Abstract
A series of studies have been implemented on Banks Lake, Washington, in recent years with the overall objective to maximize the fishery by determining the factors that limit the recruitment of various sport fish. When sampling commenced, it became apparent that the lake whitefish (Coregonus clupeaformis) was a major component of the system. The purpose of this study was to estimate the species composition, distribution, and abundance of the limnetic fish community of Banks Lake using hydroacoustics and gill nets. Surveys were conducted in May and July to test for differences in seasonal distribution and efficacy of the survey gear to establish a time period for conducting annual surveys for trend monitoring. Whitefish dominated the limnetic gill-net species composition by weight (95% both months) and number (83% July and 88% May). In May, relatively few fish were distributed deeper than 8 m at the south end of the reservoir, and low densities were common for the deepest strata (16–24 m). In July, most fish were in the deepest strata available, 16–24 m throughout most of the reservoir. Reservoir-wide mean fish density was not significantly different between May and July, but acoustic target distribution varied by depth and location within and between surveys. In July, fish were concentrated near the North Dam where cooler water was being pumped in from Lake Roosevelt and in Devil's Lake embayment where deeper bottom depths offered a cool water refuge. These areas represent cool water refuge for salmonids and will be key spatial considerations for future fish management actions.
Introduction
Whitefishes (Coregonus spp.) are an important global fisheries resource in north temperate portions of Europe, Asia, and North America (Baldwin et al. Citation1979; Mookerji et al. Citation1998). Extensive research efforts have been employed throughout Europe, Canada, and the Great Lakes region of the United States (Walker et al. Citation1993; Bégout Anras et al. Citation1999; Raitaniemi et al. Citation1999). However, in the western United States, whitefish are an underutilized fisheries resource that receives very little management or research effort. In general, other salmonids (trout, salmon, and char) and spiny ray fishes (Micropterous spp., Perca flavescens, Sander vitreus) are more desirable to western anglers and management agencies. Hence, little is known about the life history, movement patterns, seasonal distribution, and population abundance and structure of lake whitefish in the western United States.
Little was known about the lake whitefish (Coregonus clupeaformis) in Banks Lake, Washington; however, they were abundant in the gill net catches of a Washington Department of Fish and Wildlife (WDFW) survey in 2000 (Woller et al. Citation2004). Concurrent angler surveys suggested that this abundant lake whitefish population was not being utilized by recreational fishermen (Lewis et al. Citation2002; Polacek et al. Citation2003a; Woller et al. Citation2004). Banks Lake is an engineered storage reservoir occupying upper Grand Coulee in north central Washington. The reservoir is 43 km long, contains 1.6 billion m3 of water, and has a wetted surface area of 10,881 ha. At an elevation of 479 m above the sea level (full pool), the average depth is 14 m with a maximum depth of 25 m throughout most of the reservoir, and 52 m in Devil's Lake embayment (a 99 ha embayment, ). Water is pumped 85 m vertically from the forebay of Grand Coulee Dam (Lake Roosevelt) to Banks Lake near the North Dam. The historic fishery was comprised primarily of kokanee (Oncorhynchus nerka) and yellow perch (P. flavescens; Duff Citation1973); however, with the introduction of walleye (S. vitreus) in the early 1960s and smallmouth bass (Micropterus dolomieu) in the early 1980s, the fishery has since shifted primarily to bass, walleye, and rainbow trout (Oncorhynchus mykiss; Lewis et al. Citation2002; Polacek et al. Citation2003b).
Figure 1. Map of Banks Lake, Washington showing locations of hydroacoustic transects (1–18) in May and July of 2002. Stars indicate limnological sites Lim1 to Lim4. Area of detail shows Devil's Lake embayment where Lim2 and hydroacoustic transect 7 were located.
