Abstract
We determined composition and trophic structure of macroinvertebrate assemblages, and also examined how environmental factors, such as riparian forest, aquatic habitat, and vertebrate predators, influenced these assemblages in relatively undisturbed headwater streams of northeastern Ohio. Using canonical correspondence analysis, we examined the relationships between macroinvertebrate assemblages, both compositional and trophic structure, and the environment. Ordination analyses showed a distinct separation of upstream and downstream macroinvertebrate faunas, while variance partitioning suggested that this may be because of the strong effects that predatory fishes and salamanders had in upstream areas. Aquatic habitat may be a strong determinant of macroinvertebrate composition and structure in these headwater streams, though this may be a reflection of site fidelity. Riparian forest habitat also explained a large portion of variation in macroinvertebrate functional feeding guild structure, emphasizing the connection between terrestrial and aquatic ecosystems in these headwater stream systems.
Introduction
It has long been recognized that streams and rivers are integrally tied to riparian areas, especially in the headwaters of most watersheds (Minshall Citation1967; Vannote et al. Citation1980; Richards et al. Citation1996; Wallace et al. Citation1997; Nakano et al. Citation1999). In these headwater streams, biota is largely dependent on allochthonous inputs from surrounding riparian forests for nutrients and habitat (Vannote et al. Citation1980; Richards et al. Citation1996). Recent research suggests that headwater streams comprise up to 80% of a watershed's stream network (Meyer and Wallace 2000) and that these small headwater streams should be the focus of restoration efforts because of their potential importance for nutrient processing (Peterson et al. Citation2001). Historically, however, many of the headwater systems in Ohio and elsewhere in the northeastern United States have received little attention from researchers and have often been ignored by land managers and developers.
As headwater streams often do not have pools of sufficient depth to sustain fish populations, recent monitoring work in Ohio has been using macroinvertebrate and salamander assemblages as indicators of water quality in these systems (OEPA Citation2002). Although macroinvertebrates, salamanders, and fish can all be found in stream ecosystems in at least some portion of their lives, they have very different physiologies and life histories; therefore, they may react differently to various disturbances or processes at different scales (Karr Citation1981). Macroinvertebrates are influenced strongly by microhabitat variables (Vinson and Hawkins Citation1998; Stewart et al. Citation2003; Kaller and Hartman Citation2004; Sandin and Johnson Citation2004) and thus have been described as good indicators of local habitat conditions (Plafkin et al. Citation1989; Lammert and Allan Citation1999). Salamanders are influenced by both microhabitat and variables operating at larger (e.g., sub-basin) scales (Welsh and Ollivier Citation1998; Hyde and Simons Citation2001; Lowe and Bolger 2002; Wilson and Dorcas Citation2003) and replace fish as the top vertebrate predator in many headwater stream systems (OEPA Citation2002). Although more common in recent years (e.g., Jackson and Harvey Citation1993; Lammert and Allan Citation1999; Williams et al. Citation2003b), studies that examine how multiple taxa interact and are structured by habitat are lacking for many types of stream systems, especially headwater streams. Knowledge of factors affecting assemblage structure of biota inhabiting headwater streams is needed to guide restoration and management of these ecosystems.
The objective of our study was to investigate relationships between macroinvertebrate assemblages and their environment in relatively undisturbed headwater streams. Specifically, we determined composition and trophic structure of macroinvertebrate assemblages and examined how environmental factors, such as riparian forest, aquatic habitat, and vertebrate predators, influenced these assemblages. To understand how watershed position may affect these relationships, we sampled in both upstream and downstream headwater stream reaches.
Materials and methods
The study was conducted in Cuyahoga Valley National Park, OH, an area of over 13,400 ha comprising relatively undeveloped land along 35 km of the Cuyahoga River between the cities of Cleveland and Akron. Much of the park is characterized by steep, forested ravines formed along the multiple tributaries to the Cuyahoga River. The forests are second-growth (>70 years old) and composed of mixed-mesophytic species representative of the Erie Gorges ecoregion. We focused on four perennial headwater streams – Boston Run, Langes Run, Riding Run, and an unnamed stream that we refer to as Perkins Trail Run. Streams were selected based upon similar riparian forest age (mature second-growth), riparian forest composition and structure (native species in a multi-cohort stand), geomorphic landforms (the presence of fluvial floodplains and terraces), stream gradient (1–5%), and substrate (minimal siltation observed) ().
