Abstract
Isoetids are submersed aquatic plants that thrive in soft water, oligotrophic bodies of water and possess small, stiff rosettes and extensive root systems. These roots absorb carbon dioxide and release oxygen, altering the biogeochemistry of sediment. Eriocaulon aquaticum and Lobelia dortmanna are representative isoetids. While Eleocharis acicularis and Utricularia resupinata do not strictly qualify as isoetids, they are commonly found anchored in or attached to sediment populated with isoetids. In a greenhouse experiment, the four macrophytes were planted in two contrasting sediment types to determine how the different species modify sediment redox potential, carbon dioxide concentration, and porewater pH. Eleocharis acicularis and U. resupinata oxidized both sediment types and acidified the porewater more than or equally as extensively as the isoetids (p < 0.05). Eleocharis acicularis was the only species that significantly reduced porewater carbon dioxide (p < 0.05). In the field study, sediment cores were collected over bare sediment and over each of the four species concurrently with redox and pH measurements. Sediment cores associated with all four aquatic plants had significantly higher redox potentials compared to bare sediment (p < 0.05). However, the redox values measured near E. acicularis and U. resupinata were generally lower than those taken near the isoetids. The field sediment oxidation data may be explained by the significantly lower biomass of U. resupinata and E. acicularis compared to the isoetids in the field study (p < 0.05). These results show that E. acicularis and U. resupinata are capable of isoetid-like sediment oxidation under greenhouse and field conditions.
Introduction
Isoetids are submersed aquatic plants with small, stiff rosettes, and extensive root systems (Hutchinson Citation1975; Richardson et al. Citation1984; Raven et al. Citation1988). These roots absorb carbon dioxide (CO2; Wium-Andersen 1971; Pedersen and Sand-Jensen Citation1992; Winkel and Borum Citation2009) and release oxygen (O2; Wium-Andersen and Andersen 1972; Sand-Jensen et al. Citation1982). Macrophytes with isoetid growth forms thrive in soft water, oligotrophic systems and the high root to shoot ratios of these species are indicative of their low-nutrient habitats (Hutchinson Citation1975; Chapin Citation1980).
The gas exchange by isoetid roots alters the biogeochemistry of sediment. The release of oxygen produced during photosynthesis increases sediment redox potential, stimulating aerobic decomposition of organic matter (Sand-Jensen et al. Citation1982), high rates of nitrification (Christensen and Sørensen Citation1986), oxidation of iron(II) (Fe2+) to iron(III) (Fe3+) (Wium-Andersen and Andersen Citation1972; Christensen et al. Citation1998), and suppression of sulfate reduction (Holmer et al. Citation1998). These processes may contribute to the declines of porewater pH typically observed in the presence of isoetids (Sand-Jensen et al. Citation1982; Risgaard-Petersen and Jensen Citation1997).
Eriocaulon aquaticum (Hill) Druce is a submersed or emersed perennial of the family Eriocaulaceae (Crow and Hellquist Citation2000a). It has a characteristic isoetid form with leaves in a basal rosette and an extensive root system (Hutchinson Citation1975; Crow and Hellquist Citation2000a). Lobelia dortmanna L. of the Campanulaceae family is the only aquatic member of its genus (Crow and Hellquist Citation2000b). As an isoetid (Hutchinson Citation1975), it has a basal rosette of lacunate leaves and an extensive root system capable of gas exchange along its entire length (Wium-Andersen and Andersen Citation1972; Pedersen et al. Citation1995).
Eleocharis acicularis (L.) Roemer & Schultes, a member of the Cyperaceae family, is a terrestrial or submersed herbaceous perennial (Rothrock and Wagner Citation1975). The inclusion of E. acicularis with the isoetids is indeterminate. While some individuals have identified it as an isoetid (Hutchinson Citation1975), others have deviated from this classification (Raven et al. Citation1988). Eleocharis acicularis can grow in acidic sediment and water (Rothrock and Wagner Citation1975; Crow and Hellquist Citation2000a) in littoral zones populated with isoetids (Hutchinson Citation1975). These macrophytes have short, lacunate culms (Rothrock and Wagner Citation1975; Rhoads and Block Citation2007), and moderately extensive root systems that superficially resemble the isoetid growth form.