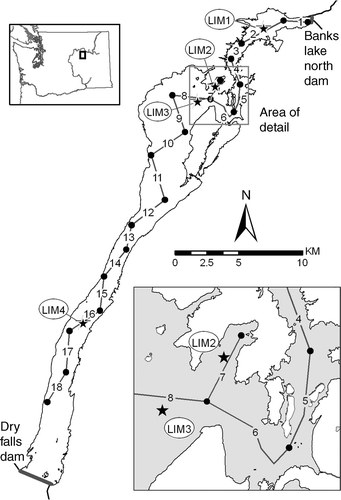
Hydroacoustics uses sound impulses transmitted through water to determine fish size, depth, and population density (Traynor and Ehrenberg Citation1979; Brandt Citation1996; Cryer Citation1996). Abundance and distribution can then be determined by expanding results from individual transects to the entire system (Thorne Citation1979; Levy et al. Citation1991; Beauchamp et al. Citation1997). Hydroacoustics is most effective for suspended limnetic species when surveyed with a vertically oriented transducer. However, recent advances in technology using a horizontally oriented transducer allow for fish detection within 1.5 m of the surface (Yule Citation2000; Baldwin and McLellan Citation2008).
Hydroacoustics cannot determine species composition, so complementary surveys must be used. Common methods for verifying acoustic targets include trawling, purse seining, and gill netting (Parkinson et al. Citation1994; Bean et al. Citation1996; Yule Citation2000; Baldwin and McLellan Citation2008). In Europe, midwater trawls and gill nets are commonly used to verify acoustic target species composition for Coregonus spp. in both large and small aquatic systems (Jurvelius et al. Citation1988; Mehner and Schultz Citation2002; Schmidt et al. Citation2005; Mehner Citation2006).
Our goal was to evaluate the fish assemblage in the offshore area of Banks Lake and to determine the vertical and horizontal distribution and abundance of lake whitefish using hydroacoustics and gill nets. Additionally, we sought to evaluate seasonal shifts in lake whitefish distribution and relate changes in distribution to temperature, basin morphology, and water management. Our literature search did not find any studies where hydroacoustics and gill-net surveys were used to evaluate lake whitefish in the western United States. Therefore, our study also represents a test of the legitimacy and efficacy of hydroacoustic and gill-net methods for evaluating a lake whitefish population in a large reservoir.
Materials and methods
Banks Lake was surveyed in May and July 2002, with an HTI model 241 echosounder with two 200 kHz transducers – a 15° split-beam transducer in vertical orientation and a 6° × 10° elliptical split-beam transducer in horizontal orientation. Data were logged directly into a computer and unprocessed echoes were backed up using digital audiotapes. A pulse repetition rate of three pings per second was multiplexed between the transducers at a pulse width of 1.25 ms and a 10 kHz pulse width chirp. The horizontal transducer was offset by 7° and sampled fish targets from 1.5 to 8 m below the surface. Data within 12 m of the horizontal transducer and 8 m of the vertical transducer were excluded from analysis due to the narrow beam width reducing the ability to detect fish and potential boat avoidance by fish in the near field (Mous and Kemper Citation1996; Yule Citation2000).
Transects were conducted in an elongated zigzag pattern across the limnetic zone of Banks Lake by navigating from predetermined global positioning system (GPS) waypoints (). On 27 and 28 May, we surveyed four transects between 2313 and 0024 h before high winds kept us from finishing the survey. On 30 and 31 May, we surveyed another 12 transects between 1026 and 0337 h. On 29 and 30 July, we surveyed 18 transects between 1018 and 0529 h. Transects averaged 2.7 km and total survey distance was 44.6 km in May and 48.2 km in July. Boat speed averaged 8.0 and 7.6 km/hour in May and July, respectively. A GPS logged the latitude and longitude into the data files, and transect distances were calculated using Terrain Navigator (Maptech Citation1999).
A series of acoustic echoes was counted as a fish if tracked for at least three consecutive pings (within 0.3 m/ping) at a maximum velocity of 5 ms/ping; target strengths between −55 and −27.7 dB were used for the vertical transducer and −27.7 and −50 dB on the horizontal transducer using an auto‐tracking feature in the post‐processing software Echoscape 2.11 (Hydroacoustic Technology Incorporated Citation2002). For each transect, individual tracked fish were later manually verified within the post‐processing software, and targets smaller than −45 dB were excluded from further analysis due to the lack of reliable target verification of fish in that size category.