Table 1. Characteristics of four headwater streams and their associated upstream and downstream reaches sampled within the Cuyahoga Valley National Park, OH.
We sampled upstream and downstream reaches of each stream in the summer of 2004. Each 100-m reach was randomly selected and sampled for benthic macroinvertebrates, riparian forest, aquatic habitat, fishes, and salamanders.
Macroinvertebrates
Each reach was sub-divided into three approximately equal segments, which were sampled for macroinvertebrates using both a Surber sampler and D-frame net. The single Surber sample was collected in each stream segment, and the stream margins within each segment were probed with the D-frame net for 5 min. The samples were preserved in 95% ethanol, with rose bengal stain. In the laboratory, samples were sorted, and macroinvertebrates and identified to family level.
Abundance for each macroinvertebrate family was summarized by reach, and the following diversity indices were calculated: richness (S = number of families present), Shannon diversity index (H′ = ∑pi ln pi , where pi is the proportion of the ith species), and evenness (E = H′/ln S). Anderson–Darling normality tests indicated non-normality of the assemblage composition data, so Mann–Whitney U-tests were run to test for differences between mean diversity indices between watershed positions (upstream vs. downstream stream reaches). An alpha level equal to 0.10 was used to indicate significant differences in all statistical analyses conducted.
To analyze trophic structure, each family was categorized into one of four functional feeding guilds (Merritt and Cummins Citation1984; Allan Citation1995; Williams et al. Citation2002; ): collectors (collector-gatherers and collector-filterers), predators (engulfers and piercers), scrapers (scrapers and grazers), or shredders. Functional feeding guilds were summarized for each stream reach and checked for normality with Anderson–Darling normality tests. Following square root transformation, the student t-test were used to test for differences in the number of individuals per guild between watershed positions.
Table 2. Mean (1 SD) of macroinvertebrate family compositional diversity indices and mean (1 SD) of the proportion of structural.
Riparian forest habitat
To characterize the riparian forest adjacent to each sample reach, transects were established perpendicular to stream-flow across the stream valley at 33, 66, and 100 m within each reach. For each transect, circular plots (400 m2) were centered on stream valley landforms (e.g., floodplain, terrace, valley toe-slope), and all overstory trees greater than 10 cm diameter at breast height (DBH; 1.4 m) were measured, and basal area and stem density estimated. Using a concave spherical densiometer held at DBH, percentage of riparian forest cover was estimated from the center of the stream for each segment and averaged for the entire stream reach.
Initial analyses suggested that several variables were not normally distributed; consequently, percentage of riparian forest cover was arc-sin square root transformed, stem density was natural log transformed, and basal area was square root transformed. Differences in mean riparian forest cover, basal area, and stem density between watershed positions were tested with the student t-test. Riparian overstory species diversity indices for each stream reach were non-normal, and no transformations adequately normalized these variables; thus, differences were tested between watershed positions using Mann–Whitney U-tests.
Aquatic habitat
To characterize the aquatic habitat of each sample stream reach, channel substrate type (percentages of sand, silt, gravel, cobble, and boulder) was estimated visually within a 1 m wide area of stream channel bottom at the end of each segment (0, 33, 66, and 100 m) and averaged for the entire stream reach (Williams et al. Citation2003a). The proportion of large wood habitat was estimated visually for each segment and averaged for the entire stream reach. Additionally, the width of the bankfull channel was measured at the end of each segment and averaged for the entire sample reach to determine the approximate area sampled.
Channel substrate and large wood habitat percentage data were arc-sin square root transformed for normally distributed data and tested for significant differences in mean values between watershed positions using the student t-test.
Vertebrate sampling
At all sites where present, fishes were sampled with single-pass electrofishing using a backpack unit (Smith Root, Inc. Model LR-24 Electrofisher), encompassing the entire 100 m reach. The fishes were preserved in the field and identified later. Salamanders were sampled within a randomly selected 30-m section of each stream reach by turning over rocks and sifting leaf packs within the stream and the adjacent stream bank. Sampling took place for a minimum of 30 min or the length of time it took to search the entire section. Salamanders were identified and released at the sites of capture. Only species with aquatic larval life stages were considered to be vertebrate predators of the macroinvertebrates studied.