Utricularia resupinata B.D. Greene ex Bigelow, a submersed rootless plant of the Lentibulariaceae family, comprises modified stems (Crow and Hellquist Citation2000a) that can penetrate and anchor in muddy substrates (Gleason and Cronquist Citation1991; Rhoads and Block Citation2007). Utricularia resupinata is also capable of living in sediment types (Borman et al. Citation2009) and acidic water (Brouwer et al. Citation2002) containing isoetids. Its habitat, combined with the small stature of its stems, has led some researchers to describe U. resupinata as an isoetid (Borman et al. Citation2009).
Without roots or a rosette of leaves, U. resupinata does not strictly qualify as an isoetid and E. acicularis with its culms and small basal sheath of leaves also does not meet this growth form's criteria. Although U. resupinata and E. acicularis may not match the morphological definition of isoetid, their similarities in habitat and appearance to isoetids are strongly evident. The sediment modification performed by isoetids contributes to the plants’ oligotrophic environments and U. resupinata and E. acicularis may be of equal ecological importance in this regard.
A greenhouse experiment and field study were performed to determine if the sediment-modifying capabilities of E. acicularis and U. resupinata rival those of the accepted isoetids, E. aquaticum and L. dortmanna. Personal observations of iron plaques on E. acicularis’ roots suggested that this species is capable of CO2 uptake from and O2 release to sediment, perhaps to the same degree as isoetids. It was hypothesized that without a root system, U. resupinata would not oxidize the sediment and would take up CO2 less extensively than the isoetids, particularly at greater substrate depths. Similarly, it was hypothesized that the oxidation of sediment would be concomitant with reductions in sediment porewater pH, specifically in treatments containing isoetids or E. acicularis.
Methods
Greenhouse experiment
Surface sediment was collected at 3 m water depth by an Ekman dredge in Eldon Bay, Raquette Lake (Hamilton County, NY, USA; 43°84′N, 74°62′W) and by a SCUBA diver in Big Moose Lake (Herkimer County, NY, USA; 43°83′N, 74°85′W) in the Adirondack Mountains. Both sediment types were stored at 5°C until the start of the experiment, at which time sediment was sieved through 0.3-cm wire mesh and homogenized. Five replicate subsamples of each sediment type were dried at 105°C to determine bulk density and combusted at 540°C to determine loss on ignition (LOI). Eriocaulon aquaticum, L. dortmanna, and U. resupinata specimens were collected from Big Moose Lake, while E. acicularis was gathered from Seventh Lake (Herkimer County, NY, USA; 43°42′N, 74°54′W).
The experiment was performed in Lebanon Valley College's west greenhouse (Annville, PA, USA) between 3 June 2009 and 2 October 2009. There were 10 treatments with eight replicates, with each of eight 76-L aquaria containing one replicate per treatment for a total of 10 pots per aquarium, in a randomized block design: U. resupinata, E. aquaticum, E. acicularis, and L. dortmanna were planted in two sediment types and both sediment types also had a bare sediment treatment. Plants were randomly assigned to one of 80 pots, each with a surface area of 86.5 cm2 and volume of approximately 530 cm3, and two individuals of L. dortmanna, E. aquaticum, and E. acicularis and two bundles of U. resupinata were planted in opposite corners of their assigned pots. The sediment level was 23 cm below the water surface. The pots were rotated weekly in their aquaria.
The pH and temperature of the water in each aquarium were regularly monitored with an Oakton® Waterproof pH/CON 10 meter (Oakton® Instruments, Vernon Hills, IL, USA). Small amounts of 0.4N hydrochloric acid (HCl) and 0.4N sodium hydroxide (NaOH) were added to the aquaria as necessary to maintain a water column pH of approximately 5.0 (±0.5). The reverse osmosis water (conductivity of 21 µS cm−1) in the aquaria was replaced as necessary to limit algal growth.
