Abstract
We tested whether leaf breakdown of American beech (Fagus grandifolia) and sugar maple (Acer saccharum) was sensitive to slight differences in episodic acidification. We measured leaf breakdown in four headwater streams within the Adirondack Mountains, New York, USA, in summer and autumn 2005. We also investigated whether episodic acidification influenced the breakdown of beech and maple in leaf litter mixtures in autumn. Breakdown of maple was faster than that of beech for both summer and autumn, and species mixtures did not affect the breakdown rates of maple or beech. Breakdown rates of maple but not beech were highest in the circumneutral stream, but only in summer. Although microbial respiration rate for maple was greatest in the circumneutral stream in autumn, both maple and beech breakdown in autumn were greatest in one of the episodically acidified streams. Higher discharge and a greater proportion of riffle areas in this stream probably led to greater physical fragmentation rates in autumn when discharge was higher but not during summer. Due to this discrepancy between summer and autumn, we suggest that leaf breakdown should be used in combination with other functional metrics (e.g., microbial respiration) to assess the response of stream ecosystems to anthropogenic disturbances.
Introduction
Organic matter derived from surrounding terrestrial vegetation is the base of food webs for small streams in forested catchments (Fisher and Likens Citation1973; Cummins Citation1974) and is processed through the combined actions of microbes and macroinvertebrate shredders. Therefore, leaf litter processing (i.e., leaf breakdown) integrates the actions of biological communities over time and can be used as an indicator of stream ecosystem health (Gessner et al. Citation1999; Gessner and Chauvet Citation2002; Dangles et al. Citation2004; Young et al. Citation2008). Leaf breakdown has been shown to respond to several anthropogenic disturbances, including land-use change (e.g., Sponseller and Benfield Citation2001; Hagen et al. Citation2006; Paul et al. Citation2006), excess nutrients (e.g., Meyer and Johnson Citation1983; Suberkropp and Chauvet Citation1995; Rosemond et al. Citation2002; Gulis and Suberkropp Citation2003), organic pollution (Pascoal et al. 2001, 2003), and toxic chemicals (e.g., Niyogi et al. Citation2001; Chaffin et al. Citation2005).
Leaf breakdown has also been shown to decrease in response to acidification of stream ecosystems caused by the anthropogenic deposition of nitric and sulfuric acids (e.g., Burton et al. Citation1985; Mulholland et al. Citation1987; Griffith and Perry Citation1994; Dangles and Chauvet Citation2003). This decrease in breakdown has been linked to reduced activity of microbes (Chamier 1986; Griffith and Perry Citation1994; Griffith et al. 1995), macroinvertebrate shredders (Griffith and Perry Citation1993; Dangles and Guérold 1998, 2001; Pretty et al. Citation2005), or both (Allard and Moreau Citation1986; Mulholland et al. Citation1987; Dangles et al. Citation2004). Dangles et al. (Citation2004) tested whether breakdown of leaf litter could be used to functionally assess the effects of stream acidification. They measured breakdown of Fagus sylvatica in several streams of the Vosges Mountains in northeastern France that differed in catchment geology. They found that breakdown of F. sylvatica was faster in circumneutral streams than in acidified streams, which they attributed to declines in microbial activity and an acid-sensitive leaf shredder, Gammarus fossarum. They concluded that leaf breakdown could be developed into a biomonitoring tool to functionally assess stream ecosystems but suggested further investigation was needed to determine the validity of this approach for other systems. Other studies have correlated leaf breakdown rates to stream pH in the eastern United States (Burton et al. Citation1985; Mulholland et al. Citation1987; Griffith and Perry Citation1993, 1994; Griffith et al. 1995), but it is not clear whether leaf breakdown is sensitive to slight differences in acidification among streams in this region.
Most studies linking stream pH to leaf breakdown have separately measured species breakdown rates even though natural leaf packs in streams usually consist of more than one species. Gartner and Cardon (Citation2004) suggested that litter mixtures could enhance or inhibit decomposition of the component species in terrestrial ecosystems by altering decomposer communities through changes in habitat complexity or by transferring nutrients or secondary chemicals between leaves of different species. Attempts have been made in stream ecosystems to determine whether the effect of multiple species is non-additive (i.e. where mixtures of different leaf species facilitate or inhibit breakdown of one or more species in the mixture). Some studies have shown that breakdown rates in mixtures are non-additive (McArthur et al. Citation1994; Swan and Palmer Citation2004; Kominoski et al. Citation2007), while others have shown limited or no evidence of non-additive effects (Leff and McArthur Citation1989; Lecerf et al. Citation2007; Taylor et al. Citation2007). It is not known, however, whether disturbances like acidic deposition augment or reduce non-additive effects.
