Abstract
Re-establishment of native aquatic macrophytes may be used to enhance habitat for fish and other organisms, with additional positive effects on water quality. Species selection and planting depth for re-vegetation efforts may be of great importance due to lake-specific ecological factors that may affect survival. The objective of this research was to test whether water depth or species selection contributed to the overall survival of macrophytes planted inside protective exclosures. We planted three species along a water depth gradient (0.30, 0.60, and 1.0 m) – Stuckenia pectinata, Vallisneria americana, and Potamogeton nodosus. Our results indicated that the depth in which species were initially planted rarely had an effect on survival of P. nodosus or V. americana (p < 0.05), but species did have an effect (p < 0.05). Stuckenia pectinata did not survive during the experiment.
Introduction
Aquatic macrophytes are important components of freshwater ecosystems and serve a number of purposes that mediate physical and biological processes (Savino and Stein Citation1982; Carpenter and Lodge Citation1986). Biologically, aquatic macrophytes provide habitat and food for invertebrates, all ages of fish, and other aquatic organisms and form the base of the food chain in many aquatic systems (Carpenter and Lodge Citation1986). Physical and physicochemical contributions by macrophytes include decreasing turbidity levels via removal of suspended particles and nutrients from the water column and stabilizing sediment (Madsen et al. Citation2001; Bouldin et al. Citation2004). Native macrophytes may increase dissolved oxygen levels during photosynthesis and cool water by shading of solar radiation via floating leaves (Honnell et al. Citation1993). Overall, macrophytes are important in structuring aquatic communities (Jeppesen et al. Citation1998) and provide essential fish habitat (Dibble et al. Citation1996).
Due to biogeographic and hydrologic limitations, natural establishment of plant communities in constructed reservoirs may be restricted (Doyle and Smart Citation1993; Smart et al. Citation1996; Smart et al. Citation2005). Therefore, native plant communities may need to be cultured to initiate colonization of some reservoirs (Smart et al. Citation1998). In areas where aquatic macrophytes are native but not currently present, restoration of these communities is potentially a tool that could be used by ecosystem managers (Doyle and Smart Citation1993; Smart et al. Citation1996; Smiley and Dibble Citation2006). Methods to establish and restore native aquatic macrophytes have been developed by Smart et al. (1996, 1998, 2005). Additionally, trials on establishment of aquatic plant communities have been performed in Lake Guntersville, Alabama (Doyle and Smart Citation1993; Doyle and Smart Citation1995) as well as Oklahoma (Dick et al. Citation2004a), and Texas (Dick et al. Citation2004b), although results and success are unclear.
Research has been conducted on the interactions between macrophytes, invertebrates, and fish (e.g., Theel and Dibble Citation2008). These studies have tested a variety of hypotheses regarding fish growth rate, density, and behavior, and invertebrate density, species richness, and diversity (Diehl and Kornijow Citation1998; Persson and Crowder Citation1998). The regulating mechanism varies somewhat within these studies, but in most cases a significant explanatory variable is macrophyte stem density. Studying effects of macrophyte density is important, especially in the context of invasive species, which can occur in densities far greater than native species. The results of the research have almost always found that intermediate densities of aquatic plants are preferred by fish because they provide the balance needed to mediate predator/prey interactions in the littoral zone. Likewise, comparisons of native macrophytes with intermediate stem densities to exotic invasive macrophytes with dense stem densities indicate that intermediate stem densities allow more efficient fish foraging, which may result in an increase in overall fitness (Dibble et al. Citation1996). Although some macrophyte species can cause significant problems, most native species provide benefits that far outweigh the consequences of their absence.