Density (fish/104 m3) was calculated for each transducer and each transect separately, and then transect densities were averaged together for a reservoir‐wide estimate of fish density. Raw fish counts were adjusted to the effective beam width (EBW) within each depth strata by the equation:
Limnetic gill‐net surveys were used to provide species verification, depth distributions, and length‐frequencies of acoustic targets larger than 100 mm. The night of the survey and for two nights following the survey, 7–14 vertical gill nets and one floating and one sinking horizontal gill net were set in randomly selected locations in the limnetic zone. Only one type of gill net (vertical or horizontal) was set in each location. Nets were set in the evening and retrieved in the morning, temporally integrating across the nighttime and both crepuscular periods. Terrain Navigator (Maptech Citation1999) software was used to spatially segregate the limnetic sampling sites in a grid pattern (∼500 m diameter). Netting locations were then randomly selected using Statview (SAS Institute Citation1999). In total, 48 of 252 (19%) limnetic sites were sampled with a combination of vertical or horizontal gill nets during each survey.
Vertical and horizontal gill nets were used with mesh sizes from 13 to 102 mm in 13 mm increments. Horizontal nets were set on the surface and the bottom to provide better coverage in areas where vertical nets were not as effective. Additional vertical gill nets were set in the Devil's Lake embayment in July to provide supplemental information due to observations of an unusually high density of acoustic targets in this area. The results of this supplemental netting effort were not included with the random – reservoir‐wide netting effort when calculating catch per unit effort (CPUE), distribution, and species composition due to the potential bias of this supplemental effort. All fish captured in gill nets were measured for total length to the nearest millimeter and weighed to the nearest gram. Otoliths were removed from a subsample of the fish and aged. For a more detailed description of netting and aging efforts, see Baldwin and Polacek (Citation2007).
Mean fish density for acoustic targets between −45 and −27.7 dB was multiplied by lake volume to estimate abundance. We applied the target strength frequency from the vertical transducer to the horizontal acoustic targets because fish target echoes in horizontal aspect do not relate to fish length as they do in vertical aspect (Love Citation1971, Citation1977; Kubecka Citation1994; Yule Citation2000). We did not include abundance estimates for acoustic targets corresponding to fish lengths less than 150 mm in this study because the gill nets were not effective at capturing the smaller fish.
Abiotic conditions were measured on 22 May and 23 July 2002 at three limnetic locations in the main basin and on 23 July 2002 in the Devil's Lake embayment (Lim1 to Lim4; ). Temperature, dissolved oxygen, conductivity, turbidity, and pH were recorded in 3 m increments, beginning at 0.5 m with a Hydrolab Minisonde 4a.
Results
Hydroacoustics sampled 8325 fish targets during the two surveys were combined with 4114 fish detections in May and 4211 in July. The reservoir‐wide fish density was not significantly different between May (mean 6.8 ± SE 1.3 fish/104 m3)] and July (8.3 ± 1.7 fish/104 m3) (ANOVA; df = 1; F‐ratio = 1.008; p = 0.323); however, acoustic target distribution varied by depth and location within and between surveys. In May, fish density was significantly higher in the upper 8 m of the water column (10.0 ± 1.7 fish/104 m3) than from 8 m to the reservoir bottom (3.7 ± 1.0 fish/104 m3)(ANOVA; df = 1, F‐ratio = 10.13, p = 0.003; ). Conversely, in July, fish density was significantly less (1.7 ± 0.8 fish/104 m3) in the upper 8 m of the water column than from 8 m to the reservoir bottom (15.0 ± 3.6 fish/104 m3; ANOVA; df = 1, F‐ratio = 14.5, p = 0.001). Finally, the average density in the upper 8 m of the water column was significantly higher in May (10.0 ± 1.7 fish/104 m3) compared to July (1.7 ± 0.8 fish/104 m3) (ANOVA; df = 1, F = 20.03, p < 0.001). Conversely, the average density was higher in the deeper depth strata in July (15.0 ± 3.6 fish/104 m3) compared to May (3.7 ± 1.0 fish/104 m3; ANOVA; df = 1, F = 10.50, p = 0.003).
Figure 2. Density of target‐tracked fish for 18 hydroacoustic transects in May and July 2002 on Banks Lake, Washington. Transects 3 and 4 were not analyzed in May due to shallow depths for the vertical transducer and excessive noise for the horizontal transducer.
