.2018.1527760
- Lee, K. B., Lim, S. H., Kim, K. H., Kim, K. J., Kim, Y. R., Chang, W. N., Hwang, B. Y., Kim, Y. D., & Hwang, B. Y. (2015). Six-month functional recovery of stroke patients: A multi-time-point study. International Journal of Rehabilitation Research, 38(2), 173–180. https://doi.org/10.1097/MRR.0000000000000108
- Medendorp, W. E., & Selen, L. J. P. (2017). Vestibular contributions to high-level sensorimotor functions. Neuropsychologia, 105, 144–152. https://doi.org/10.1016/j.neuropsychologia.2017.02.004
- Nashner, L. (1997). Practical biomechanics and physiology of balance. In G. Jacobson, C. Newman, & J. Kartush (Eds.), Handbook of balance function testing (pp. 261–279). Singular Publishing Group.
- Ono, Y., Wada, K., Kurata, M., & Seki, N. (2018). Enhancement of motor-imagery ability via combined action observation and motor-imagery training with proprioceptive neurofeedback. Neuropsychologia, 114, 134–142. https://doi.org/10.1016/j.neuropsychologia.2018.04.016
- Orand, A., Ushiba, J., Tomita, Y., & Honda, S. (2012). The comparison of motor learning performance with and without feedback. Somatosensory & Motor Research, 29(3), 103–110. https://doi.org/10.3109/08990220.2012.687419
- Pageaux, B., Marcora, S. M., Rozand, V., & Lepers, R. (2015). Mental fatigue induced by prolonged self-regulation does not exacerbate central fatigue during subsequent whole-body endurance exercise. Frontiers in Human Neuroscience, 9, 67. https://doi.org/10.3389/fnhum.2015.00067
- Pagé, C., Bernier, P. M., & Trempe, M. (2019). Using video simulations and virtual reality to improve decision-making skills in basketball. Journal of Sports Sciences, 37(21), 2403–2410. https://doi.org/10.1080/02640414.2019.1638193
- Paris-Alemany, A., La Touche, R., Agudo-Carmona, D., Fernández-Carnero, J., Gadea-Mateos, L., Suso-Martí, L., & Cuenca-Martínez, F. (2019). Visual motor imagery predominance in professional Spanish dancers. Somatosensory & Motor Research, 36(3), 179–188. https://doi.org/10.1080/08990220.2019.1641480
- Payne, G. V., & Isaacs, L. D. (2020). Human motor development: A lifespan approach (10th ed.). Routledge, Taylor & Fraincis.
- Pfurtscheller, G., Müller-Putz, G. R., Scherer, R., & Neupeur, C. (2008). Rehabilitation with brain-computer interface systems. IEEE Computer, 41(10), 58–65. https://doi.org/10.1109/MC.2008.432
- Rossi-Izquierdo, M., Ernst, A., Soto-Varela, A., Santos-Pérez, S., Faraldo-García, A., Sesar-Ignacio, A., & Basta, D. (2013). Vibrotactile neurofeedback balance training in patients with Parkinson’s disease: Reducing the number of falls. Gait & Posture, 37(2), 195–200. https://doi.org/10.1016/j.gaitpost.2012.07.002
- Seidler, R. D., Bo, J., & Anguera, J. A. (2012). Neurocognitive contributions to motor skill learning: The role of working memory. Journal of Motor Behavior, 44(6), 445–453. https://doi.org/10.1080/00222895.2012.672348
- Seidler, R. D., & Noll, D. C. (2008). Neuroanatomical correlates of motor acquisition and motor transfer. Journal of Neurophysiology, 99(4), 1836–1845. https://doi.org/10.1152/jn.01187.2007
- Seidler, R. D., Noll, D. C., & Chintalapati, P. (2006). Bilateral basal ganglia activation associated with sensorimotor adaptation. Experimental Brain Research, 175(3), 544–555. https://doi.org/10.1007/s00221-006-0571-y
- Stöckel, T., & Fries, U. (2013). Motor adaptation in complex sports – the influence of visual context information on the adaptation of the three-point shot to altered task demands in expert basketball players. Journal of Sports Sciences, 31(7), 750–758. https://doi.org/10.1080/02640414.2012.750003
- Tan, A. L., Chiong, Y., Nadkarni, N., Cheng, J. Y. X., Chiu, M. T., & Wong, T. H. (2018). Predictors of change in functional outcome at six months and twelve months after severe injury: A retrospective cohort study. World Journal of Emergency Surgery, 13(1), 57. https://doi.org/10.1186/s13017-018-0217-y
- Tan, G., Thornby, J., Hammond, D. C., Strehl, U., Canady, B., Arnemann, K., & Kaiser, D. A. (2009). Meta-analysis of EEG biofeedback in treating epilepsy. Clinical EEG and Neuroscience, 40(3), 173–179. https://doi.org/10.1177/155005940904000310
- van Abswoude, F., van der Kamp, J., & Steenbergen, B. (2019). The roles of declarative knowledge and working memory in explicit motor learning and practice among children with low motor abilities. Motor Control, 16(1), 1–18. https://doi.org/10.1123/mc.2017-0060
- Vogt, S., & Thomaschke, R. (2007). From visuo-motor interactions to imitation learning: Behavioural and brain imaging studies. Journal of Sports Sciences, 25(5), 497–517. https://doi.org/10.1080/02640410600946779
- Willingham, D. B. (1998). A neuropsychological theory of motor skill learning. Psychological Review, 105(3), 558–584. https://doi.org/10.1037/0033-295X.105.3.558
- Zeuwts, L. H. R. H., Vansteenkiste, P., Deconinck, F. J. A., Cardon, G., & Lenoir, M. (2017). Hazard perception in young cyclists and adult cyclists. Accident; Analysis and Prevention, 105, 64–71. https://doi.org/10.1016/j.aap.2016.04.034