Prior to the early 1990s there had been little research regarding the active propagation of aquatic macrophytes as a management tool for ecosystem restoration. Regarding native aquatic macrophyte re-establishment efforts, the word restoration itself may be a misnomer. The National Research Council (Citation1992) defined restoration as ‘returning a system to a close approximation of its condition prior to disturbance’ and accomplishing restoration as ‘ensuring that ecosystem structure and function are recreated or repaired, and that natural dynamic ecosystem processes are operating effectively again.’ In the case of constructed reservoirs, this definition would imply that the system should return to its pre-human altered state which is unrealistic when dealing with reservoirs constructed for purposes, such as flood control. In practice, restoration may involve temporal, multi-phase goals from restoring desired species or species richness (providing community structure), to monitoring development of these communities, to finally verifying that the desired structure and function have been linked and established (Palmer et al. Citation1997).
The history of macrophyte restoration is relatively short, indicating the recent emergence of this ecological application and most studies thus far have described the re-growth or pioneer colonization of native aquatic plant communities as a result of increased light availability and water clarity conducive to plant growth following some environmental manipulation (e.g., Sondergaard et al. Citation2007). For the purposes of this research, the use and interchange of the terms restoration, re-establishment, or re-vegetation will refer to anthropogenically propagated specimens of native aquatic plants to restore or re-establish native community structure and function and increase fish habitat in an aquatic ecosystem.
The only experimental testing of a hypothesis regarding establishment of native submersed aquatic plants was performed in Lake Charlie Capps in west-central Mississippi (Smiley and Dibble Citation2006). The results indicated that it is feasible to establish plants in southern US reservoirs, even in systems suffering from cultural eutrophication and high turbidity (Smiley and Dibble Citation2006). However, the species tested differed in percentage of cover, stem density, and probability of extinction, which indicated that not all species may be successful in re-vegetation projects.
Some of the most recently published documents regarding macrophyte restoration are related to site selection and environmental factors needed for native aquatic plant re-establishment. This includes simulation models combining hydrological, geological, and ecological information to predict the success of planting efforts (Best et al. Citation2008; Grodowitz et al. Citation2009).
The first objective of this project was to identify and successfully cultivate submersed or floating leaved aquatic macrophytes in Little Bear Creek Reservoir (LBCR), Alabama. This objective consisted of two phases, the first of which was to identify species that would be feasible for the re-establishment effort. The first evaluation of species was conducted in 2007 (Cheshier et al. Citation2008). The second phase focused on identified successful species (Potamogeton nodosus, Vallisneria americana, and Stuckenia pectinata), and we tested the null hypothesis that survival would be different for the three species because they differentially tolerate environmental conditions in LBCR. We also tested the null hypothesis that survival of each species would be different when initially planted at different water depths because of potential environmental variations, such as temperature, light availability, and wave action. In addition, trials were performed measuring the survival of specimens planted without herbivore protection.
Materials and methods
Study area description
LBCR is located in Franklin County in Northwest Alabama. The reservoir was impounded in 1975 for flood control, has a total impounded surface area (at full pool) of approximately 650 ha, extends 13 km upstream from the dam, and has a fluctuating water level of approximately 3.6 m each year, with full pool occurring from mid-April until late October (Tennessee Valley Authority (TVA) 2009). After impoundment, LBCR reportedly contained submersed (e.g., Potamogeton spp.) and emergent (e.g., waterwillow [Justicia americana] plants (Phillip Cooper and Gary Don Fleming, Bear Creek Millennium Project, personal communication). However, in recent years, there have been no submersed aquatic plant species present except muskgrass (Chara spp.), a non-vascular macro-alga. Additionally, there are local reports of a reduction in emergent plant assemblages.
The remnant flooded timber that once served as habitat for many fish species is in decline and needs to be replaced with a self-renewing habitat for forage fishes and young-of-the-year bass (Cheshier et al. Citation2008). A beneficial replacement for the flooded timber would be a diverse community of native aquatic plants, such as American pondweed (P. nodosus) and water celery (V. americana) (Smart et al. Citation1996).
Study design
Three native submersed aquatic plant species (P. nodosus, V. americana [northern ecotype], and S. pectinata) were transplanted into LBCR, and survival was evaluated relative to species, water depth, and presence of herbivore protection. Methods for growing and planting generally followed the recommendations of Smart et al. (Citation2005). Stuckenia pectinata and V. americana were obtained from Kester's Wild Game Food Nurseries, Inc., Omro, WI.
