Abstract
Experimental ponds (0.4 ha) were used to evaluate the effects of vegetation density on bluegill (Lepomis macrochirus) diet and growth in the absence of pelagic predation risk. Fish (30–50 mm, total length) were stocked at a rate of 15 kg per pond. By the end of the 3-month experiment, bluegill in the low vegetation treatment (109 g m−2 ± 21.0 SE, n = 4) had grown 20% longer than the fish in the high vegetation treatment (712 g m−2 ± 54.3 SE, n = 4) despite having similar mean stomach fullness. Bluegill in the high vegetation treatment ingested more gastropods and odonates and less benthic prey (chironomids) than did the fish in the low vegetation treatment. Very little pelagic zooplankton were eaten by fish in either treatment despite the lack of predation risk in the open water habitat. These results suggest that bluegill chose to forage in a vegetated habitat even in the absence of predation risk, resulting in reduced growth.
Introduction
Many juvenile fishes select structurally complex habitats, such as submerged vegetation, in response to predation risk (Magnhagen Citation1988; Persson and Eklov Citation1995; Olson et al. Citation2003b; Abdel-Tawwab Citation2005). However, structurally complex habitats can reduce foraging return and growth rate of juvenile fishes (Persson Citation1991; Diehl and Eklov Citation1995; Shoup et al. Citation2003). The optimal diet for juvenile bluegill (Lepomis macrochirus) is often considered to be pelagic zooplankton (Mittelbach Citation1981). Yet juveniles often forage suboptimally in structurally complex habitats, such as dense vegetation, to avoid pelagic predation risk (Mittelbach Citation1981), which can be a major factor determining recruitment to age-1 (Santucci and Wahl Citation2003). Studies of bluegill behavior in the laboratory (Gotceitas and Colgan Citation1987; Savino and Stein Citation1989; Shoup et al. Citation2003) and in ponds (Werner et al. Citation1983a) both show that bluegill spend more time in structurally complex habitats when predators are present. Furthermore, structurally complex habitats can negatively affect foraging returns of juvenile bluegill (Mittelbach Citation1981; Gotceitas Citation1990a; Pothoven et al. Citation1999), and vegetation density negatively correlates with bluegill growth (Shoup et al. Citation2007).
However, other studies have found that fast-growing juvenile bluegill eat mostly littoral invertebrates (Schneider Citation1999; Olson et al. Citation2003a) or that increased vegetation density either has no effect (Savino et al. Citation1992; Hayse and Wissing Citation1996) or even increases bluegill growth (Richardson et al. Citation1998), suggesting that foraging in vegetated habitat may not be suboptimal. The lack of growth effect may, in part, be caused by the greater biomass of prey resources that occurs as vegetation biomass increases (Gerking Citation1957; Gotceitas and Colgan Citation1990; Savino et al. Citation1992). All these studies were conducted in environments with different levels of predation risk, and therefore, it is difficult to determine if changes in growth were related to predator avoidance behavior or vegetation-related effects on prey choice or foraging efficiency. Therefore, the role of vegetation density in influencing juvenile bluegill diet and growth remains unclear.
Foraging by fishes can be affected in many ways by habitat, which can influence prey abundance (Gerking Citation1957; Savino et al. Citation1992; Barwick Citation2004), behavior patterns (Savino and Stein Citation1989), predator attack success (Savino and Stein Citation1982; Gotceitas and Colgan Citation1987), and ultimately prey selection (Schramm and Zale Citation1985; Dibble and Harrel Citation1997; Shoup and Wahl Citation2009) and competitive interactions (Abrahams Citation1994; Wellenreuther et al. Citation2007; Robertson et al. Citation2008). Therefore, changes in vegetation abundance could cause changes in diet and growth rate of juvenile bluegill that are independent of the effects of predation risk.
Previous studies of the role of vegetation densities in the absence of predation risk have used enclosures in lakes (Savino et al. Citation1992) or ponds (Crowder and Cooper Citation1982) that had homogeneous vegetation growth rather than the combination of littoral and pelagic habitat that exists in most natural systems. Werner et al. (Citation1983b) conducted an unreplicated pond experiment with both littoral and pelagic habitat, but with a single density of emergent vegetation. Because of the conflicting information in the literature related to optimal foraging habitat for juvenile bluegill in natural systems and the complexity of predation risk-foraging return trade-offs, additional information is needed to quantify the effects of vegetation density independent of the influence of predation risk. In this study, replicated ponds were used to assess the effect of vegetation density on diet and growth of juvenile bluegill in the absence of predation risk.