Fish and salamander species abundance were summarized for each sample stream reach, and density was calculated. Fish and salamander abundance data was combined to represent vertebrate predators of macroinvertebrate assemblages, and vertebrate diversity indices were calculated for each stream reach. Density and diversity indices were square root transformed and tested for mean differences between watershed positions using the student t-test.
Macroinvertebrate assemblage-environment analyses
We used canonical correspondence analysis (CCA) to examine the relationships between macroinvertebrate assemblages, both compositional and trophic structures, and the measured environmental factors, both biotic and abiotic. To characterize relationships between variation in macroinvertebrate assemblage composition and each group of environmental factors, three separate CCAs were conducted, one for each group of environmental factors analyzed – riparian forest habitat, aquatic habitat, and vertebrates. The macroinvertebrate composition matrix for all three CCAs contained abundance data for all macroinvertebrate families sampled in each reach. The riparian forest habitat matrix included percentage of riparian forest cover, basal area, overstory diversity, and stem density, while the aquatic habitat matrix included percentages of silt, sand, gravel, cobble, boulders, and large wood, and the vertebrate matrix included fish density, salamander density, and predator diversity.
A second series of three CCAs was conducted to characterize the relationships between variation in macroinvertebrate assemblage trophic structure and each group of environmental factors. The macroinvertebrate trophic structure matrix contained the abundance of individuals per guild at each sample reach, while the riparian forest, aquatic habitat, and vertebrate matrices were the same as those used for the macroinvertebrate composition CCA analyses.
Since environmental factors occur together spatially and often interact, partial CCA was used to further partition macroinvertebrate assemblage variation (Borcard et al. Citation1992; Williams et al. Citation2002) into seven variance components – riparian forest habitat, aquatic habitat, vertebrate predators, and the shared variation between the components that cannot be partitioned into pure effects. Variance partitioning is done through a series of CCAs where pure effects of each variable are determined by treating the other variables as covariates. In partial CCA, the number of variables in the second multivariate matrix used must equal N − 1, where N equals the number of variance components to be explained to avoid over-fitting the model. Six variables were selected based on the individual CCA biplot scores, two from each group of measured environmental factors with the two highest scores in each group selected. To partition variation in the macroinvertebrate composition, the following environmental variables were used: basal area, stem density, percentages of silt and sand, salamander density, and fish density. To partition variation in the macroinvertebrate trophic structure, the following environmental variables were used: percentage of riparian forest cover, basal area, percentage of silt, percentage of boulder, salamander density, and fish density.
Results
A total of 12,691 individuals comprising 12 orders and 45 families of macroinvertebrates was collected in the eight sample reaches. Diversity indices indicated downstream reaches have higher mean richness of macroinvertebrate families, higher mean Shannon diversity index values, and assemblages that on average are more evenly distributed than the upstream reaches (), though none are statistically different (Mann–Whitney W = 15.5, p = 0.561; W = 14.0, p = 0.312; W = 17.0, p = 0.885, respectively). The mean abundance of macroinvertebrates collected was higher in downstream reaches than upstream, 1892 versus 1280 individuals, respectively. Functional feeding guild proportions also differed between upstream and downstream reaches. Specifically, mean percentage of shredder families was significantly higher in upstream reaches than downstream (5.76% ± 4.55 vs. 0.78% ± 0.28; T = 2.81, p = 0.04). Very few scraper families were collected and proportions were similar between watershed positions. Collector families were dominant in both upstream and downstream reaches, but there was no significant difference between upstream and downstream reaches (92.67% ± 1.15 and 86.24% ± 5.06, respectively; T = −1.08; p = 0.33).
Table 3. Mean (1 SD) of measured riparian forest and aquatic habitat and vertebrate predator environmental factors by headwater reach watershed position.