In the Adirondack Mountain region of New York State, US regulations mandated by the Clean Air Act Amendments of 1990 have led to reductions in sulfur and nitrogen emissions responsible for acidic deposition (Driscoll et al. Citation2001). Acid neutralizing capacity and pH of surface waters in this region have increased slightly since these laws were enacted (Warby et al. Citation2005). However, in a survey of 189 small streams in the western Adirondack Mountain region, Lawrence et al. (Citation2009) found that 30% remain affected by episodic acidification. This type of chemical disturbance could lead to changes in leaf litter breakdown.
In this study, we investigated whether leaf breakdown could be used to functionally assess slight differences in episodic acidification of headwater stream ecosystems. We compared leaf breakdown rates among four low-order streams with similar underlying geology that were located in neighboring catchments of the Adirondack Mountain region. We also examined whether non-additive effects of two common tree species, sugar maple (Acer saccharum) and American beech (Fagus grandifolia), were influenced by episodic acidification. We expected that leaf breakdown would be sensitive to slight differences in episodic acidification. We also expected that non-additive effects of beech or maple in mixed-species packs would be reduced by episodic acidification due to a decrease in microbial and macroinvertebrate shredder activity.
Materials and methods
Site description
We selected four unnamed streams in the Moose River drainage basin in Herkimer County, New York, which hereafter will be referred to as streams S5, S6, S8, and S10. Hydrographs of small streams in this region are typically characterized by peaks in discharge during snowmelt in spring and during storms in summer and autumn. Stream pH and alkalinity generally decrease when discharge is high. Our study streams were first- or second-order streams, located in neighboring catchments (). Riffle areas in the streams consisted mainly of cobbles and pebbles, and runs and pools were composed mainly of sand with some pebbles. Due to a steeper stream gradient, riffles composed a greater proportion of stream area in S5 than in the other streams, which were dominated by runs and pools. All streams were shaded during the growing season by a dense canopy of red maple (Acer rubrum), yellow birch (Betula allegheniensis), and American beech along with some scattered sugar maple.
Table 1. Water physicochemical characteristics of the four study streams during summer and autumn 2005.
Stream chemistry
Water samples were collected daily by automated samplers (ISCO, Inc., Lincoln, Nebraska, USA) from 28 June to 2 August 2005 and from 28 August 2005 to 14 September, 2005. Grab samples were collected three times in early summer and four times in autumn 2005. We measured pH using an Accumet pH meter with a glass electrode, and alkalinity was determined by titration with 0.02 N hydrochloric acid and a bromcresol green-methyl red indicator. Samples were analyzed for ,
, Ca+2, and Mg+2 using ion chromatography (anions Model DX500, cations – Model DX100, DIONEX Corporation, Sunnyvale, California, USA). Soluble reactive phosphorus (SRP) was determined using the stannous chloride method where molybdenum blue intensity was measured at 690 nm.
Leaf breakdown and macroinvertebrate sampling
Freshly abscised leaves of sugar maple and American beech were collected from a site near our study streams and air-dried to constant weight. We used these leaves to construct leaf packs to measure leaf breakdown in summer and again in autumn. Leaf breakdown was determined in summer because many natural leaf packs remained in our study streams in late spring and early summer. However, we realize summer leaf packs may have served as resource patches for macroinvertebrate shredders that could artificially inflate leaf breakdown rates (Webster and Waide Citation1982; Benfield and Webster Citation1985; Benfield et al. Citation1991; Schofield et al. Citation2001).
We constructed single-species leaf packs in summer 2005 by tying 3 g of maple or beech leaves together with fishing line. On 21 June 2005, we anchored 15 packs of each species to bricks and placed the bricks at random locations on the bottom of each stream. Five additional packs of each species were immediately returned to the laboratory to estimate handling loss. We retrieved five packs per species from each stream 14, 27, and 42 days after initial placement. In autumn 2005, we constructed three types of leaf packs – mixed-species, single-species maple, and single-species beech. Single-species packs consisted of either 3 g of maple or beech, whereas mixed-species packs contained an equal proportion of maple and beech (1.5 g each). We placed the leaves into large mesh (∼0.4 cm2) bags that were fastened with cable ties to 20 bricks per stream so that each brick consisted of one leaf pack of each type (three packs total). Fifteen bricks were randomly placed on the bottom of each stream on 14 September, and the other five bricks were immediately returned to the laboratory to estimate handling loss. We retrieved five bricks from each stream 14, 28, and 49 days after initial placement.