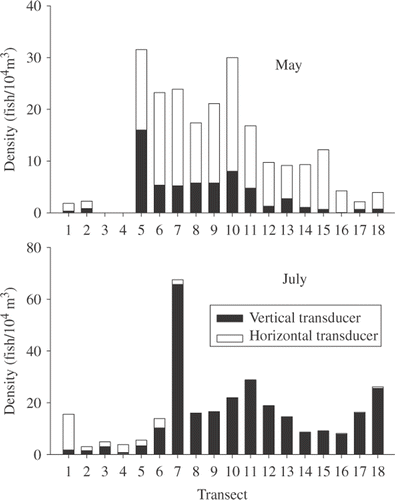
In May, fish densities were lowest near the north and south ends of the reservoir with the highest densities occurring in the middle transects (). In July, fish were more uniformly distributed throughout the reservoir, with the highest density occurring in the Devil's Lake embayment. In July, target density was threefold higher in transect 7 (Devil's Lake embayment) than it had been in May. Likewise, at each end of the reservoir there were substantial increases in fish target density in July compared to May. Transect 1 had a 13‐fold increase, and transects 17 and 18 had seven and sixfold increases, respectively.
In May, limnetic nets caught 127 fish, and lake whitefish dominated the species composition by weight (95%) and number (88%; ). Whitefish were captured at all depths, with the highest frequency (32%) occurring between 16 and 20 m (). Thirty‐five percent were captured between 0 and 8 m, 20% between 8 and 16 m, and 45% below 16 m.
Figure 3. The vertical depth distribution of lake whitefish captured in vertical and horizontal gill nets in the offshore zone of Banks Lake, Washington in May and July of 2002 (a) and in Devil's Lake embayment in July of 2002 (b).
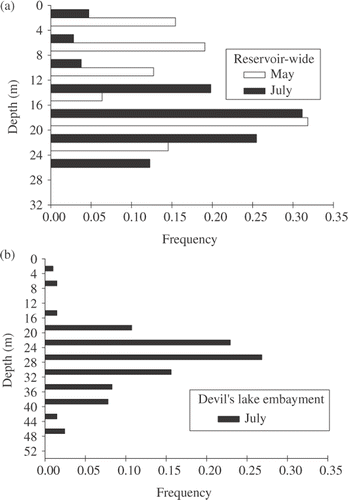
Table 1. Species composition by number and weight and the minimum and maximum lengths of fish captured in offshore gill nets on Banks Lake, Washington in May and July 2002.
In July, limnetic nets caught 127 fish and lake whitefish dominated the species composition by weight (95%) and number (83%; ). Whitefish were captured at all depths with the highest frequency occurring between 16 and 20 m (31%). Only 8% were captured between 0 and 8 m, 24% between 8 and 16 m, and 69% below 16 m (). Lake whitefish vertical distribution (from the gill nets) was significantly deeper in July than in May (Kolmogorov–Smirnov two sample test, p < 0.001).
Two nights of vertical gill‐net effort captured 219 fish in the Devil's Lake embayment and whitefish comprised 94% of the total catch (by number). Whitefish were distributed between 4 and 48 m, with the highest density at 22–24 m and 65% captured between 16 and 24 m ().
Reservoir‐wide hydroacoustic abundance estimates for all fish targets were 1.01 × 106 (±1.85 × 105 SE) in May and 1.23 × 106 (±2.57 × 105) in July (). Lake whitefish specific abundance estimates were 8.93 × 105 (±1.63 × 105) in May and 1.02 × 106 (±2.13 × 105). The target strength frequency distribution showed a single mode for all targets between −45 and −27.7 dB, which shifted slightly between May and July (). Therefore, we did not try to generate age‐ or size‐specific abundance estimates. Likewise, the length at age analysis revealed a very fast seasonal growth rate for age‐1 whitefish and substantial overlap between age classes of lake whitefish after age‐2 ().
Figure 4. Frequency of acoustic target strengths observed during mobile hydroacoustic surveys of Banks Lake, Washington in May and July 2002.