Riparian forest habitat differed between watershed positions with downstream reach riparian forests having higher mean species richness and higher mean Shannon diversity index values than upstream reach forests; however, these differences were not significant (W = 529.0, p = 0.13; W = 538.5, p = 0.17, respectively) (). Upstream and downstream reach riparian forests were not significantly different in species distribution evenness (W = 630.0, p = 1.00) (). Mean basal area of riparian overstory trees was higher along upstream reaches than downstream, but not significantly different (10.38 m2/ha ± 7.73 and 8.60 m2/ha ± 6.17, respectively; W = 666.0, p = 0.60). Mean stem density was higher along downstream reaches than upstream, but not significantly different (161 trees/ha ± 99 and 125 trees/ha ± 49, respectively; W = 550.5, p = 0.23). Mean percent riparian forest cover was significantly higher along upstream reaches than downstream (80.00% ± 7 and 55.31% ± 16.78, respectively; T-value = 2.81, p = 0.05).
Aquatic habitat also differed between watershed positions with downstream reaches having higher mean percentages of silt, sand, and gravel in channel substrates than upstream reaches (), though differences were not significant (T-value = −1.42, p = 0.23; T-value = −1.42, p = 0.21; T-value = −0.98, p = 0.38, respectively). Upstream reaches had higher mean percentage of cobble and boulder in the channel than downstream reaches (), though differences were again not significant (T-value = 0.38, p = 0.72; T-value = 1.44, p = 0.21, respectively). Mean percentage of large wood habitat was higher in upstream reaches than downstream but the difference was not significant (15.00% ± 3.95 vs. 12.19% ± 3.29, T-value = 1.07, p = 0.33).
Vertebrate predators were present in all eight sample reaches; however, salamanders and fishes were not located together at all reaches. Salamanders were present at seven of the eight stream reaches, specifically all four upstream and three downstream reaches. Two salamander species with aquatic larval stages were collected – the northern dusky salamander (Desmognathus fuscus fuscus) and the two-lined salamander (Eurycea bislineatea). Fishes were present at five of the eight stream reaches, specifically one upstream reach and all four downstream reaches. While 13 fish species were collected, two species were most abundant and present at all sample reaches with fish – the black-nosed dace (Rhinichthys atratulus) and the northern creek chub (Semotilus atromaculatus). Vertebrates had higher richness in downstream reaches, had higher evenness, and were more diverse than in upstream reaches (); however, the differences were not statistically significant (T-value = −1.63, p = 0.202; T-value = −1.78, p = 0.173; and T-value = −2.04, p = 0.111, respectively). Salamander density tended to be higher in upstream reaches than downstream, but this difference was not significant (0.10 m−2 ± 0.11 and 0.02 m−2 ± 0.02, respectively; T-value = 2.13, p = 0.100). Fish density was significantly higher downstream (0.14 m−2 ± 0.11 vs. 0.02 m−2 ± 0.05, respectively; T-value = −2.45, p = 0.058).
The CCA revealed that measured environmental factors were strong indicators of upstream and downstream reach characteristics, while macroinvertebrate assemblage composition was relatively homogeneous in its distribution and may be more related to site-specific differences than the measured environmental factors (Figures ). Similarly, macroinvertebrate trophic structure are not well-explained by the measured environmental factors; however, the factors successfully discriminated between the upstream from downstream reaches. For riparian forest habitat, upstream reaches were positively associated with increased percentage of riparian forest cover and basal area, while downstream reaches were positively associated with increased stem density and higher overstory species diversity. Upstream sample reach aquatic habitat had more boulders, while downstream reaches were positively associated with silt and gravel. Reaches positively associated with percentage of cobble and boulders were negatively associated with percentage of sand. Upstream reaches were positively associated with higher salamander density, while downstream reaches are positively associated with higher fish density and predator diversity.
Figure 1. CCA triplots of the relationship between the riparian environment and macroinvertebrate family composition (a) and macroinvertebrate functional feeding guilds (b), along four headwater streams of Cuyahoga Valley National Park, OH. Black circles refer to upstream reaches while gray circles refer to downstream reaches.