In summer 2005, leaf packs were returned to the laboratory, washed over a 250 mm2 sieve, dried at 60°C, weighed, and ashed at 550°C to determine ash-free dry mass (AFDM). Macroinvertebrates were identified to the lowest practical taxonomic level and categorized according to functional feeding groups (Merrit and Cummins Citation1996). Autumn leaf packs were processed in a similar manner except sieved material was preserved in 5% formalin prior to macroinvertebrate identification and quantification. In addition, one leaf from single-species packs and one leaf per species from mixed-species packs were removed prior to washing. These individual leaves were used to estimate microbial respiration.
Microbial respiration
We estimated aerobic respiration of microbes associated with leaves by measuring consumption of dissolved oxygen (DO) according to Carlisle and Clements (2005). The day after each sampling date, a single leaf was removed from each single-species leaf pack, and one leaf of each species was removed from each mixed-species leaf pack. These leaves were rinsed gently with water from their corresponding stream and then were placed into 300 mL BOD bottles filled with stream water. Three additional BOD bottles per site without leaves were used as controls to determine initial dissolved oxygen concentration and account for DO consumption by microbes within the water column. Bottles were placed on an orbital shaker and incubated for 48 h in the dark at ambient stream temperature (i.e., 13°C on day 14, 11°C on day 28, and 7°C on day 49). After incubation, leaves were removed from the bottles, and DO concentration was measured with a meter (TSI Incorporated, Shoreview, Minnesota, USA). Leaves were dried, weighed, and ashed to calculate AFDM. Respiration was calculated as the net change in DO per g AFDM per day.
Data analysis
We used an exponential decay model to determine breakdown rates (k), where k was the negative slope of the natural log of percentage of AFDM loss over time (Benfield Citation1996). We conducted all statistical analyses using Statistical Analysis System (version 9.1, SAS Institute, Cary, North Carolina). We used an analysis of covariance (ANCOVA) with a dummy variable to make comparisons in breakdown rates between species, between single- and mixed-species packs, and among streams (Neatrour et al. Citation2004). To make pairwise comparisons in breakdown rates, we controlled the false discovery rate (i.e., the fraction of rejected null hypotheses) to reduce inflation of the Type 1 error rate resulting from multiple pairwise comparisons (Bejamini and Hochberg 1995). This technique is less conservative than traditional multiple test procedures (e.g., sequential Bonferroni) which control the familywise error rate to limit the chance of making any Type I errors (Garcia Citation2004; Verhoeven et al. Citation2005).
We tested for differences in log-transformed shredder density and the density of individual shredder taxa by performing two-way analysis of variance (ANOVA) with stream and species (summer) or stream and pack type (autumn) as main effects and date as a blocking factor. We also tested for differences in autumn respiration rates by running a two-way ANOVA with stream and pack type as main effects and date as a blocking factor. Posthoc comparisons were done using Tukey's test.
Results
Stream chemistry
Median pH was similar among streams (); however, pH of S6 was never <6.0, whereas pH of streams 5, 8, and 10 were all <5.1 at some point during the study when precipitation was high (). Stream pH also was higher in summer than in autumn. During two summer storms (27–28 July and 31 August) when pH was measured daily, pH decreased from 0.8 to 1.4 units but rebounded rapidly after the storms. Alkalinity tended to be higher in S6 relative to the other streams and decreased during the two summer storms (data not shown). and
concentrations were similar among streams, while Ca+2 tended to be greater for streams 6 and 8, and Mg+2 was highest for S6. Soluble reactive phosphate was low for all streams (<5 µg/L) but tended to be lower in S6 relative to the other streams. All ions increased during the two summer storms (data not shown), which indicated significant leaching from surrounding catchment soils.
Figure 1. Precipitation at Old Forge, New York, and pH at the four study sites during summer and autumn 2005.
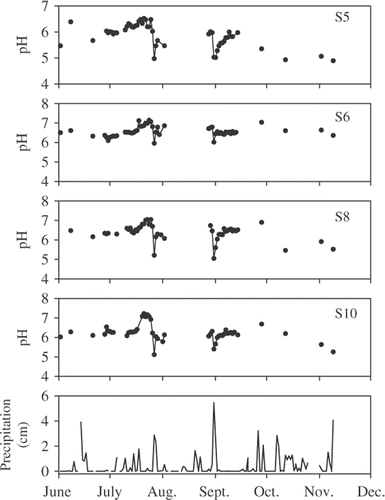
Table 2. Density of macroinvertebrate shredder taxa for the four study streams.