In 2008, tubers or fragments of S. pectinata and V. americana were planted in 7.6 cm (diameter) peat pots and allowed to grow in outdoor aquatic mesocosm tanks for approximately 6 weeks. Osmocote fertilizer (19-12-6) was used in each pot to boost production and improve plant survival during initial growth periods. Two specimens of a given species were planted in each pot. Plants were transplanted (early July) when sufficient growth had occurred and the water level in LBCR was stable. Potamogeton nodosus (approximately 45 cm stem length) was collected locally in Alabama and directly transplanted with bare roots at the time of exclosure plantings.
Specimens were transplanted among three sites in LBCR. Two sites were located in Trace Branch, and one site was in Cooper's Branch. Plants were transplanted inside 1.0 m diameter exclosures made of PVC-coated wire mesh (2.5 cm). Stuckenia pectinata and V. americana were left in the biodegradable pots, and the pots were placed in small excavated holes in the sediment. Four pots of the same species were planted inside an exclosure, and each exclosure contained only one species. Potamogeton nodosus rhizomes were planted directly into the sediment. Approximately 15 stems of P. nodosus were planted in each exclosure. Each planting site contained nine exclosures of each species, with three replicate exclosures at each of three contour intervals (0.3, 0.6, and 1.0 m). Three additional ‘patches’ of each species at each location were planted without exclosures at a water depth of 60 cm. Three exclosures per site in which no macrophytes were planted were used as a reference (one exclosure at each depth). Each exclosure was evaluated individually, and the data were analyzed based on species and water depth. Water level did not reach full pool but was relatively stable throughout the study period. Species and depth data from different sites were analyzed together, and site was not considered as an experimental variable in statistical tests.
Statistical analysis
Exclosures were initially evaluated based on two factors – (1) presence/absence of macrophytes planted in each location (exclosure or patch) to assess plant survival, and (2) visual estimation of percentage of cover inside each exclosure to assess growth. However, visual estimations of percentage of cover were confounded by differences in water clarity. In some cases, the presence of plants was only detectable by reaching into the exclosure to feel for them. In addition, differences in the morphologies of the different species did not allow a comparison of percentage of cover among species. Thus, the analyses were only of the survival of the plant species, measured from the presence or absence during the sampling periods of 2008 and 2009.
The presence/absence data were grouped by sampling period (date) and analyzed using a generalized linear model in SAS software v. 9.2 and SAS Enterprise Guide 4.2 (SAS Institute Citation2008). The presence/absence data have a binomial distribution and do not fit assumptions of normality for parametric statistical analysis. Therefore the generalized linear model analysis procedure (Proc Genmod) was set to analyze a binomial distribution using a link (logit) function. Hypothesis testing for significant differences was conducted with type III tests of fixed effects, and if differences were found, pairwise comparisons to detect means separation of all treatment class levels were performed using a least square means (lsmeans) analysis. All tests were performed with a significance level of 0.05.
Results
The propagules of all species planted outside of protective exclosures were absent 2 days after planting, which indicated intense herbivory. Therefore, no analysis relative to herbivore protection was possible.
Of the three species planted, P. nodosus had a significantly greater survival each sampling period (p < 0.05; ). Vallisneria americana survival in both Trace Branch sites was minimal. In Cooper's Branch, V. americana grew and expanded on the substrate but did not appear to substantially grow toward the surface. In September, after an initial decline during August, V. americana began re-growing in some exclosures. This was likely the result of cooling water temperature. Approximately 63% of the exclosures planted with V. americana had surviving plants in September 2008. This was significantly greater than S. pectinata (p < 0.001) but less than P. nodosus (p < 0.001).
Figure 1. Percentage of exclosures containing living propagules of macrophytes (by species) planted in LBCR during 2008 and 2009. Different capital letters represent significant differences in percentage survival on that sampling date.