Methods
Eight experimental 0.4-ha ponds (mean depth of 1 m) were used to evaluate the effects of habitat complexity on growth of small bluegill. The ponds were located at the Sam Parr Biological Station in Kinmundy, Illinois, USA. Vegetation was dominated by brittle naiad (Najas minor), with lesser amounts of Chara spp. and coontail (Ceratophyllum demersum). Sparse patches of emergent vegetation such as water primrose (Ludwigia hexapetala) and cattail (Typha spp.) were also present. The ponds, containing established vegetation, were drained and refilled with lake water from adjacent Forbes Lake (filtered to exclude fish) in mid-June to allow for colonization of zooplankton and benthic invertebrates, and to allow time to establish the vegetation treatments. After 1 month (11 July), each pond was stocked with 15 kg of young-of-year bluegills (30–50 mm, total length (TL), approximately 20,000 fish per pond) to produce a natural density for small ponds (Hackney Citation1979; Wahl and Stein Citation1988).
In order to create the two levels of vegetation complexity, grass carp (Ctenopharyngodon idella; ∼200 mm, TL) were used to control macrophytes in four randomly selected ponds (low vegetation treatment). The four other ponds (high vegetation treatment) received four grass carp that were enclosed in a 2 × 2 × 1.5-m mesh cage and fed grass clippings, so that nutrient flux into the water column from fish was similar between treatments. Grass was fed at a rate of 40% of total estimated grass carp body weight per day (Wiley and Wike Citation1986). Ponds had similar topography and were sufficiently deep at their deepest point that even in the high vegetation treatment approximately 20% of the pond surface area did not contain vegetation.
Plant biomass was determined by identifying and weighing (to the nearest g) the above-ground plant material contained within 1-m diameter rings (0.785 m2) placed at 10 random locations within the littoral zone of each pond. Plant biomass was determined in this fashion every 2 weeks to verify that the treatments were maintained throughout the experiment.
Bluegill diet data were collected by dissection from up to 15 fish per pond during the last week of August and again at the end of the experiment. Stomachs were preserved in 95% ethanol until they could be analyzed in the laboratory where stomach contents were identified to the lowest possible taxonomic group (typically family). Once sorted and enumerated, up to 10 prey items of each taxa from each fish were measured using an optical micrometer (±0.04 mm). Prey lengths were converted to pre-digested dry-weight biomass using published regression equations (Dumont et al. Citation1975; Smock Citation1980; Culver et al. Citation1985; Sample et al. Citation1993; Benke et al. Citation1999). On the final day of the experiment (28 September), the ponds were drained and 200 bluegill from each treatment were counted and measured to the nearest mm, TL.
Because vegetation was measured over the course of the experiment, a repeated-measures ANOVA (SAS Proc GLM; SAS Institute Citation2004) was used to determine if the experimental design was successful in creating high and low vegetation treatments. Bluegill at the end of the experiment were measured (mm, TL) and a mean size was determined for each pond. Mean bluegill size was analyzed using an ANOVA with vegetation treatment as the main effect. Length-frequency distributions between treatments were compared with a Kolmogorov–Smirnov test. Mean stomach fullness (prey biomass divided by bluegill mass) was analyzed using a repeated-measures ANOVA with vegetation treatment and date as factors. Bluegill diet composition was analyzed using two-way MANOVA (ponds treated as subjects, vegetation density and date as treatment factors) conducted with SAS Proc mixed (Wright Citation1998; SAS Institute Citation2004). Separate MANOVA analyses were performed for percent by weight and percent by number diet metrics. Only taxa that constituted ≥2% of the diet were used as response variables; other taxa were pooled as ‘other taxa.’ Significant statistical results (p < 0.05) in the above tests were subsequently analyzed with a Tukey's test to compare means. Data were transformed (log or arcsine, as appropriate) to meet the assumptions of normality.
Results
While vegetation biomass in the low vegetation treatment was relatively constant over time (mean 109 g m−2 ± 21 SE), biomass in the high vegetation treatment (mean 712 g m−2 ± 54 SE) first increased and then decreased during the summer but was always significantly higher than in the low vegetation treatment (p ≤ 0.01 for all comparisons; treatment × date interaction F 5,30 = 5.42, p < 0.01; ). The low vegetation treatment ranged from 72 to 118 g m−2, with the highest values occurring during the midpoint of the experiment. Vegetation density in the high treatment was 556 g m−2 at the beginning of the experiment and increased to 867 g m−2 by mid-August before it declined to 549 g m−2 by the end of the experiment. Vegetation growth was relatively uniform in both treatments.