Leaf breakdown
In summer, leaf breakdown rates were influenced by stream (F 3,105 = 6.8, p < 0.001), species (F 1,105 = 28.5, p < 0.0001), and the interaction between stream and species (F 3,105 = 4.5, p < 0.01) (). The interaction occurred because maple breakdown was 2.2 times greater at S6 compared to the other streams (p < 0.0001) while streams did not differ in beech breakdown rates (p > 0.05). In autumn, breakdown rates were affected by stream (F 3,255 = 6.8, p < 0.001) and species (F 1,255 = 21.6, p < 0.0001). Stream 5 had greater breakdown rates compared to the other streams (p < 0.01). However, breakdown rates did not differ between single- and mixed-species packs (F 1,255 = 0.3, p = 0.57). For both summer and autumn, maple decomposed about three times faster than beech. After 6 weeks, we found that 43% of maple and 76% of beech remained in summer and 54% of maple and 80% of beech remained in autumn.
Shredders
In summer, shredder density differed among streams (F 3,89 = 5.1, p < 0.01) but not between maple and beech leaf packs (F 3,89 = 3.0, p = 0.09) (). Shredder density was greater at S5 and S10 than at S8 (p < 0.01). Shredder assemblages were dominated by species of Leuctridae and to a lesser extent Nemouridae and Lepidostoma. Pycnopsyche sp. was only found on three beech leaf packs at S6 and a single maple leaf pack at S10. Lepidostoma sp. and Pycnopsyche sp. densities did not differ among streams (p > 0.05); however, Leuctridae sp. density was greater at S10 than at S8, and Nemouridae sp. density was greatest at S5 (p < 0.05). Shredder taxa did not differ between maple and beech leaf packs (p > 0.05).
In autumn, shredder density differed among streams (F 3,137 = 3.35, p < 0.05) with density being greater at S6 relative to S5 (p < 0.05) (). However, shredder density was not influenced by pack type (F 3,137 = 1.17, p = 0.31). Shredder assemblages were dominated species of Capniiadae and Pycnopsyche. Tipula sp. was restricted to S6 but at low density. Capniidae sp., Limnophilus sp., and Tipula sp. did not differ among streams, but Pycnopsyche sp. and Lepidostoma sp. were higher at S6 compared to S5. Shredder taxa did not differ among pack types (p > 0.05).
Microbial respiration
In autumn, microbial respiration rates associated with maple and beech leaves did not differ between single- and mixed-species leaf packs (F 1,161 = 0.49, p = 0.49; ). Respiration rates differed among streams (F 3,161 = 34.4, p < 0.0001) and between maple and beech leaves (F 1,161 = 96.2, p < 0.0001), but there was an interaction between stream and species (F 3,161 = 3.6, p < 0.05). The stream × species interaction was due to a greater difference in respiration rates between beech and maple leaves at S6, S8, and S10 than at S5 and a greater difference between S6 and the other sites for maple (>1.5×, p < 0.0001) than for beech (>1.2×, p < 0.05). Respiration also was higher for beech at S5 compared to streams 8 or 10 (p < 0.05).
Discussion
Episodic acidification and leaf breakdown
Our hypotheses that leaf breakdown would be sensitive to slight differences in episodic acidification was partially supported by our data. Breakdown of maple was greatest in the circumneutral stream (S6) in summer but was highest at S5 in autumn, an episodically acidified stream with the lowest median pH. Our findings for maple breakdown in summer were consistent with other studies that have shown a decrease in breakdown rates of Acer spp. in response to acidification (Burton et al. Citation1985; Mulholland et al. Citation1987; Griffith and Perry Citation1993, 1994; Griffith et al. 1995). Maple breakdown rates in our study streams were either higher (S6 in summer, S5 in autumn) or within the range (S5, S8, and S10) of values reported for moderately or well-buffered streams in these studies. Thus, none of our streams was severely impacted by acidification, although differences in breakdown among streams suggests that further improvement is possible if acid deposition continues to decrease in this region.
We found no differences in beech breakdown among streams in summer, and beech breakdown was greatest at S5 in autumn, which contradicted the findings of Dangles et al. for Fagus spp. (Dangles and Guérold 1998, 2001; Dangles and Chauvet Citation2003; Dangles et al. Citation2004). They measured the breakdown of European beech (Fagus sylvatica) rather than American beech (F. grandifolia) as was used in our study, and the duration of their studies was much longer (>150 days) than our study (42–49 days). Hence, our study was likely not long enough to detect differences in beech breakdown among our streams in summer.