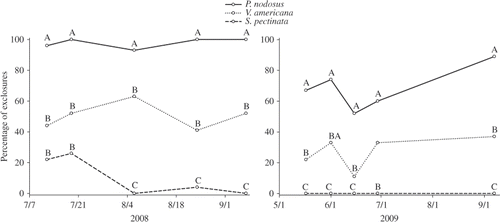
Initially, 20% of the S. pectinata propagules survived. However, they never expanded beyond the initial propagules inside exclosures and were absent in most exclosures after only a few weeks. In September 2008, no living plants were detected inside any exclosures, and no S. pectinata were detected in 2009. Therefore, S. pectinata was excluded from subsequent analyses.
During 2009, P. nodosus had a significantly greater survival (p < 0.05) than other species during all sampling periods except for V. americana on 29 June (p = 0.059; ). All other pairwise comparisons differed significantly (p < 0.05). Vallisneria americana was present during every sampling period but did not expand beyond exclosures.
Water depth at planting did not result in any significant differences in survival of P. nodosus at any of the three planting depths at any sampling period in 2008 (p < 0.05; ). In 2009, planting depth comparisons did not indicate any significant differences in survival of P. nodosus at the 17 May, 1 June, or 7 September sampling period (p < 0.05). However, there was a significant difference in survival between exclosures planted at 0.60 m and 1.0 m at the 15 June (p = 0.012) and 29 June (p = 0.012) sampling period. This may be attributable to a short rise in water level that possibly inundated the exclosures planted in 1.0 m water, leaving them exposed to herbivory.
Figure 2. Percentage of exclosures containing living propagules of macrophytes (by depth) planted in LBCR during 2008 and 2009. Upper graphs = P. nodosus, lower graphs = V. americana. Different capital letters represent significant differences in percentage survival on that sampling date. Asterisk (*) in upper left graph indicates an overlap in survival percentages of 0.3 m and 1.0 m depths.
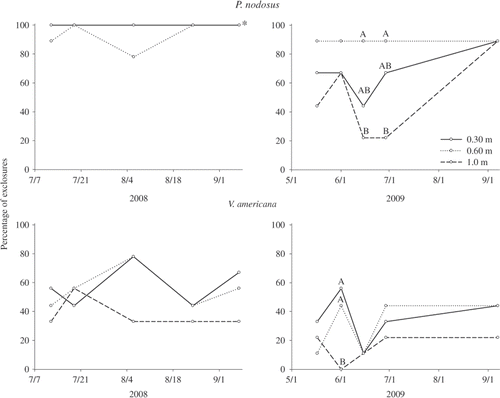
Planting depth comparisons during 2008 did not indicate any significant differences in survival rate of V. americana at any of the three planting depths (p < 0.05; ). During 2009, planting depth pairwise comparisons indicated a significant difference between survival in exclosures planted at 1.0 m and 0.60 m (p < 0.001) and 1.0 m and 0.30 m (p < 0.001) but did not indicate a difference between exclosures planted at 0.60 m and 0.30 m (p = 0.638) at the 1 June sampling period. All other sampling periods indicated no significant differences in survival of V. americana (p < 0.05).
Discussion
Re-establishment of native aquatic plants is a potential technique to restore aquatic habitats in the southeastern US (Smiley and Dibble Citation2006). Aquatic plant community restoration efforts have been attempted in Lake Guntersville, Alabama (Doyle and Smart Citation1993; Doyle and Smart Citation1995) as well as in Oklahoma (Dick et al. Citation2004a), Texas (Dick et al. Citation2004b), and Mississippi (Smiley and Dibble Citation2006). Establishment of aquatic plant communities can have positive effects on water quality and provide essential habitat and sanctuary for fish fauna (Dibble et al. Citation1996). Successfully cultivating submersed aquatic vegetation in LBCR was problematic with difficulties possibly attributable to fluctuating water level, high water temperature, and herbivory. In 2008, water level never reached full pool which complicated restoration efforts. In 2009, water level was dropped early, further complicating experimental hypothesis testing.