Figure 1. Mean vegetation biomass (g m−2 wet weight ± standard error (SE)) of high (n = 4; hatched bars) and low (n = 4; open bars) vegetation treatments in eight 0.4-ha ponds at the Sam Parr Biological Station, Kinmundy, IL, used to evaluate the effects of habitat complexity on growth of small bluegill. Mean vegetation biomass was 109 g m−2 ± 21.0 SE in the low vegetation treatment and 712 g m−2 ± 54.3 SE in the high vegetation treatment over the entire experiment.
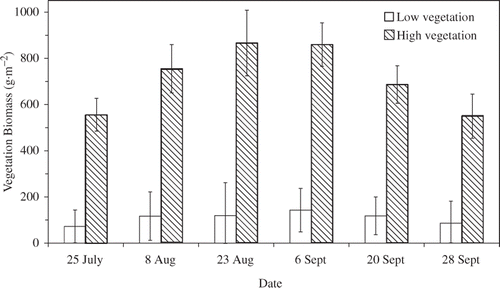
Growth of bluegill was observed in all ponds and differed between treatments (). Pooled length-frequencies from high and low vegetation treatments at the end of the experiment were significantly different (KSa = 3.84, p < 0.01), being skewed toward larger sizes in the low vegetation treatment. By the end of the experiment, bluegill from the low vegetation treatment were significantly longer (mean TL ± SE; 84.2 ± 2.5 mm) than bluegill from the high vegetation treatment (74.7 ± 2.4; F 1,6 = 7.53, p = 0.03; ).
Figure 2. Bluegill length-frequency distribution at the end of a 3-month experiment conducted in eight 0.4-ha ponds. Ponds were either high (712 ± 54.3 g m−2; hatched bars) or low (109 ± 21.0 g m−2; open bars) vegetation biomass treatments. Bluegill were 30–50 mm in TL at the beginning of the experiment. Bars indicate 1 SE.
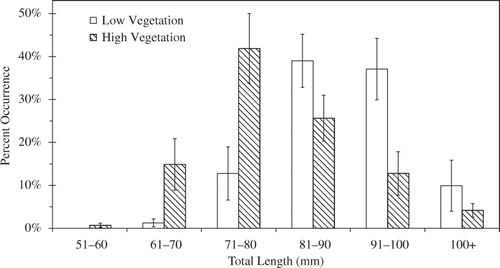
Bluegill stomach fullness (mg dry prey biomass/g wet predator biomass) was similar between vegetation treatments (F 1,6 = 1.48, p = 0.27) and dates (F 1,6 = 0.00, p = 0.98), and there was no treatment by date interaction (F 1,6 = 0.13, p = 0.73; ). However, diet composition varied with treatment (). Across all taxa, the effects of treatment varied by date (MANOVA Wilk's λ = 0.18, F 10,175 = 16.37, p < 0.01); however, only the treatment effect was significant when the taxa were individually analyzed (all ANOVA interactions F 1,12 ≤ 2.52, p ≥ 0.14). Small effects for some of the diet categories combined to jointly indicate significant interaction in the more powerful MANOVA analysis; in this case, the main effects from ANOVAs can still be interpreted outside of the interactive MANOVA effects (Rencher Citation1995). Fish from the low vegetation treatment ate a greater proportion by weight of chironomids (F 1,12 ≥ 9.28, p = 0.01) and a lower proportion of gastropods (F 1,12 ≥ 5.10, p = 0.04) and odonates (F 1,12 ≥ 5.18, p = 0.04) than fish from the high vegetation treatment (). Fish also ate more coleopterans in August than in September (F 1,12 ≥ 14.79, p < 0.01), but this difference was consistent across vegetation treatments (treatment effect F 1,12 ≥ 0.27, p = 0.61). For both treatments, zooplankton made up only 6–20% of the diet by weight (). Excluding benthic ostracods, zooplankton consumption was ≤1% by weight in both treatments.
Figure 3. Mean stomach fullness (mg dry prey biomass/g wet bluegill mass) of bluegill from high (712 ± 54.3 g m−2; hatched bars) or low (109 ± 21.0 g m−2; open bars) vegetation treatments over a 3-month experiment conducted in 0.4-ha ponds at the Sam Parr Biological Station to test the effects of vegetation biomass on growth of juvenile bluegill. Bars indicate 1 SE.