Shredder density and assemblages could not explain the difference in maple breakdown among sites for summer. Shredder density did not differ between the circumneutral stream (S6) and two episodically acidic streams (S5 and S10) in summer 2005 even though one acid-tolerant shredder, Neumoridae, was greater in S5 than in S6. The lack of response of shredders to acidification is not consistent with the findings of other studies (Allard and Moreau Citation1986; Mulholland et al. Citation1987; Griffith and Perry Citation1993; Dangles and Guérold 1998, 2001, Dangles et al. Citation2004, Pretty et al. Citation2005). However, our study streams lacked a key shredder, Peltoperla sp., which is an important component of shredder assemblages in other streams of the eastern United States (e.g., Mulholland et al. Citation1987; Griffith and Perry Citation1993). Furthermore, correlating shredder abundance or colonization rates to leaf litter breakdown can be problematic (Graca et al. Citation2001); therefore, we may have been unable to detect the functional contribution of shredders by measuring shredder abundance alone. Other methods, such as comparing leaf packs in coarse- and fine-mesh bags or relating breakdown to shredder biomass, may have been more effective in demonstrating the importance of shredders.
Microbial processing of maple litter may have contributed to higher maple breakdown at S6 relative to the other streams for summer. Respiration rates were greatest for S6 in autumn, which is consistent with other studies that have shown a decrease in microbial respiration in more acidic streams (Mulholland et al. Citation1987; Dangles et al. Citation2004). However, we did not measure respiration in summer and therefore do not know whether this trend was specific to autumn or indicative of a general difference among streams.
Biological processing was probably not responsible for the higher breakdown rates at S5 in autumn 2005. Shredder density and a key shredder, Pycnopsyche sp., were lower in S5 compared to the circumneutral stream (S6). In addition, microbial respiration rates for maple were lower in S5 than in S6. Instead, we feel that differences in physical fragmentation among sites were responsible for the differences in leaf breakdown.
Physical fragmentation is an important mechanism of leaf breakdown in stream ecosystems (Webster and Benfield 1986) and is generally greater during high-flow events (Paul and Meyer Citation1996; Ferreira et al. Citation2006a; Paul et al. Citation2006) and in high-velocity areas (e.g., riffles) compared to depositional areas (e.g., pools or debris dams) (Meyer Citation1980; Smith Citation1986; Casas Citation1996; Ferreira et al. Citation2006b; Spaenhoff et al. Citation2007). During summer, physical fragmentation was minimal because all streams were near baseflow conditions. In contrast, physical fragmentation was most likely much more important to autumn breakdown because the discharge of all streams was high due to a combination of high rainfall and low evapotranspiration rates. Although we did not measure discharge during autumn, total rainfall for nearby Old Forge, New York, was 1.8 times greater in autumn than in summer (National Climatic Data Center, Asheville, North Carolina, USA), and downstream discharge of the Black River was about 2.5 times greater in autumn than summer (US Geological Survey). Furthermore, physical fragmentation was likely greatest at S5 because it had a largest catchment area and baseflow discharge and had a steeper gradient leading to more high-velocity areas than the other streams. Ferreira et al. (Citation2006b) showed similar results when measuring breakdown of alder (Alnus glutinosa) leaves in a fourth-order stream in Portugal. They found that leaves exposed to high velocity areas had faster decomposition rates in autumn than in summer, which they attributed to greater sediment transport or shredder abundance.
Mixed-species versus single-species packs
Breakdown rates were always greater for maple than for beech in both summer and autumn and in single- and mixed-species packs. This was not surprising because the leaves of Fagacea (e.g., American beech) have slower breakdown rates than those of Aceracea (e.g., sugar maple) in stream ecosystems (Webster and Benfield Citation1986). Furthermore, Chadwick and Huryn (Citation2003) found that breakdown of maple was three times greater relative to beech in a small stream in Hubbard Brook, New Hampshire. Beech litter is much more refractory than maple because it has a higher lignin content and lignin:N ratio than that of maple (Melillo et al. Citation1982; Lovett et al. Citation2004).
Our results did not support our hypothesis that episodic acidification would reduce the influence of beech or maple on the breakdown of the other species (i.e., non-additive effects) because we did not find any evidence of non-additive effects in any of our study streams. Empirical evidence for facilitation or inhibition of breakdown of species in mixtures is conflicting. Some studies have shown non-additive effects (McArthur et al. Citation1994; Swan and Palmer Citation2004; Kominoski et al. Citation2007), whereas others have not (Leff and McArthur Citation1989; Lecerf et al. Citation2007). Taylor et al. (Citation2007) showed that the initial foliar chemistry strongly influenced whether breakdown rates were non-additive. They conducted eight trials with mixtures of five different tree species in streams of Nova Scotia, Canada, and found that breakdown rates increased only when speckled alder, a nitrogen-fixing species, was part of the mixture.