Results from trials performed in LBCR in 2007 indicated that P. nodosus was the best candidate for restoration when compared to other species tested (Cheshier et al. Citation2008). In 2008, S. pectinata and V. americana were planted along with P. nodosus to assess their survival potential for another year in case environmental conditions had changed from past trials. These species all produce some form of subterranean overwintering structures, such as tubers or winter-buds. Tubers and winter-buds provide new plants with the necessary carbohydrates needed to initiate growth in subsequent growing seasons (Cronk and Fennessy Citation2001). They may also serve as a mechanism to aid in plant survival during adverse environmental conditions, such as drawdown. Given the nature of water level fluctuations in LBCR, focused re-vegetation efforts are only feasible for species adapted to survival when environmental conditions do not meet the necessary requirements for year-round growth.
Potamogeton nodosus was the most successful species planted in 2008. Stuckenia pectinata never appeared to adjust to lake conditions and disappeared soon after planting. This result is consistent with findings by Doyle and Smart (Citation1995) regarding S. pectinata. Water depth may play a key role in the success of submersed macrophytes (Rea et al. Citation1998). To evaluate this in LBCR planting was stratified at initially controlled water levels (depths). Water temperature in shallow areas can become a significant factor impacting plant growth (Barko et al. Citation1982; Pilon and Santamaria Citation2002). The hypothesis was that water depth would facilitate temperature differences and thus cause differential survival and growth of plant species. However, results indicated that water depths of 0.30, 0.60, and 1.0 m rarely had any significant effect on survival percentage of P. nodosus or V. americana.
In spring 2008, the water level appeared to be rising in concordance with the operating guide for LBCR; however, structural complications with LBCR dam required TVA to drop the water level to implement repairs. Therefore, in 2008 the water level still did not reach full pool but was relatively stable throughout the study period. The unpredictable nature of water level from year to year must be considered when planning additional re-vegetation efforts in LBCR or when considering re-vegetation in other reservoirs.
After 2 years of trials, it appeared that efforts should focus on P. nodosus as the primary species to maximize re-vegetation success. Other studies also have indicated this species as a good candidate for re-establishment (Doyle and Smart Citation1995; Smart et al. Citation2005; Smiley and Dibble Citation2006). Potamogeton nodosus has shown promising results in its ability to grow and expand within exclosures. However, plant growth outside of the exclosures was rare. This was likely due to some form of herbivory from fauna that could not penetrate the exclosures but could consume or otherwise decimate plant material that grew beyond the exclosure. This has been observed in past re-vegetation trials (Doyle and Smart Citation1995; Dick et al. Citation2004a). Aquatic turtles and common carp were observed in LBCR, and on occasion were actually found inside the plant exclosures.
Future work should focus on mitigating for negative abiotic (e.g., water level fluctuations) and biotic (e.g., herbivory) factors, possibly by manipulating patch sizes to increase total plant biomass. Future studies might also focus on establishing emergent species, such as American waterwillow. Quantitative studies regarding its establishment have been reported recently (Strakosh et al. Citation2005; Collingsworth et al. Citation2009). Strakosh et al. (Citation2009) also suggested that waterwillow can have a significant positive impact on largemouth bass density. Using this species for re-establishment has been suggested (Smart et al. Citation2005) but has not been attempted in LBCR.
Acknowledgements
This research was supported by the Alabama Department of Conservation and Natural Resources and the Little Bear Creek Millennium Group. We thank Phillip Cooper, Gary Don Fleming, Cindy Fleming, Joshua Cheshier, Matthew Spickard, Thad Huenemann, Rafael Gonzalez, Ryan Wersal, Bear Creek Development Authority, and all the volunteers who helped build exclosures and propagate plants.
References
- Barko , JW , Hardin , DG and Matthews , MS . 1982 . Growth and morphology of submersed freshwater macrophytes in relation to light and temperature . Canadian Journal of Botany , 60 : 877 – 887 .
- Best , EPH , Teeter , AM , Landwehr , KJ , James , WF and Nair , SK . 2008 . Restoration options for potential persistence of submersed aquatic vegetation: combining ecological, hydrodynamic, and sediment transport modeling . Freshwater Biology , 53 : 814 – 826 .