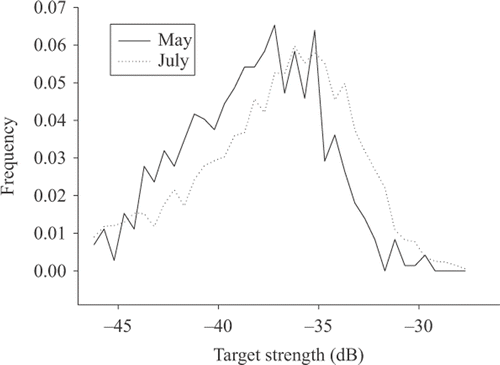
Figure 5. Length‐at‐age as determined by otolith age analysis for lake whitefish in Banks Lake, Washington in 2002.
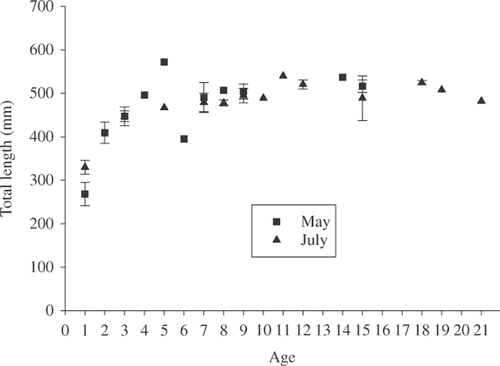
Table 2. Abundance estimates of all acoustic targets (−45 to −27.7 dB), the proportion of lake whitefish in the gill‐net catch, and the abundance of lake whitefish (age‐1 and older) in Banks Lake, Washington in May and July 2002.
In May, vertical temperature at the limnology stations gradually increased from 9°C near the bottom to 13°C near the surface (Baldwin and Polacek Citation2007). Temperature was more variable in July, with temperature as high as 23°C at 0.5 m and approximately 14°C near the bottom. Dissolved oxygen was greater than 10 mg/L throughout the water column in May, with no stratification. Dissolved oxygen conditions were also more variable in July, dropping to 6.2 mg/L near the bottom. In the Devil's Lake embayment in July (Lim2), water temperatures ranged from 24.5°C at the surface to 4.8°C at the bottom and a thermocline at 20 m. Dissolved oxygen reached a high of 10.7 mg/L at 6 m and low of 0.6 mg/L near the bottom.
Discussion
Whitefish dominated the catch in the limnetic zone of Banks Lake, had relatively consistent abundance between May and July, and showed a vertical and horizontal shift in distribution between months. Whitefish were commonly captured throughout the water column in May when the temperature was favorable regardless of depth. In July, when temperature commonly exceeded 16°C in the upper 8 m, whitefish were rarely captured (8%) in the upper portion of the water column and hydroacoustic density was significantly lower in the near surface strata. In July, fish were concentrating near the North Dam where cool water was being pumped in from Lake Roosevelt and in Devil's Lake embayment where a deeper bottom allowed for thermal stratification. These areas offered cool water refuge for salmonids. Both hydroacoustic and gill‐net surveys showed an apparent mass migration of whitefish into the Devil's Lake embayment. To our knowledge, a phenomenon like this has not been reported for lake whitefish in the literature.
The random net site selection resulted in only one gill‐net set at the north end of the reservoir in July and it caught a rainbow trout near the surface. Since hatchery rainbow trout are released in this area in May and/or June each year, it is possible that the high abundance of acoustic targets near the surface was due to recently released rainbow trout. The water temperature in this area increased from 16°C in July to 19°C in August, thereby eliminating this area as a potential thermal refuge for kokanee, but it was still well within the thermal tolerance of rainbow trout and lake whitefish.
In Devil's Lake embayment, the gill nets with 89 and 102 mm mesh sizes were so satiated with large whitefish that the net efficiency may have been compromised. If other species were present at lower densities, they may not have had a chance to encounter the net before it ‘filled‐up’ with whitefish. We do not know if the catch rate of whitefish would have been higher based on the attraction of struggling fish or lower based on avoidance. Therefore, we did not use that data in our reservoir‐wide species composition expansion of the acoustic abundance estimate. Regardless, it was apparent that there was an incredible biomass of lake whitefish in the Devil's Lake embayment. Given that lake whitefish were virtually non‐existent in the creel surveys, this represents an underutilized resource that has potential for recreational or commercial harvest. Development of a recreational fishery for lake whitefish could represent a unique opportunity in eastern Washington. Removal of lake whitefish through harvest would likely benefit the recruitment and growth of other fishes such as rainbow trout and kokanee. Diet studies have shown that lake whitefish feed extensively on zooplankton and invertebrates and could therefore compete with rainbow trout or kokanee (Polacek et al. Citation2003a, 2003b).