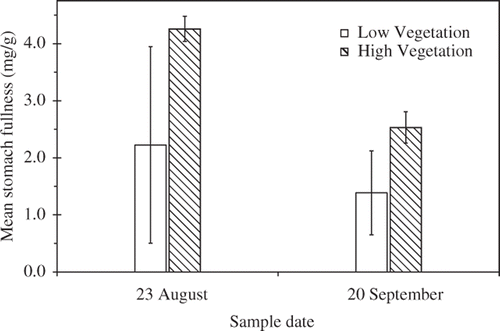
Figure 4. Proportion by weight and number of different prey taxa consumed by juvenile bluegill during a 3-month experiment conducted in 0.4-ha ponds to test the effects of vegetation biomass on growth of bluegill. Ponds were high (n = 4; 712 ± 54.3 g m−2; hatched bars) or low (n = 4; 109 ± 21.0 g m−2; open bars) vegetation biomass treatments. Taxa that always had proportion by weight or number <2% of the total were pooled with unidentifiable taxa as ‘other’. Taxa marked ‘NA’ were grouped as ‘other’ for the analysis. Bars indicate 1 SE.
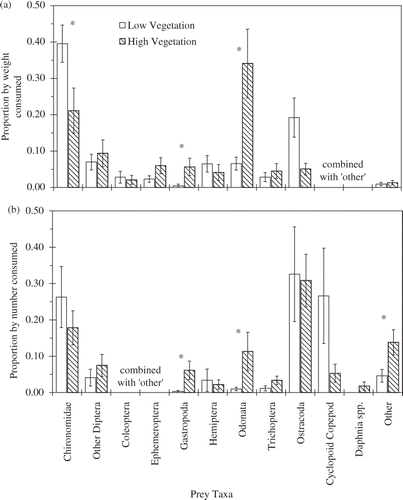
Diets expressed as percent by number also varied between treatments (Wilk's λ = 0.075, treatment effect F 10,125 = 6.25, p = 0.03). Bluegill from the low vegetation treatment ate fewer gastropods (F 1,12 = 4.43, p = 0.03), odonates (F 1,12 = 6.40, p = 0.03) and ‘other’ taxa (F 1,12 = 5.81, p = 0.03) than the fish in the high vegetation treatment (). Despite their small size, zooplankton still accounted for only 38–59% of fish diets by number (). If benthic ostracods are excluded, zooplankton consumption was only 7–27% by number.
Discussion
There is much disagreement in the literature about how vegetation density affects foraging return and growth of juvenile bluegill. Several studies have found reduced foraging return for bluegill when they forage in structurally complex habitats (Mittelbach Citation1981; Gotceitas Citation1990a; Pothoven et al. Citation1999), whereas others have found that bluegill growth was unaffected or even increased when foraging in complex environments (Savino et al. Citation1992; Hayse and Wissing Citation1996; Richardson et al. Citation1998). Much of this is confounded by differing levels of pelagic predation risk; so, it has been difficult to determine if changes in growth were related to predator avoidance behavior or vegetation-related effects on prey choice or foraging efficiency. Our study is the first to examine the effect of vegetation density on diet and growth of bluegill in the absence of predation risk outside of the laboratory environment. Increased vegetation led to a greater use of macrophyte-dwelling insect prey (gastropods and odonates) and reduced use of benthic prey (chironomids). These changes in prey consumption likely caused the observed decrease in growth rate of juvenile bluegill in high vegetation environments.
The difference in growth rates of bluegill in low and high vegetation density could be caused by several mechanisms. First, the high vegetation treatment may have had lower prey abundance, and bluegill were unable to find sufficient food. Prey abundance data were not collected during the experiment; however, this hypothesis does not appear likely as fish in both treatments appeared to find and ingest ample food resources. Mean stomach fullness (1.4–4.3 mg dry prey/g wet predator) was higher than those reported by Booth (Citation1990) for bluegill feeding in Lake Opinicon (0–1.4 mg dry prey/g wet bluegill, depending on the time of the day). Stomach fullness was also similar to fish fed a 2.2% daily ration (g wet prey/g wet bluegill; Gerking Citation1955), the midpoint of the range of daily rations (1–4%) reported for this species (Gerking Citation1954; Windell Citation1966; Keast and Welsh Citation1968; Whitledge and Hayward Citation2000). Further, invertebrate abundance typically increases with increased vegetation density (Gerking Citation1957; Savino et al. Citation1992; Gotceitas and Colgan Citation1990), suggesting that insufficient food is not the primary mechanism.