- Bouldin , JL , Farris , JL , Moore , MT and Cooper , CM . 2004 . Vegetative and structural characteristics of agricultural drainages in the Mississippi Delta landscapes . Environmental Pollution , 132 : 403 – 411 .
- Carpenter , SR and Lodge , DM . 1986 . Effects of submersed macrophytes on ecosystem processes . Aquatic Botany , 26 : 341 – 370 .
- Cheshier , J , Madsen , JD and Wersal , RM . 2008 . Restoring native aquatic vegetation in Little Bear Creek Reservoir for 2007 , Mississippi : Mississippi State University. . GeoResources Institute Report 4004. p. 8
- Collingsworth , PD , Oster , RA , Hickey , CW , Heidinger , RC and Kohler , CC . 2009 . Factors affecting water willow establishment in a large reservoir . Lake and Reservoir Management , 25 : 200 – 207 .
- Cronk , JK and Fennessey , MS . 2001 . Wetland plants: biology and ecology , Boca Raton (FL) : Lewis Publishers .
- Dibble , ED and Harrel , SL . 1997 . Largemouth bass diets in two aquatic plant communities . Journal of Aquatic Plant Management , 35 : 74 – 78 .
- Dibble , ED , Killgore , KJ and Harrel , SL . 1996 . Assessment of fish-plant interactions . Multidimensional approaches to reservoir fisheries management. American Fisheries Society Symposium , 16 : 357 – 372 .
- Dick , GO , Smart , RM and Gilliland , ER . 2004a . Aquatic vegetation restoration in Arcadia Lake, Oklahoma: a case study , ERDC/EL TR-04-7. Lewisville Aquatic Ecosystem Research Facility. Lewisville (TX) : U. . S. Army Engineer Research and Development Center
- Dick , GO , Smart , RM and Smith , JK . 2004b . Aquatic vegetation restoration in Cooper Lake, Texas: a case study , ERDC/EL TR-04-5. Lewisville Aquatic Ecosystem Research Facility. Lewisville (TX) : U. . S. Army Engineer Research and Development Center
- Diehl , S and Kornijow , R . 1998 . “ Influence of submerged macrophytes on trophic interactions among fish and macroinvertebrates ” . In The structuring role of submerged macrophytes in lakes , Edited by: Jeppesen , E , Sondergaard , Ma , Sondergaard , Mo and Christoffersen , K . 24 – 46 . New York : Springer Verlag .
- Doyle , RD and Smart , RM . 1993 . Potential use of native aquatic plants for long-term control of problem aquatic plants in Guntersville Reservoir, Alabama , Report 1, Establishing native plants. Technical Report A-93-6. Vicksburg (MS) : U. . S. Army Corps of Engineers Waterways Experiment Station
- Doyle , RD and Smart , RM . 1995 . Potential use of native aquatic plants for long-term control of problem aquatic plants in Guntersville Reservoir, Alabama , Report 2, Competitive interactions between beneficial and nuisance species. Technical report A-93-2. Vicksburg (MS) : U. . S. Army Engineer Waterways Experiment Station
- Grodowitz , MJ , Smart , M , Dick , GO , Stokes , JA and Snow , J . 2009 . Development of a multi-attribute utility analysis model for selecting aquatic plant restoration sites in reservoirs , APCRP Technical Notes Collection. ERDC/TN APCRP-EA-21. Vicksburg (MS) : U. . S. Army Engineer Research and Development Center
- Honnell , DR , Madsen , JD and Smart , RM . 1993 . Effects of selected exotic and native aquatic plant communities on water temperature and dissolved oxygen , Vicksburg (MS) : U. . S. Army Engineer Research and Development Center. A-93-2. p. 7
- Jeppesen E, Sondergaard Ma, Sondergaard Mo, Christoffersen K, editors. 1998. The structuring role of submerged macrophytes in lakes. New York: Springer-Verlag. p. 423
- Madsen , JD , Chambers , PA , James , WF , Koch , EW and Westlake , DF . 2001 . The interaction between water movement, sediment dynamics and submersed macrophytes . Hydrobiologia , 444 : 71 – 84 .