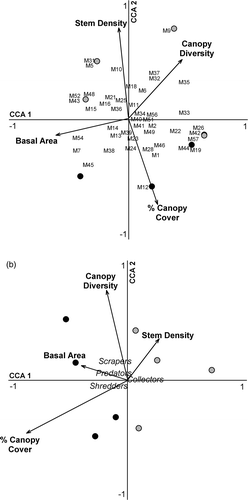
Figure 2. CCA triplots of the relationship between the aquatic environment and macroinvertebrate family composition (a) and macroinvertebrate functional feeding guilds (b), along four headwater streams of Cuyahoga Valley National Park, OH. Black circles refer to upstream reaches while gray circles refer to downstream reaches.
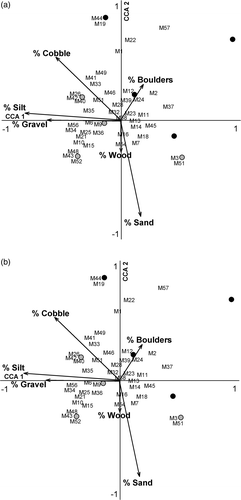
Figure 3. CCA triplots of the relationship between the vertebrate predators and macroinvertebrate family composition (a) and macroinvertebrate functional feeding guilds (b), along four headwater streams of Cuyahoga Valley National Park, OH. Black circles refer to upstream reaches while gray circles refer to downstream reaches.
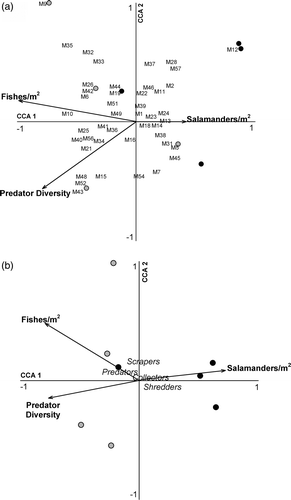
Variance partitioning between the three groups of measured environmental factors indicate that 89% of the variation in macroinvertebrate assemblage composition could be explained by the three factor groups, with 25.5% explained by the shared variation between aquatic habitat and vertebrate predators and 24.3% explained by vertebrate predators alone (). While variation in composition could be explained by shared variation and individual groups, variation in macroinvertebrate trophic structure was explained primarily by the individual groups of environmental factors with essentially no shared variation. Aquatic habitat explained the most variation in structure (37.2%); riparian forest habitat explained 27.9%. The three groups of environmental factors explained 93% of the variation in the trophic structure of macroinvertebrate assemblages.
Discussion
Overall, we found a trend of higher richness, diversity, and abundance of macroinvertebrates in downstream reaches, though this trend was not statistically significant. This pattern of higher family richness downstream is consistent with stream ecology models, such as the river continuum concept (Vannote et al. Citation1980) that predict more complex assemblage structure of macroinvertebrates in downstream reaches. These models also predict higher proportion of shredder taxa upstream, which process leaf litter that enters the system (Minshall et al. Citation1985). Also predicted by the models is the pattern that fewer scrapers are found in upstream areas. Scrapers tend to become more abundant when canopies are open allowing periphyton growth due to increased sunlight on the water column. Most of the sites we sampled had relatively closed riparian forest overstories and thus did not allow for sufficient growth of periphyton to permit greater abundances of scrapers. Sites in our study area were dominated by collector taxa, specifically chironomids. It may be that the high degree of disturbance from surrounding urbanization created an environment that allowed chironomid taxa to dominate the assemblages similar to rural–urban interface headwater streams in Connecticut (Urban et al. Citation2006). This pattern is corroborated by the increased dominance of finer substrata in downstream reaches.
Ordination analyses showed a distinct separation of upstream and downstream macroinvertebrate faunas. Variance partitioning suggested this may be because of the strong effects that predatory fishes and salamanders have in upstream areas. When water levels in headwater stream reaches are low, especially in the dry periods of the summer, predators can act to structure the macroinvertebrate assemblages, particularly in the smallest streams (Williams et al. Citation2003a). Variance partitioning also suggested that aquatic habitat may be a strong determinant of macroinvertebrate composition and structure in these headwater streams, which follows what has been found in other headwater systems (Angradi Citation1996; Johnson et al. Citation2003). However, the macroinvertebrate taxa tended to concentrate towards the center of the CCA ordination diagrams, suggesting that local site fidelity may be an even larger controlling factor on assemblage composition and structure than the measured environmental factors in this study. This may indicate that there is strong site-level fidelity in the assemblages. Thus, the spatial location of sites may have a stronger influence than the local environmental conditions. This pattern has been documented in other headwater systems (Williams et al. Citation2002, Citation2005). In previous work, this has been because of the overriding influence of refugia areas during low water. The location of refugia habitat, in this case deep pools, may be critically important areas of future recolonization and may override many species-environment relationships.