We assumed equal probability of gill‐net capture between species; however, this assumption could have overestimated abundance if a species was more vulnerable to the gill nets. The gill nets only captured fish greater than 100 mm, and larger fish have greater capture probabilities in gill nets (Hamley Citation1975; Rudstam et al. Citation1984; Henderson and Wong Citation1991). We applied the species composition from all fish captured in the gill nets to all acoustic targets greater than −45.0 dB (∼100 mm). If species composition of the smaller fish was different from that of the larger fish, then our acoustic estimates would be biased for the smaller size classes. This was the primary reason we did not generate species‐specific estimates for acoustic targets smaller than −45.0 dB.
We could not determine the volume of water in the limnetic zone independently from the littoral zone. Mean density was extrapolated to reservoir‐wide volume; therefore, we assumed that fish density in the littoral zone was equal to the limnetic zone for the species composition observed in limnetic gill nets. We recognize that species composition was much different in the littoral zone and included many more species than we observed in the limnetic zone (Polacek et al. Citation2003a, 2003b). Additionally, if nearshore densities of whitefish were higher than offshore densities, then we underestimated reservoir‐wide abundance of whitefish. There was a threefold decrease in CPUE of whitefish in nearshore gill nets between May and July (Polacek et al. Citation2003a). Gill nets are not the best capture method for determining density because they are dependent on fish activity rates, but this could help explain the increase in density we observed in the offshore areas in July. The effect of this potential bias was further minimized during the July survey due to the high epilimnetic temperature that was well above thermal optimum for whitefish.
The horizontal transducer could not differentiate target strength, so we could not determine the density of specific size classes for near‐surface targets. We assumed that the size distribution of fish was the same from 1.5 to 8 m and from 8 m to the bottom. Similar mean lengths for each species and depth interval from the gill nets verified this assumption.
This study evaluated the limnetic fish community of Banks Lake as one portion of a larger project with the goal of evaluating the Banks Lake fishery and determining limiting factors for various sportfish, such as kokanee. We have identified two potential limiting factors for stocked kokanee and rainbow trout: (1) competition with whitefish and (2) limited refuge from warm water in late summer. Initial studies have shown relatively high abundance of zooplankton forage and the presence of large‐bodied Daphnia spp., indicating that Banks Lake is not likely food limited (Polacek et al. Citation2003a, 2003b). Future efforts may need to determine the carrying capacity of Banks Lake for kokanee based on the available late‐summer cool water habitat. Managers may be overestimating the ability of Banks Lake to yield kokanee if they base stocking rates on total hectares and not usable volume during the most limiting time period. The stress of sharing habitat with a high density whitefish population may reduce kokanee survival even more. Little is known about competition for space in lentic ecosystems and knowing the tolerance limits for the density of competing species may be critical for the success of kokanee in Banks Lake or other similar situations. Substantial reservoir‐wide reductions in whitefish abundance could be necessary to allow success for the hatchery kokanee and rainbow trout programs.
Finally, these surveys indicate that July provided a more precise estimate of limnetic fish abundance than May and that future monitoring should continue during this period when fish are distributed deeper thereby making them more susceptible to detection by the vertically oriented transducer. Future efforts should also evaluate the distribution and life history of juvenile whitefish to better understand recruitment and spatial and temporal overlap that could be influencing competition with more desirable sport fishes.
Acknowledgements
We thank the Bonneville Power Administration (BPA) for funding the project, and the Northwest Power Planning Council, Columbia Basin Fish and Wildlife Authority, Independent Scientific Review Panel, and R. Morinaka (BPA) for evaluation and input during development of the research plan. We thank D. Burgess (WDFW) and the Moses Lake research team for assistance during fish sampling. Additional fieldwork or planning assistance was contributed by K. Knuttgen, J. Korth, J. McLellan, A. Smith, J. Kiesel, and H. Woller. We thank John Sneva and Lucinda Morrow (WDFW) for aging the otoliths and Arleta Agun for making the map. Finally, we thank the reviewers and editor of the Journal of Freshwater Ecology for their contributions to the final manuscript.