Sediment redox potential values were measured 122 days into the experiment at 2, 4, and 6 cm depths using an MI-800E micro-redox electrode and an MI-402 Ag/AgCl reference electrode (Microelectrodes Inc.®, Bedford, NH, USA) with a Thermo Scientific Orion™ 5Star meter (Thermo Fisher Scientific, Waltham, MA, USA). Measurements were taken 1 cm from the base of an original plant in each pot after it was removed from its aquarium. A comparative approach was used to negate a bias created by the introduction of oxygen into the sediment during the redox potential readings.
Equilibrators were used to sample porewater for pH and [CO2]. At the start of the experiment, PVC sections matching the size of the equilibrators were inserted into the sediment as placeholders, minimizing the disruption of plant growth upon the introduction of the equilibrators to the sediment. Equilibrators were constructed using 6 cm long (inner diameter 1.2 cm) PVC pipe with holes drilled through the side and Versapore® 0.2 µm membrane attached to the holes and one PVC end using a latex sealant. A PVC cap sealed the other end of the pipe. On day 50, equilibrators were placed in the sediment.
Equilibrators were removed beginning on day 153. The pH and CO2 levels of the water contained in the equilibrators were measured within a few minutes of removal. Porewater aliquots were injected into 2% nitric acid that was continuously sparged with nitrogen gas to carry the CO2 to an ADC-225-MK3 infrared gas analyzer (Analytical Development Company, Hoddesdon, UK) and a Hewlett-Packard 3390A Integrator (Hewlett-Packard Company, Palo Alto, CA, USA) to measure dissolved inorganic carbon. After measurement of each sample's pH with a Thermo Orion™ model 91-03 combination pH semi-micro electrode (Thermo Fisher Scientific, Waltham, MA, USA) and Thermo Scientific Orion™ 5Star meter, dissolved CO2 concentrations of the porewater were calculated using published equilibrium constants for carbonic acid (Stumm and Morgan Citation1981).
On day 183, plants were removed from each pot, cleaned, and their final dry biomasses determined by drying them to a constant weight at 80°C.
Field study
To compare the results of the greenhouse experiment to what naturally exists in the field, a snorkeler collected 40 in situ sediment cores, each with a surface area of 19.6 cm2, from Limekiln Lake (Hamilton County, NY, USA; 43°43′N, 74°48′W) on 21 July 2010. The sediment cores were acquired by hand using an acrylic corer. Eight replicate sediment cores each were collected over bare sediment, U. resupinata, E. aquaticum, E. acicularis, and L. dortmanna to sediment depths of 10–12 cm. Because of the natural distribution of the plants, cores were collected at 0.5 m water depth for U. resupinata, E. aquaticum, and L. dortmanna and at 1.2 m in depth for E. acicularis. Bare sediment was collected 20 cm from the closest observed macrophyte, with six bare sediment cores from 0.5 m in depth and two cores from 1.2 m in depth. The samples were kept submersed in buckets and immediately transported to a field cabin where they could be processed.
The redox potential of the sediment was measured with an MI-800E micro-redox electrode and an MI-403 Ag/AgCl reference electrode with an Orion™ model 350 (PerpHecT LogR) meter (Thermo Fisher Scientific, Waltham, MA, USA). Measurements were taken at 2, 4, and 6 cm depths after sediment removal from the lake. A comparative approach was used to negate the introduction of oxygen into the sediment during this process. The pH of the sediment was also taken at approximately 4 cm in depth with a 9203BN semi-micro combination pH electrode (Thermo Fisher Scientific, Waltham, MA, USA).
Entire plants were removed from each core, cleaned, and divided into their aboveground and belowground components. Their aboveground, belowground, and total biomasses were determined by drying the plants to a constant weight at 80°C. The remaining sediment was refrigerated and brought back to Lebanon Valley College (Annville, PA, USA) to determine sediment bulk density (after drying sediment at 105°C) and LOI (after combusting sediment at 540°C).