Conclusion
Leaf litter breakdown has been suggested as a way to functionally assess response of stream ecosystem to disturbances (Gessner et al.Citation1999; Gessner and Chauvet Citation2002; Young et al. Citation2008) such as episodic acidification. Based on our results, we suggest that leaf litter breakdown is sensitive to differences in episodic acidification and can be used as functional indicator of stream health if the effects of physical fragmentation are minimized through the placement of leaf packs in pools rather than riffles. However, we feel that leaf breakdown should be used in combination with other functional metrics (e.g., microbial respiration), particularly when streams differ in size or geomorphology.
Acknowledgements
We thank Devin Clifford, Anne Sammarco, and Patrick McDermott for assisting with data collection in the field and laboratory. We are grateful to Town of Webb for allowing us to use its property. Funding was provided by a Cross Disciplinary Research at Undergraduate Institutions (CRUI) grant through the US National Science Foundation.
References
- Allard , M and Moreau , G . 1986 . Leaf decomposition in an experimentally acidified stream channel . Hydrobiologia , 139 : 109 – 117 .
- Benfield , EF . 1996 . “ Leaf breakdown in stream ecosystems ” . In Methods in stream ecology , Edited by: Hauer , R and Lamberti , GA . 579 – 589 . New York : Academic Press .
- Benfield , EF and Webster , JR . 1985 . Shredder abundance and leaf breakdown in an Appalachian Mountain stream . Freshwater Biology , 15 : 113 – 120 .
- Benfield , EF , Webster , JR , Golladay , SW , Peters , GT and Stout , BM . 1991 . Effects of forest disturbance on leaf breakdown in southern Appalachian streams . Theoretical and Applied Limnology , 24 : 1687 – 1690 .
- Benjamini , Y and Hochberg , Y . 1995 . Controlling the false discovery rate – a practical and powerful approach to multiple testing . Journal of the Royal Statistical Society: Series B , 57 : 289 – 300 .
- Burton , TM , Stanford , RM and Allan , JW . 1985 . Acidification effects on stream biota and organic matter processing . Canadian Journal of Fisheries and Aquatic Sciences , 42 : 669 – 675 .
- Carlisle , DM and Clements , WH . 2005 . Leaf litter breakdown, microbial respiration and shredder production in metal-polluted streams . Freshwater Biology , 50 : 380 – 390 .
- Casas , JJ . 1996 . Environmental patchiness and processing of maple leaf litter in a backwater of a mountain stream: riffle area vs . debris dams. Archiv für Hydrobiologie , 136 : 489 – 508 .
- Chadwick , MA and Huryn , AD . 2003 . Effect of a whole-catchment N addition on stream detritus processing . Journal of the North American Benthological Society , 22 : 194 – 206 .
- Chaffin , JL , Valett , HM , Webster , JR and Schreiber , ME . 2005 . Influence of elevated As on leaf breakdown in an Appalachian headwater stream . Journal of the North American Benthological Society , 24 : 553 – 568 .
- Chamier , AC . 1987 . Effect of pH on microbial degradation of leaf litter in seven streams of the English lake district . Oecologia , 71 : 491 – 500 .
- Cummins , KW . 1974 . Structure and function of stream ecosystems . Bioscience , 24 : 631 – 641 .
- Dangles , O and Chauvet , E . 2003 . Effects of stream acidification on fungal biomass in decaying beech leaves and leaf palatability . Water Research , 37 : 533 – 538 .
- Dangles , O , Gessner , MO , Guérold , F and Chauvet , E . 2004 . Impacts of stream acidification on litter breakdown: implications for assessing ecosystem function . Journal of Applied Ecology , 41 : 365 – 378 .
- Dangles , O and Guérold , F . 1998 . A comparative study of beech leaf breakdown, energetic content, and associated fauna in acidic and non-acidic streams . Archiv für Hydrobiologie , 144 : 25 – 39 .
- Dangles , O and Guérold , F . 2001 . Linking shredders and leaf litter processing: insights from an acidic stream study . International Review of Hydrobiology , 86 : 395 – 406 .
- Driscoll , CT , Lawrence , GB , Bulger , AJ , Butler , TJ , Cronan , CS , Eagar , C , Lambert , KF , Likens , GE , Stoddard , JL and Weathers , KC . 2001 . Acidic deposition in the northeastern United States: sources and inputs, ecosystem effects, and management strategies . Bioscience , 51 : 180 – 198 .