- National Research Council . 1992 . Restoration of aquatic ecosystems: science, technology, and the public , Washington, DC : National Academy Press .
- Palmer , MA , Ambrose , RF and Poff , NL . 1997 . Ecological theory and community restoration ecology . Restoration Ecology , 5 : 291 – 300 .
- Persson , L and Crowder , LB . 1998 . “ Fish-habitat interactions mediated via ontogenetic niche shifts ” . In The structuring role of submerged macrophytes in lakes , Edited by: Jeppesen , E , Sondergaard , Ma , Sondergaard , Mo and Christoffersen , K . 3 – 23 . New York : Springer Verlag .
- Pilon , J and Santamaria , L . 2002 . Clonal variation in the thermal response of the submerged aquatic macrophyte Potamogeton pectinatus . Journal of Ecology , 90 : 141 – 152 .
- Rea , TE , Karapatakis , DJ , Guy , KK , Pinder , JE III and Mackey , HE Jr . 1998 . The relative effects of water depth, fetch and other physical factors on the development of macrophytes in a small southeastern US pond . Aquatic Botany , 61 : 289 – 299 .
- Richardson , WB , Zigler , SJ and Dewey , MR . 1998 . Bioenergetic relations in submerged aquatic vegetation: an experimental test of prey use by juvenile bluegills . Ecology of Freshwater Fish , 7 : 1 – 12 .
- SAS Institute . 2008 . The SAS system for Windows, version 9.2 and Enterprise Guide version 4.2 , Cary (NC) : SAS Institute .
- Savino , JF and Stein , RA . 1982 . Predator-prey interaction between largemouth bass and bluegills as influenced by simulated, submersed vegetation . Transactions of the American Fisheries Society , 111 : 255 – 266 .
- Smart , RM , Dick , GO and Doyle , RD . 1998 . Techniques for establishing native aquatic plants . Journal of Aquatic Plant Management , 36 : 44 – 49 .
- Smart RM, Dick GO, Snow JR. 2005. Update to the propagation and establishment of aquatic plants handbook. U.S. Army Engineer Research and Development Center, Lewisville Aquatic Ecosystem Research Facility. Report ERDC/EL TR-05-4. p. 44
- Smart , RM , Doyle , RD , Madsen , JD and Dick , GO . 1996 . Establishing native submersed aquatic plant communities for fish habitat . American Fisheries Society Symposium , 16 : 347 – 356 .
- Smiley , PC Jr and Dibble , ED . 2006 . Evaluating the feasibility of planting aquatic plants in shallow lakes in the Mississippi Delta . Journal of Aquatic Plant Management , 44 : 73 – 80 .
- Sondergaard , M , Jeppesen , E , Lauridsen , TL , Skov , C , van Nes , EH , Roijackers , R , Lammens , E and Portielje , R . 2007 . Lake restoration: successes, failures and long-term effects . Journal of Applied Ecology , 44 : 1095 – 1105 .
- Strakosh , TR , Eitzmann , JL , Gido , KB and Guy , CS . 2005 . The response of water willow Justicia americana to different water inundation and desiccation regimes . North American Journal of Fisheries Management , 25 : 1476 – 1485 .
- Strakosh , TR , Gido , KB and Guy , CS . 2009 . Effects of American water willow establishment on density, growth, diet, and condition of age-0 largemouth bass in Kansas reservoirs . Transactions of the American Fisheries Society , 138 : 269 – 279 .
- Theel , HJ and Dibble , ED . 2008 . An experimental simulation of an exotic aquatic macrophyte invasion and its influence on foraging behavior of bluegill . Journal of Freshwater Ecology , 23 : 79 – 89 .
- Tennessee Valley Authority [TVA]. 2009. Little Bear Creek Operating Guide [cited December 2009]. Available from: http://www.tva.com/river/lakeinfo/op_guides/littlebear.htm