Second, differences in energetic value or digestibility of the prey types eaten by fish in the two treatments may have differed. Bluegill in the high vegetation treatment ingested more gastropods and odonates and less chironomids than did the fish in low vegetation biomass treatment. However, all the commonly eaten prey types have similar energy densities (Cummins and Wuycheck Citation1971). No published information exists on the digestibility of these prey types to bluegill, but rainbow trout are able to assimilate similar amounts of energy from all three prey types (Groot Citation1995). Therefore, it seems unlikely that energetic or digestibility differences among prey types could account for the difference in growth rate of bluegill between treatments.
Finally, difference in search efficiency or handling costs could account for the differences in growth rates between treatments. Bluegill that forage in vegetation expend more energy searching for prey (Gotceitas Citation1990a, Citation1990b). Reduced search and handling efficiency has also been demonstrated by laboratory studies for roach (Rutilusrutilus; Persson Citation1991) and largemouth bass (Micropterus salmoides; Anderson Citation1984) feeding in dense vegetation. Additionally, gastropods (a prey type heavily used by fish in the high vegetation treatment) require longer handling times than other prey such as chironomids (Mittelbach Citation1984; Osenberg et al. Citation1992). Therefore, while fish from both treatments had similar stomach fullness (suggesting similar consumption rates), it is likely that bluegill in the high vegetation treatment expended more energy to ingest their food, leading to reduced growth. Regardless of the mechanism, our results demonstrate that increased vegetation densities reduced bluegill growth rates even in the absence of pelagic predation risk.
Diets of bluegill indicated that they fed mostly in littoral or benthic habitats. Percent of pelagic zooplankton in the diet, excluding benthic ostracods, was <1% by weight in both treatments. Although pelagic zooplankton are often considered the optimal prey type for bluegill, several studies have demonstrated that bluegill eat a high proportion of invertebrates (Mittelbach Citation1981; Schneider Citation1999; Olson et al. Citation2003a), presumably because they use littoral habitat to avoid predation risk associated with pelagic habitat (Mittelbach Citation1981; Ostrand et al. Citation2004). Similar predator-mediated habitat and diet shifts have been found for other fish species (Diehl and Eklov Citation1995; Persson and Eklov Citation1995). Because piscivorous fish were not present in the ponds, it was surprising that bluegill foraged so heavily in the vegetated habitat. Shoup et al. (Citation2003) found that bluegill in the laboratory spent 40–80% of their time in artificial macrophytes even in the absence of a predator and when food was only available in the open water. Either bluegill cannot accurately assess predation risk or some other mechanism causes bluegill to forage in vegetated habitat. The propensity of bluegill to forage in vegetated habitat could be genetically linked (Lister and Neff Citation2006) or related to phenotype (Layzer and Clady Citation1987; Ehlinger and Wilson Citation1988; Chipps et al. Citation2004). In both cases, fish would not be expected to alter their habitat use in response to the absence of predators over short time scales. Bluegill may also select habitat due to temperature preference rather than foraging return (Wildhaber Citation2001), highlighting the potential for mechanisms other than predation risk. Previous laboratory (Persson Citation1991) and enclosure (Diehl and Eklov Citation1995) studies have found that Eurasian perch (Perca fluviatilis) and roach occasionally chose to feed in vegetation rather than open water even when no predator was present. Additional research is needed to determine the pervasiveness of these behaviors and the underlying mechanisms.
Interactions among structural complexity, fish habitat selection and predator–prey interactions are complex, making recommendations about habitat management for optimizing fish densities and size structure difficult. By separating the role of each of these factors, it is possible to better understand the importance of each in the overall system response. Our results demonstrate that bluegill chose to forage in littoral habitat even in the absence of open-water predation risk, leading to reduced growth rate at higher vegetation densities. Managing for moderate vegetation density or cutting deep channels through vegetation (Olson et al. Citation1998) may be useful for maximizing bluegill growth, even when piscivore biomass is not high enough to constitute a significant predation risk.
Acknowledgments
The authors thank C. Kolar, S. Callahan, and the staff of the Kaskaskia and Sam Parr Biological Stations for their help in collecting and analyzing data. Funding for this project was provided in part by the Federal Aid in Sport Fish Restoration Act, Project F-128-R and F-135-R through the Illinois Department of Natural Resources (IDNR), Division of Fisheries. S. Pallo, L. Dunham, J. Ferencak, and M. Conlin coordinated activities with the IDNR.