Statistical analyses
Initial tests using the Shapiro–Wilk test for normality, normal probability plots of residuals, and the Levene or Bartlett test for equal variances were performed on the data. Greenhouse carbon dioxide, 6 cm redox potential, and log-transformed final total dry biomass data met all analysis of variance (ANOVA) assumptions and were analyzed with a two-way ANOVA and post hoc Tukey's Honestly Significant Difference (HSD) tests. Greenhouse data for sediment redox potentials at 2 and 4 cm, Box–Cox transformed (λ = −1.4) pH data, sediment bulk density, and sediment LOI were heteroskedastic. Field data for sediment redox potentials at 2, 4, and 6 cm; pH; sediment bulk density; sediment LOI; and total plant biomass were heteroskedastic, while belowground biomass data were non-normal and heteroskedastic. All heteroskedastic data sets were analyzed with two-way analyses of deviance (ANODEV, a generalized linear model equivalent of the ANOVA that permits distributions other than the normal) adjusted with a type HC3 heteroskedasticity-corrected coefficient covariance matrix (HCCCM) recommended for small sample sizes (Long and Ervin Citation2000) and pairwise post hoc max-t tests using a type HC3 HCCCM (Herberich et al. Citation2010) with Holm–Bonferroni adjustments for multiple comparisons (Holm Citation1979). For all tests, p values less than 0.05 were considered statistically significant. Tests were performed using R software (R Foundation for Statistical Computing, Vienna, Austria).
Results
Greenhouse experiment
A comparison of the two sediment types revealed several differences. The pre-experiment mean percent LOI for Raquette sediment (3.0%) was significantly lower than that for Big Moose (7.6%; p < 0.05). Raquette's pre-experiment mean sediment bulk density (0.869 g dry mass cm−3) was significantly higher than that of Big Moose sediment (0.586 g dry mass cm−3; p < 0.05). While redox potentials did not differ at any depth between the two bare sediment types at the experiment's conclusion (), Big Moose bare sediment had a significantly lower mean porewater pH (5.7) than Raquette sediment (6.4) at that time ().
Figure 1. Mean (n = 8; +SE) redox potentials (mV) at sediment depths of (a) 2, (b) 4, and (c) 6 cm in Big Moose or Raquette substrate in a greenhouse experiment. Eleocharis acicularis (Eleo), U. resupinata (Utr), E. aquaticum (Erio), and L. dortmanna (Lob) were planted in both sediment types, and each sediment type also had a bare sediment treatment. Treatments not sharing letters are significantly different (p < 0.05) within each depth as detected by (a, b) max-t tests or (c) Tukey's HSD test.
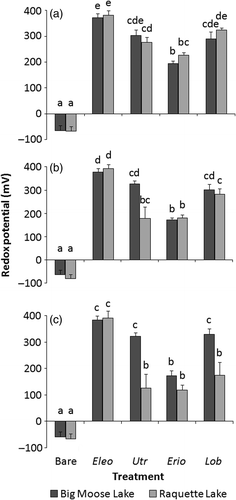
Figure 2. Mean (n = 8; +SE) sediment porewater pH values of samples collected using sediment porewater equilibrators in Big Moose or Raquette substrate in a greenhouse experiment. Eleocharis acicularis (Eleo), U. resupinata (Utr), E. aquaticum (Erio), and L. dortmanna (Lob) were planted in both sediment types, and each sediment type also had a bare sediment treatment. Treatments not sharing letters are significantly different (p < 0.05) as detected by max-t tests.