References
- Baldwin , CM and McLellan , JG . 2008 . Use of gill nets for target verification of a hydroacoustic fisheries survey and comparison with kokanee spawner escapement estimates from a tributary trap . North American Journal of Fisheries Management , 28 : 1744 – 1757 .
- Baldwin , CM and Polacek , MC . 2007 . Hydroacoustic and gill net assessment; Banks Lake, Washington, 2001–2002 annual report , Portland (OR) : BPA. . Project No. 200102800. BPA Report DOE/BP‐00005860‐3: 34 p
- Baldwin , NS , Saalfeld , RW , Ross , MS and Buettner , HJ . 1979. Commercial fish production in the Great Lakes, 1867–1977. Great Lakes Fishery Commission, Technical Report No. 3
- Bean , CW , Winfield , IJ and Fletcher , JM . 1996 . “ Stock assessment of the artic charr (Salvelinus alpinus) population in Loch Ness, UK ” . In Stock assessment in inland fisheries , Edited by: Cowx , IG . 206 – 223 . London (England) : Fishing News Books .
- Beauchamp , DA , Luecke , C , Wurtsbaugh , WA , Gross , HG , Budy , PE , Spaulding , S , Dillenger , R and Gubala , CP . 1997 . Hydroacoustic assessment of abundance and diel distribution of sockeye salmon and kokanee in the Sawtooth Valley Lakes, Idaho . North American Journal of Fisheries Management , 17 : 253 – 267 .
- Bégout Anras , ML , Cooley , PM , Bodaly , RA , Anras , L and Fudge , RJP . 1999 . Movement and habitat use by lake whitefish during spawning in a boreal lake: integrating acoustic telemetry and geographic information systems . Transactions of the American Fisheries Society , 128 : 939 – 952 .
- Brandt , SB . 1996 . “ Acoustic assessment of fish abundance and distribution ” . In Fisheries techniques , Edited by: Murphy , BR and Willis , DW . 385 – 432 . Bethesda (MA) : American Fisheries Society .
- Cryer , M . 1996 . “ A hydroacoustic assessment of rainbow trout (Oncorhynchus mykiss) population in a deep oligotrophic lake ” . In Stock assessment in inland fisheries , Edited by: Cowx , IG . 196 – 205 . London (England) : Fishing News Books .
- Duff , RL . 1973 . 1971–1972 Banks Lake creel census , Olympia (WA) : Washington Department of Game. . Internal report. Region 2. 39 p
- Hamley , JM . 1975 . Review of gillnet selectivity . Journal of the Fisheries Research Board of Canada , 32 : 1943 – 1969 .
- Henderson , BA and Wong , JL . 1991 . A method for estimating gillnet selectivity of walleye (Stizostedion vitreum vitreum) in multimesh multifilament gill nets in Lake Erie and its application . Canadian Journal of Fisheries and Aquatic Sciences , 48 : 2420 – 2428 .
- Hydroacoustic Technology Incorporated. 2002. Echoscape version 2.10, Seattle, Washington
- Jurvelius , J , Lindem , T and Heikkinen , T . 1988 . The size of a vendance, Coregonus albula L., stock in a deep lake basin monitored by hydroacoustic methods . The Fisheries Society of the British Isles , 32 : 679 – 687 .
- Kubecka , J . 1994 . Simple model on the relationship between fish acoustic target strengths and aspect for high‐frequency sonar in shallow waters . Journal of Applied Ichthyology , 10 : 75 – 81 .
- Levy , DA , Ransom , B and Burczynski , J . 1991 . Hydroacoustic estimation of sockeye salmon abundance and distribution in the Strait of Georgia, 1986 , Vancouver (BC) : Pacific Salmon Commission. . Technical Report No. 2
- Lewis , M , Polacek , M , Knuttgen , K , Luvrak , J and Baldwin , C . 2002 . Ford Hatchery; Annual Report 2001–2002 , Portland (OR) : Bonneville Power Administration. . Project No. 2001‐02900. Report DOE/BP‐00005850‐1: 35 p
- Love , RH . 1971 . Dorsal‐aspect target strength of an individual fish . Journal of the Acoustical Society of America , 49 : 816 – 823 .