Because of the importance of aquatic habitat, particularly the availability of refugia habitat, to macroinvertebrate assemblage composition and functional feeding guild structure, high quality aquatic habitat needs to be a priority for ecological restoration and management of these headwater systems. Additionally, the effects of surrounding urbanization may be more far reaching than known as these headwater stream sample reaches were selected due to their relatively undisturbed condition; yet, the macroinvertebrate fauna was still dominated by chironomid species. The effects of human disturbance may be affecting micro-habitat variables not sampled in this study.
In addition to aquatic habitat, riparian forest habitat also explained a large portion of variation in macroinvertebrate functional feeding guild structure, emphasizing the connection between terrestrial and aquatic ecosystems in these headwater stream systems. For example, in this study, large wood was considered a component of the aquatic habitat even though its source is the riparian forest. Also, the nearly complete riparian forest cover that is characteristic of headwater streams is responsible for regulating temperature as well as organic matter and sediment input into the aquatic system, all of which are critical to the quality of aquatic habitat and macroinvertebrate assemblages.
Acknowledgements
Funding and salaries of this research were provided by the Ohio Agricultural Research and Development Center (OARDC) through an OARDC SEEDS grant. We thank Lisa Petit and staff of the Cuyahoga Valley National Park for assistance with site identification and sampling permission.
References
- Allan , JD . 1995 . Stream ecology: structure and function of running waters , 388 London : Chapman and Hall .
- Angradi , TR . 1996 . Inter-habitat variation in benthic community structure, function, and organic matter storage in 3 Appalachian headwater streams . Journal of the North American Benthological Society , 15 : 42 – 63 .
- Borcard , D , Legendre , P and Drapeau , P . 1992 . Partialling out the spatial component of ecological variation . Ecology , 73 : 1045 – 1055 .
- Hyde , EJ and Simons , TR . 2001 . Sampling plethodontid salamanders: Sources of variability . Journal of Wildlife Management , 65 : 624 – 632 .
- Jackson , DA and Harvey , HH . 1993 . Fish and benthic invertebrates: community concordance and community-environment relationships . Canadian Journal of Fisheries and Aquatic Sciences , 50 : 2641 – 2651 .
- Johnson , LB , Breneman , DH and Richards , C . 2003 . Macroinvertebrate community structure and function associated with large wood in low gradient streams . River Research and Applications , 19 : 199 – 218 .
- Kaller , MD and Hartman , KJ . 2004 . Evidence of a threshold level of fine sediment accumulation for altering benthic macroinvertebrate communities . Hydrobiologia , 518 : 95 – 104 .
- Karr , JR . 1981 . Assessment of biotic integrity using fish communities . Fisheries , 6 : 21 – 27 .
- Lammert , M and Allan , JD . 1999 . Assessing biotic integrity of streams: effects of scale in measuring the influence of land use/cover and habitat structure on fish and macroinvertebrates . Environmental Management , 23 : 257 – 270 .
- Lowe , WH and Bolger , DT . 2002 . Local and landscape-scale predictors of salamander abundance in New Hampshire headwater streams . Conservation Biology , 16 : 183 – 193 .
- Merritt , RW and Cummins , KW . 1984 . Introduction to the aquatic insects of North America , Dubuque, IA : Kendall/Hunt Publishing .
- Meyer , JL and Wallace , JB . 2000 . “ Lost linkages and lotic ecology: rediscovering small streams ” . In Ecology: an achievement and challenge , Edited by: Press , MC , Huntly , NJ and Levin , SA . 295 – 317 . Ames, IA : Blackwell Science .
- Minshall , GW . 1967 . Role of allochthonous detritus in the trophic structure of a woodland springbrook community . Ecology , 48 : 139 – 149 .