References
- Abdel-Tawwab , M . 2005 . The effect of artificial vegetation density on growth and growth related parameters of Nile tilapia, Oreochromis niloticus (L.) Fry . Turkish Journal of Fisheries and Aquatic Sciences , 2 ( 5 ) : 63 – 68 .
- Abrahams , MV . 1994 . Risk of predation and its influence on the relative competitive abilities of two species of freshwater fishes . Canadian Journal of Fisheries and Aquatic Sciences , 51 ( 7 ) : 1629 – 1633 .
- Anderson , O . 1984 . Optimal foraging by largemouth bass in structured environments . Ecology , 65 ( 3 ) : 851 – 861 .
- Barwick , DH . 2004 . Species richness and centrarchid abundance in littoral habitats of three southern US reservoirs . North American Journal of Fisheries Management , 24 ( 1 ) : 76 – 81 .
- Benke , AC , Huryn , AD , Smock , LA and Wallace , JB . 1999 . Length-mass relationships for freshwater macroinvertebrates in North America with particular reference to the southeastern United States . Journal of the North American Benthological Society , 18 ( 3 ) : 308 – 343 .
- Booth , DJ . 1990 . Effect of water temperature on stomach evacuation rates, and estimation of daily food intake of bluegill sunfish (Lepomis macrochirus Rafinesque) . Canadian Journal of Zoology , 68 ( 3 ) : 591 – 595 .
- Chipps , SR , Dunbar , JA and Wahl , DH . 2004 . Phenotypic variation and vulnerability to predation in juvenile bluegill sunfish (Lepomis macrochirus) . Oecologia , 138 ( 1 ) : 32 – 38 .
- Crowder , LB and Cooper , WE . 1982 . Habitat structural complexity and the interaction between bluegills and their prey . Ecology , 6 ( 63 ) : 1802 – 1813 .
- Culver , DA , Boucherle , MM , Bean , DJ and Fletcher , JW . 1985 . Biomass of freshwater crustacean zooplankton from length-weight regressions . Canadian Journal of Fisheries and Aquatic Sciences , 42 ( 8 ) : 1380 – 1390 .
- Cummins , KW and Wuycheck , JC . 1971 . Caloric equivalents for investigations in ecological energetics , 158 Stuttgart (Germany) : International Association of Theoretical and Applied Limnology. Communication No. 18; .
- Dibble , ED and Harrel , SL . 1997 . Largemouth bass diets in two aquatic plant communities . Journal of Aquatic Plant Management , 35 : 74 – 78 .
- Diehl , S and Eklov , P . 1995 . Effects of piscivore-mediated habitat use on resources, diet, and growth of perch . Ecology , 76 ( 6 ) : 1712 – 1726 .
- Dumont , HJ , Van de Velde , I and Dumont , S . 1975 . The dry weight estimate of biomass in a selection of cladocera, copepoda and rotifera from the plankton, periphyton and benthos of continental waters . Oecologia , 19 ( 1 ) : 75 – 97 .
- Ehlinger , TJ and Wilson , DS . 1988 . Complex foraging polymorphism in bluegill sunfish . Proceedings of the National Academy of Sciences of the United States of America , 85 ( 6 ) : 1878 – 1882 .
- Gerking , SD . 1954 . The food turnover of a bluegill population . Ecology , 35 ( 4 ) : 490 – 498 .
- Gerking , SD . 1955 . Influence of rate of feeding on body composition and protein metabolism of bluegill sunfish . Physiological Zoology , 28 ( 4 ) : 267 – 282 .
- Gerking , SD . 1957 . A method of sampling the littoral macrofauna and its application . Ecology , 38 ( 2 ) : 219 – 226 .
- Gotceitas , V . 1990a . Foraging and predator avoidance: a test of a patch choice model with juvenile bluegill sunfish . Oecologia , 83 ( 3 ) : 346 – 351 .
- Gotceitas , V . 1990b . Variation in plant stem density and its effects on foraging success of juvenile bluegill sunfish . Environmental Biology of Fishes , 27 ( 1 ) : 63 – 70 .
- Gotceitas , V and Colgan , P . 1987 . Selection between densities of artificial vegetation by young bluegills avoiding predation . Transactions of the American Fisheries Society , 116 ( 1 ) : 40 – 49 .
- Gotceitas , V and Colgan , P . 1990 . The effects of prey availability and predation risk on habitat selection by juvenile bluegill sunfish . Copeia , 1990 ( 2 ) : 409 – 417 .