- Love , RH . 1977 . Target strength of an individual fish at any aspect . Journal of the Acoustical Society of America , 62 : 1397 – 1403 .
- Maptech 1999. Terrain Navigator, version 4.05. Andover (MA)
- Mehner , T . 2006 . Prediction of hydroacoustic target strength of vendace (Coregonus albula) from concurrent trawl catches . Fisheries Research , 79 : 162 – 169 .
- Mehner , T and Schulz , M . 2002 . Monthly variability of hydroacoustic fish stock estimates in a deep lake and its correlation to gillnet catches . Journal of Fish Biology , 61 : 1109 – 1121 .
- Mookerji , N , Heller , C , Meng , HJ , Bürgi , HR and Müller , R . 1998 . Diel and seasonal patterns of food intake and prey selection by Coregonus sp. in re-oligotrophicated Lake Lucerne, Switzerland . Journal of Fish Biology , 52 : 443 – 457 .
- Mous , PJ and Kemper , J . 1996 . “ Application of hydroacoustic sampling technique in a large wind-exposed shallow lake ” . In Stock assessment in inland fisheries , Edited by: Cowx , IG . 179 – 195 . London (England) : Fishing News Books .
- Parkinson , EA , Ransom , BE and Rudstam , LG . 1994 . Comparison of acoustic and trawl methods for estimating density and age composition of kokanee . Transactions of the American Fisheries Society , 123 : 841 – 854 .
- Polacek , M , Baldwin , C , Knuttgen , K and Woller , H . 2003a . Banks lake fishery evaluation project , Portland (OR) : Bonneville Power Administration Report. Annual report for 2001–2002 . Project No. 2001‐02800. DOE/BP‐00005860‐1: 102 p
- Polacek , M , Knuttgen , K and Shipley , R . 2003b . Banks lake fishery evaluation project annual report: fiscal year 2002 (September 1, 2002 to August 31, 2003) , Portland (OR) : Bonneville Power Administration. . Project No. 2001‐028‐00: 72 p
- Raitaniemi , J , Malinen , T , Nyberg , K and Rask , M . 1999 . The growth of whitefish in relation to water quality and fish species composition . Journal of Fish Biology , 54 : 741 – 756 .
- Rudstam , LG , Magnuson , JJ and Tonn , WM . 1984 . Size selectivity of passive fishing gear: a correction for encounter probability applied to gill nets . Canadian Journal of Fisheries and Aquatic Sciences , 41 : 1252 – 1255 .
- Institute. , SAS . 1999. Statview version 5.0.1. Cary (NC)
- Schmidt , MB , Gassner , H and Meyer , EI . 2005 . Distribution and biomass of an under fished vendace, Coregonus albula, population in a mesotrophic German reservoir . Fisheries Management and Ecology , 12 : 169 – 175 .
- Systat Software Incorporated. 2004. Version 11.00.01. Richmond (CA)
- Thorne , RE . 1979 . Hydroacoustic assessments of adult sockeye salmon (Oncorhynchus nerka) in Lake Washington 1972–1975 . Journal of the Fisheries Research Board of Canada , 36 : 1145 – 1149 .
- Traynor , JJ and Ehrenberg , JE . 1979 . Evaluation of the dual beam acoustic fish target strength measurement method . Journal of Fisheries Research Board of Canada , 36 : 1065 – 1071 .
- Walker , SH , Prout , MW , Taylor , WW and Winterstein , SR . 1993 . Population dynamics and management of lake whitefish stocks in Grand Traverse Bay, Lake Michigan . North American Journal of Fisheries Management , 13 : 73 – 85 .
- Woller , H , Baldwin , C , Polacek , M and Knuttgen , K . 2004 . Banks Lake Fishery Survey, September 2000 , Olympia (WA) : Washington State Department of Fish and Wildlife. . Report No. FPT 04‐06
- Yule , DL . 2000 . Comparison of horizontal acoustic and purse‐seine estimates of salmonid densities and sizes in eleven Wyoming waters . North American Journal of Fisheries Management , 20 : 759 – 775 .