- Minshall , GW , Cummins , KW , Petersen , RC , Bruns , DA and Cushing , CE . 1985 . Developments in stream ecosystem theory . Canadian Journal of Fisheries and Aquatic Science , 42 : 1045 – 1055 .
- Nakano , S , Miyasaka , H and Kuhara , N . 1999 . Terrestrial-aquatic linkages: riparian arthropod inputs alter trophic cascades in a stream food web . Ecology , 80 : 2435 – 2441 .
- OEPA 2002 . Field evaluation manual for Ohio's primary headwater habitat streams, Version 1.0, July 2002. Division of Surface Water, Columbus, OH
- Peterson , BJ , Wollheim , WM , Mullolland , PJ , Webster , JR , Meyer , JL , Tank , JL , Marti , E , Bowden , WB , Valett , HM Hershey , AE . 2001 . Control of nitrogen export from watersheds by headwater streams . Science , 292 : 86 – 89 .
- Plafkin , JL , Barbour , MT , Porter , KD , Gross , SK and Hughes , RM . 1989. Rapid bioassessment protocols for use in stream and rivers: macroinvertebrates and fish. EPA/444/4-89-011. US Environmental Protection Agency, Washington, DC
- Richards , C , Johnson , LB and Host , GE . 1996 . Landscape-scale influences on stream habitats and biota . Canadian Journal of Fisheries and Aquatic Sciences , 53 : 295 – 311 .
- Sandin , L and Johnson , RK . 2004 . Local, landscape and regional factors structuring benthic macroinvertebrate assemblages in Swedish streams . Landscape Ecology , 19 : 501 – 514 .
- Stewart , TW , Shumaker , TL and Radzio , TA . 2003 . Linear and nonlinear effects of habitat structure on composition and abundance in the macroinvertebrate community of a large river . The American Midland Naturalist , 149 : 293 – 305 .
- Urban , MC , Skelly , DK , Burchsted , D , Price , W and Lowry , S . 2006 . Stream communities across a rural-urban landscape gradient . Diversity and Distributions , 12 : 337 – 350 .
- Vannote , RL , Minshall , GW , Cummins , KW , Sedell , JR and Cushing , CE . 1980 . The river continuum concept . Canadian Journal of Fisheries and Aquatic Sciences , 37 : 130 – 137 .
- Vinson , MR and Hawkins , CP . 1998 . Biodiversity of stream insects: variation at local, basin, and regional scales . Annual Review of Entomology , 43 : 271 – 293 .
- Wallace , JB , Eggert , SL , Meyer , JL and Webster , JR . 1997 . Multiple trophic levels of a forest stream linked to terrestrial litter inputs . Science , 277 : 102 – 104 .
- Welsh , HH and Ollivier , LM . 1998 . Stream amphibians as indicators of ecosystem stress: a case study from California's redwoods . Ecological Applications , 8 : 1118 – 1132 .
- Williams , LR , Bonner , TH , Hudson , III JD , Williams , MG , Leavy , TR and Williams , CS . 2005 . Interactive effects of environmental variability and military training on stream biota of three headwater drainages in Western Louisiana . Transactions of the American Fisheries Society , 134 : 192 – 206 .
- Williams , LR , Taylor , CM and Warren Jr , ML . 2003a . Influence of fish predation on assemblage structure of macroinvertebrates in an intermittent stream . Transactions of the American Fisheries Society , 132 : 120 – 130 .
- Williams , LR , Taylor , CM , Warren Jr , ML and Clingenpeel , JA . 2002 . Large-scale effects of timber harvesting on stream systems in the Ouachita Mountains, Arkansas . Environmental Management , 29 : 76 – 87 .
- Williams , LR , Taylor , CM , Warren Jr , ML and Clingenpeel , JA . 2003b . Environmental variability, historical contingency, and the structure of regional fish and macroinvertebrate faunas in Ouachita Mountain stream systems . Environmental Biology of Fishes , 67 : 203 – 216 .
- Wilson , JD and Dorcas , ME . 2003 . Effects of habitat disturbance on stream salamanders: implications for buffer zones and watershed management . Conservation Biology , 17 : 763 – 771 .