- Groot , C . 1995 . Physiological ecology of Pacific salmon , 384 Vancouver (BC) : UBC Press .
- Hackney , PA . 1979 . “ Influence of piscivorous fish on fish community structure of ponds ” . In Predator–prey systems in fisheries management , Edited by: Clepper , H . 111 – 121 . Washington (DC) : Sport Fishing Institute .
- Hayse , JW and Wissing , TE . 1996 . Effects of stem density of artificial vegetation on abundance and growth of age-0 bluegills and predation by largemouth bass . Transactions of the American Fisheries Society , 125 ( 3 ) : 422 – 433 .
- Keast , A and Welsh , L . 1968 . Daily feeding periodicities, food uptake rates, and dietary changes with hour of day in some lake fishes . Journal of the Fisheries Research Board of Canada , 25 ( 6 ) : 1133 – 1144 .
- Layzer , JB and Clady , MD . 1987 . Phenotypic variation of young-of-year bluegills (Lepomis macrochirus) among microhabitats . Copeia , 1987 ( 3 ) : 702 – 707 .
- Lister , JS and Neff , BD . 2006 . Paternal genetic effects on foraging decision-making under the risk of predation . Ethology , 112 ( 10 ) : 963 – 970 .
- Magnhagen , C . 1988 . Predation risk and foraging in juvenile pink (Oncorhynchus gorbuscha) and chum salmon (O . keta). Canadian Journal of fisheries and Aquatic Sciences , 45 ( 4 ) : 592 – 596 .
- Mittelbach , GG . 1981 . Foraging efficiency and body size: a study of optimal diet and habitat use by bluegills . Ecology , 62 ( 5 ) : 1370 – 1386 .
- Mittelbach , GG . 1984 . Predation and resource partitioning in two sunfishes (Centrarchidae) . Ecology , 65 ( 2 ) : 499 – 513 .
- Olson , MH , Carpenter , SR , Cunningham , P , Gafny , S , Herwig , BR , Nibbelink , NP , Pellett , T , Storlie , C , Trebitz , AS and Wilson , KA . 1998 . Managing macrophytes to improve fish growth: a multi-lake experiment . Fisheries , 23 ( 2 ) : 6 – 12 .
- Olson , MH , Paukert , CP , Willis , DW and Klammer , JA . 2003a . Prey selection and diets of bluegill Lepomis macrochirus with differing population characteristics in two Nebraska natural lakes . Fisheries Management and Ecology , 10 ( 1 ) : 31 – 40 .
- Olson , MH , Young , BP and Blinkoff , KD . 2003b . Mechanisms underlying habitat use of juvenile largemouth bass and smallmouth bass . Transactions of the American Fisheries Society , 132 ( 2 ) : 398 – 405 .
- Osenberg , CW , Mittelbach , GG and Wainwright , PC . 1992 . Two-stage life histories in fish: the interaction between juvenile competition and adult performance . Ecology , 73 ( 1 ) : 255 – 267 .
- Ostrand , KG , Braeutigam , BJ and Wahl , DH . 2004 . Consequences of vegetation density and prey species on spotted gar foraging . Transactions of the American Fisheries Society , 133 ( 3 ) : 794 – 800 .
- Persson , L . 1991 . Behavioral response to predators reverses the outcome of competition between prey species . Behavioral Ecology and Sociobiology , 28 ( 2 ) : 101 – 105 .
- Persson , L and Eklov , P . 1995 . Prey refuges affecting interactions between piscivorous perch and juvenile perch and roach . Ecology , 76 ( 1 ) : 70 – 81 .
- Pothoven , SA , Vondracek , B and Pereira , DL . 1999 . Effects of vegetation removal on bluegill and largemouth bass in two Minnesota lakes . North American Journal of Fisheries Management , 19 ( 3 ) : 748 – 757 .
- Rencher , AC . 1995 . Methods of multivariate analysis , 627 New York (NY) : John Wiley and Sons, Inc .
- Richardson , WB , Zigler , SJ and Dewey , MR . 1998 . Bioenergetic relations in submerged aquatic vegetation: an experimental test of prey use by juvenile bluegills . Ecology of Freshwater Fishes , 7 ( 1 ) : 1 – 12 .
- Robertson , CR , Zeug , SC and Winemiller , KO . 2008 . Associations between hydrological connectivity and resource partitioning among sympatric gar species (Lepisosteidae) in a Texas river and associated oxbows . Ecology of Freshwater Fish , 17 ( 1 ) : 119 – 129 .