- Ferreira , V , Elosegi , A , Gulis , V , Pozo , J and Graca , MAS . 2006a . Eucalyptus plantations affect fungal communities associated with leaf-litter decomposition in Iberian streams . Archiv für Hydrobiologie , 166 : 467 – 490 .
- Ferreira , V , Graca , MAS , de Lima , JLMP and Gomes , R . 2006b . Role of physical fragmentation and invertebrate activity in the breakdown rate of leaves . Archiv für Hydrobiologie , 165 : 493 – 513 .
- Fisher , SG and Likens , GE . 1973 . Energy flow in Bear Brook: an integrative approach to stream ecosystem metabolism . Ecological Monographs , 43 : 421 – 439 .
- Garcia , LV . 2004 . Escaping the bonferroni iron claw in ecological studies . Oikos , 105 : 657 – 663 .
- Gartner , TB and Cardon , ZG . 2004 . Decomposition dynamics in mixed-species leaf litter . Oikos , 104 : 230 – 246 .
- Gessner , MO and Chauvet , E . 2002 . A case for using litter breakdown to assess functional stream integrity . Ecological Applications , 12 : 498 – 510 .
- Gessner , MO , Chauvet , E and Dobson , M . 1999 . A perspective on leaf litter breakdown in streams . Oikos , 85 : 377 – 384 .
- Graca , MAS , Ferreira , RCF and Coimbra , CN . 2001 . Litter processing along a stream gradient: the role of invertebrates and decomposers . Journal of the North American Benthological Society , 20 : 408 – 420 .
- Griffith , MB and Perry , SA . 1993 . Colonization and processing of leaf litter by macroinvertebrate shredders in streams of contrasting pH . Freshwater Biology , 30 : 93 – 103 .
- Griffith , MB and Perry , SA . 1994 . Fungal biomass and leaf litter processing in streams of different water chemistry . Hydrobiologia , 294 : 51 – 61 .
- Griffith , MB , Perry , SA and Perry , WB . 1995 . Processing and exoenzyme production on leaves in streams of different pH . Oecologia , 102 : 460 – 466 .
- Gulis , V and Suberkropp , K . 2003 . Leaf litter decomposition and microbial activity in nutrient-enriched and unaltered reaches of a headwater stream . Freshwater Biology , 48 : 123 – 134 .
- Hagen , EM , Webster , JR and Benfield , EF . 2006 . Are leaf breakdown rates a useful measure of stream integrity along an agricultural land use gradient? . Journal of the North American Benthological Society , 25 : 330 – 343 .
- Kominoski , JS , Pringle , CM , Ball , BA , Bradford , MA , Coleman , DC , Hall , DB and Hunter , MD . 2007 . Nonadditive effects of leaf litter species diversity on breakdown dynamics in a detritus-based stream . Ecology , 88 : 1167 – 1176 .
- Lawrence , GB , Roy , KM , Baldigo , BP , Simonin , HA , Capone , SB , Sutherland , JW , Nierwicki-Bauer , SA and Boylen , CW . 2009 . Chronic and episodic acidification of Adirondack streams from acid rain in 2003–2005 . Journal of Environmental Quality , 37 : 2264 – 2274 .
- Lecerf , A , Risnoveanu , G , Popescu , C , Gessner , MO and Chauvet , E . 2007 . Decomposition of diverse litter mixtures in streams . Ecology , 88 : 219 – 227 .
- Leff , LG and McArthur , JV . 1989 . The effect of leaf pack composition on processing: a comparison of mixed and single species packs . Hydrobiologia , 182 : 219 – 224 .
- Lovett , GM , Weathers , KC , Arthur , MA and Schultz , JC . 2004 . Nitrogen cycling in a northern hardwood forest: do species matter? . Biogeochemistry , 67 : 289 – 308 .
- McArthur , JV , Aho , JM , Rader , RB and Mills , GL . 1994 . Interspecific leaf interactions during decomposition in aquatic and floodplain ecosystems . Journal of the North American Benthological Society , 13 : 57 – 67 .
- Melillo , JM , Aber , JD and Muratore , JF . 1982 . Nitrogen and lignin control of hardwood leaf litter decomposition dynamics . Ecology , 63 : 621 – 626 .
- Merrit , RW and Cummins , KW . 1996 . An introduction to the aquatic insects of North America, , 3rd , Dubuque, IA : Kendall Hunt Publishing Company .
- Meyer , JL . 1980 . Dynamics of phosphorus and organic matter during leaf decomposition in a forest stream . Oikos , 34 : 44 – 53 .