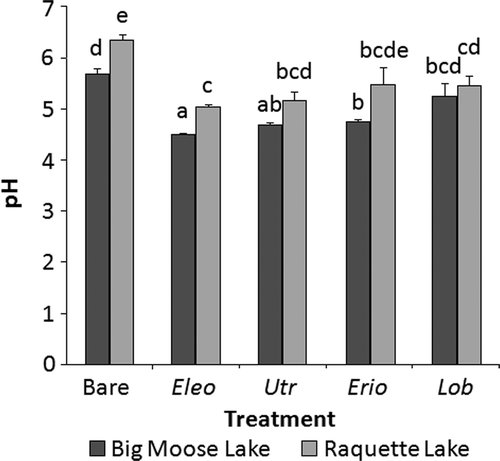
In an evaluation of the plants’ greenhouse sediment oxidation capabilities, the species present in each sediment type affected redox potential at 2 cm (F = 156.6, p < 0.001), 4 cm (F = 108.1, p < 0.001), and 6 cm (F = 76.1, p < 0.001) with all species significantly increasing sediment redox potential compared to bare sediment (). Utricularia resupinata oxidized the substrate to a greater extent than E. aquaticum at all depths in Big Moose sediment and equally as much as L. dortmanna in both sediment types at every depth (). Eleocharis acicularis and L. dortmanna equivalently oxidized Big Moose sediment (). However, E. acicularis oxidized Raquette sediment significantly more than L. dortmanna at 4 and 6 cm (), and both substrates containing E. acicularis possessed greater redox potentials than the E. aquaticum treatments at all three depths ().
At 6 cm (F = 22.4, p < 0.001) sediment type affected the plants’ abilities to oxidize the substrate. Post hoc pairwise comparisons showed that U. resupinata, L. dortmanna, and, to a lesser extent, E. aquaticum exhibited trends of oxidizing Raquette Lake sediment less than they oxidized Big Moose substrate (). Mean redox potentials of Big Moose sediment containing U. resupinata at 4 and 6 cm depths were 82.1% and 155.0% higher than the respective potentials in Raquette sediment () and this disparity was significant at 6 cm (). There was also a significant difference between the mean 6 cm potential of Big Moose sediment (330 mV) and Raquette sediment (176 mV) containing L. dortmanna (). The redox potential of Big Moose sediment containing E. aquaticum was 54 mV above the equivalent Raquette treatment at 6 cm ().
Plant species (F = 56.7, p < 0.001) had a significant effect on porewater pH, which also differed significantly between sediment types (F = 63.2, p < 0.001). All species except L. dortmanna in Big Moose sediment and E. aquaticum in Raquette sediment significantly lowered the porewater pH levels of their substrate (). Eleocharis acicularis generated the lowest mean pH values for both Big Moose (4.5) and Raquette (5.1) sediments () and its Big Moose pH level was significantly lower than the respective measurements for the two isoetids ().
There was no significant CO2 reduction by any species in Raquette Lake sediment (p > 0.05). Only E. acicularis, which reduced Big Moose porewater CO2 by 43.4%, had a significant effect on porewater CO2 concentrations (p < 0.01).
Sediment affected a species’ mean final biomass in only one treatment. Eriocaulon aquaticum's final total dry biomass in Big Moose sediment was 181.8% greater than the equivalent measurement in Raquette sediment, which was significantly lower than the total biomasses for all other species in both sediment types ().
Figure 3. Mean (n = 8; +SE) total biomasses (g dry mass/86.5 cm2 pot surface area) of plants, including above- and belowground biomass, harvested from Big Moose or Raquette sediment in a greenhouse experiment. Eleocharis acicularis (Eleo), U. resupinata (Utr), E. aquaticum (Erio), and L. dortmanna (Lob) were planted in both sediment types. Treatments not sharing letters are significantly different (p < 0.05) as detected by Tukey's HSD test.
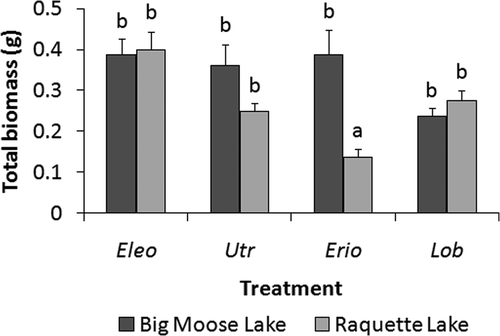
Field study
Sediment cores taken with the four plant species had significantly greater redox values than bare sediment at 2 cm (F = 46.6, p < 0.001), 4 cm (F = 38.3, p < 0.001), and 6 cm (F = 59.3, p < 0.001) in depth (). At 2 cm substrate depth, redox potential was higher in cores associated with U. resupinata, E. aquaticum, and L. dortmanna compared to E. acicularis (). At both 4 and 6 cm, E. aquaticum and L. dortmanna were collected in sediment with significantly higher redox potentials than those containing E. acicularis or U. resupinata ().