- Sample , BE , Cooper , RJ , Greer , RD and Whitmore , RC . 1993 . Estimation of insect biomass by length and width . American Midland Naturalist , 129 ( 2 ) : 234 – 240 .
- Santucci , VJ and Wahl , DH . 2003 . The effects of growth, predation, and first-winter mortality on recruitment of bluegill cohorts . Transactions of the American Fisheries Society , 132 ( 2 ) : 346 – 360 .
- SAS Institute . 2004 . SAS/STAT user's guide. ver. 9.1.3 , 5180 Cary (NC) : SAS Institute .
- Savino , JF and Stein , RA . 1982 . Predator-prey interaction between largemouth bass and bluegills as influenced by simulated, submersed vegetation . Transactions of the American Fisheries Society , 111 ( 3 ) : 255 – 266 .
- Savino , JF and Stein , RA . 1989 . Behavioural interactions between fish predators and their prey: effects of plant density . Animal Behavior , 37 ( 2 ) : 311 – 321 .
- Savino , JF , Marschall , EA and Stein , RA . 1992 . Bluegill growth as modified by plant density: an exploration of underlying mechanisms . Oecologia , 89 ( 2 ) : 153 – 160 .
- Schneider , JC . 1999 . Dynamics of quality bluegill populations in two Michigan lakes with dense vegetation . North American Journal of Fisheries Management , 19 ( 1 ) : 97 – 109 .
- Schramm Jr , HL and Zale , AV . 1985 . Effects of cover and prey size on preference of juvenile largemouth bass for blue tilapias and bluegills in tanks . Transactions of the American Fisheries Society , 114 ( 5 ) : 725 – 731 .
- Shoup , DE , Callahan , SP , Wahl , DH and Pierce , CL . 2007 . Size-specific growth of bluegill, largemouth bass and channel catfish in relation to prey availability and limnological variables . Journal of Fish Biology , 70 ( 1 ) : 21 – 34 .
- Shoup , DE , Carlson , RE and Heath , RT . 2003 . Effects of predation risk and foraging return on the diel use of vegetated habitat by two size-classes of bluegills . Transactions of the American Fisheries Society , 132 ( 3 ) : 590 – 597 .
- Shoup , DE and Wahl , DH . 2009 . The effect of turbidity on prey selection by piscivorous largemouth bass . Transactions of the American Fisheries Society , 138 ( 5 ) : 1018 – 1027 .
- Smock , LA . 1980 . Relationships between body size and biomass of aquatic insects . Freshwater Biology , 10 ( 4 ) : 375 – 383 .
- Wahl , DH and Stein , RA . 1988 . Selective predation by three esocids: the role of prey behavior and morphology . Transactions of the American Fisheries Society , 117 ( 2 ) : 142 – 151 .
- Wellenreuther , M , Barrett , PT and Clements , KD . 2007 . Ecological diversification in habitat use by subtidal triplefin fishes (Tripterygiidae) . Marine Ecology Progress Series , 330 : 235 – 246 .
- Werner , EE , Gilliam , JF , Hall , DJ and Mittelbach , GG . 1983a . An experimental test of the effects of predation risk on habitat use in fish . Ecology , 64 ( 6 ) : 1540 – 1548 .
- Werner , EE , Mittelbach , GG , Hall , DJ and Gilliam , JF . 1983b . Experimental tests of optimal habitat use in fish: the role of relative habitat profitability . Ecology , 64 ( 6 ) : 1525 – 1539 .
- Whitledge , GW and Hayward , RS . 2000 . Determining sampling date interval for precise in situ estimates of cumulative food consumption by fishes . Canadian Journal of Fisheries and Aquatic Sciences , 57 ( 6 ) : 1131 – 1138 .
- Wildhaber , ML . 2001 . The trade-off between food and temperature in the habitat choice of bluegill sunfish . Journal of Fish biology , 58 ( 5 ) : 1476 – 1478 .
- Wiley , MJ and Wike , LD . 1986 . Energy balances of diploid, triploid, and hybrid grass carp . Transactions of the American Fisheries Society , 115 ( 6 ) : 853 – 863 .
- Windell , JT . 1966 . Rate of digestion in the bluegill sunfish . Investigations of Indiana Lakes and Streams , 7 ( 6 ) : 185 – 214 .
- Wright , SP . 1998 . Multivariate analysis using the MIXED procedure , 1238 – 1232 . In : Proceedings of the annual SAS users group international Conference 23, March 22–25, 1998, Nashville, Tennessee .