- Meyer , JL and Johnson , C . 1983 . The influence of elevated nitrate concentration on rate of leaf decomposition in a stream . Freshwater Biology , 13 : 177 – 183 .
- Mulholland , PJ , Palumbo , AV , Elwood , JW and Rosemond , AD . 1987 . Effects of acidification on leaf decomposition in streams . Journal of the North American Benthological Society , 6 : 147 – 158 .
- Neatrour , MA , Webster , JR and Benfield , EF . 2004 . The role of floods in particulate organic matter dynamics of a southern Appalachian river-floodplain ecosystem . Journal of the North American Benthological Society , 23 : 198 – 213 .
- Niyogi , DK , Lewis Jr , WM and Mcknight , DM . 2001 . Litter breakdown in mountain streams affected by mine drainage: biotic mediation of abiotic controls . Ecological Applications , 11 : 506 – 516 .
- Pascoal , C , Cassio , F and Gomes , P . 2001 . Leaf breakdown rates: a measure of water quality? . International Review of Hydrobiology , 86 : 407 – 416 .
- Pascoal , C , Pinho , M , Cassio , F and Gomes , P . 2003 . Assessing structural and functional ecosystem condition using leaf breakdown: studies on a polluted river . Freshwater Biology , 48 : 2033 – 2044 .
- Paul , MJ and Meyer , JL . 1996 . Fungal biomass of 3 leaf litter species during decay in an Appalachian stream . Journal of the North American Benthological Society , 15 : 421 – 432 .
- Paul , MJ , Meyer , JL and Couch , CA . 2006 . Leaf breakdown in streams differing in catchment land use . Freshwater Biology , 51 : 1684 – 1695 .
- Pretty , JL , Giberson , DJ and Dobson , M . 2005 . Resource dynamics and detritivore production in an acid stream . Freshwater Biology , 50 : 578 – 591 .
- Rosemond , AD , Pringle , CM , Ramirez , A , Paul , MJ and Meyer , JL . 2002 . Landscape variation in phosphorus concentration and effects on detritus-based tropical streams . Limnology and Oceanography , 47 : 278 – 289 .
- Schofield , KA , Pringle , CM , Meyer , JL and Sutherland , AB . 2001 . The importance of crayfish in the breakdown of rhododendron leaf litter . Freshwater Biology , 46 : 1191 – 1204 .
- Smith , DL . 1986 . Leaf litter processing and the associated invertebrate fauna in a tallgrass prairie stream . American Midland Naturalist , 116 : 78 – 86 .
- Spaenhoff , B , Augspurger , C and Kuesel , K . 2007 . Comparing field and laboratory breakdown rates of coarse particulate organic matter: sediment dynamics mask the impacts of dissolved nutrients on CPOM mass loss in streams . Aquatic Sciences , 69 : 495 – 502 .
- Sponseller , RA and Benfield , EF . 2001 . Influences of land use on leaf breakdown in southern Appalachian headwater streams: a multiple-scale analysis . Journal of the North American Benthological Society , 20 : 44 – 59 .
- Suberkropp , K and Chauvet , E . 1995 . Regulation of leaf breakdown by fungi in streams: influences of water chemistry . Ecology , 76 : 1433 – 1445 .
- Swan , CM and Palmer , MA . 2004 . Leaf diversity alters litter breakdown in a Piedmont stream . Journal of the North American Benthological Society , 23 : 15 – 28 .
- Taylor , BR , Mallaley , C and Cairns , JF . 2007 . Limited evidence that mixing leaf litter accelerates decomposition or increases diversity of decomposers in streams of eastern Canada . Hydrobiologia , 592 : 405 – 422 .
- Verhoeven , KJF , Simonsen , KL and McIntyre , LM . 2005 . Implementing false discovery rate control: increasing your power . Oikos , 108 : 643 – 647 .
- Warby , AF , Johnson , CE and Driscoll , CT . 2005 . Chemical recovery of surface waters across the northeastern United States from reduced inputs of acidic deposition: 1984–2001 . Environmental Science and Technology , 39 : 6548 – 6554 .
- Webster , JR and Benfield , EF . 1986 . Vascular plant breakdown in freshwater ecosystems . Annual Review of Ecology and Systematics , 17 : 567 – 594 .
- Webster , JR and Waide , JB . 1982 . Effects of forest clearcutting on leaf breakdown in a southern Appalachian stream . Freshwater Biology , 12 : 331 – 344 .
- Young , RG , Matthaei , CD and Townsend , CR . 2008 . Organic matter breakdown and ecosystem metabolism: functional indicators for assessing ecosystem health . Journal of the North American Benthological Society , 27G : 605 – 625 .