Figure 4. Mean (+SE) redox potentials (mV) measured at sediment depths of: (a) 2, (b) 4, and (c) 6 cm in sediment cores collected in a field study at Limekiln Lake. Measurements were taken in cores containing E. acicularis (Eleo), U. resupinata (Utr), E. aquaticum (Erio), or L. dortmanna (Lob). Treatments not sharing letters are significantly different (p < 0.05) within each depth as detected by max-t test (n = 6 for Bare and E. acicularis, n = 8 for others).
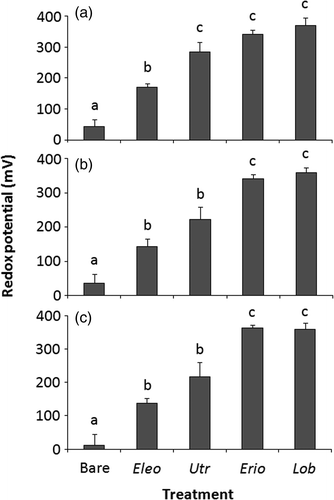
Porewater pH was significantly lower for sediment containing E. acicularis (5.8) than U. resupinata (6.3) and E. aquaticum (6.3; ). Similarly, the mean bulk density of sediment in which E. acicularis was collected was 16.1% lower than that of sediment containing U. resupinata (). The LOI of sediment around E. acicularis (1.3%) was greater than that of sediment in which U. resupinata (0.6%) or L. dortmanna (0.5%) was growing, but all values were generally low (). There were significant differences between species for total plant dry biomass (F = 24.4, p < 0.001), aboveground plant dry biomass (F = 20.3, p < 0.001), and belowground plant dry biomass (F = 19.5, p < 0.001). Utricularia resupinata and E. acicularis possessed mean total, aboveground, and belowground biomasses that were considerably lower than those of E. aquaticum and L. dortmanna (, ).
Figure 5. Mean (+SE) total biomasses (g dry mass/19.6 cm2 sediment core surface area) of plants, including above- and belowground biomass, collected in a field study at Limekiln Lake. Eleocharis acicularis (Eleo), U. resupinata (Utr), E. aquaticum (Erio), and L. dortmanna (Lob) were sampled. Treatments not sharing letters are significantly different (p < 0.05) as detected by Tukey's HSD test (n = 6 for Bare and E. acicularis, n = 8 for others).
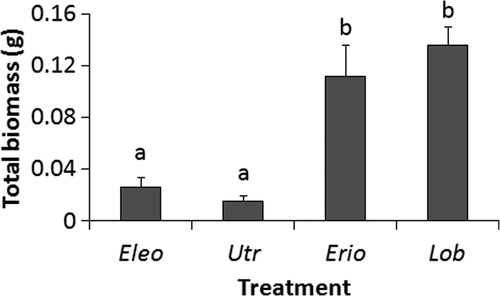
Table 1. Mean (SE) pH values, bulk densities, and losses on ignition of bare sediment and sediment collected near plants in the field study, as well as the mean belowground dry biomasses of collected plant specimens per core with 19.6 cm2 surface area.
Discussion
In the greenhouse experiment, all species demonstrated an ability to oxidize sediment at every depth, contradicting the initial expectation for U. resupinata. Despite lacking roots, U. resupinata oxidized the sediment to the same degree as or more than the isoetid E. aquaticum and equally as extensively as the other isoetid, L. dortmanna. Since U. resupinata's greenhouse total biomasses were not significantly different from L. dortmanna's in Big Moose or Raquette sediment nor from E. aquaticum's in Big Moose substrate, it is unlikely that biomass disparities played a role in the greenhouse redox results. Although belowground biomasses were not determined for the greenhouse experiment, the Limekiln field data suggest that the isoetids possess greater belowground biomasses than U. resupinata. If this were the case in the greenhouse experiment, it would render U. resupinata's ability to oxidize the sediment even more remarkable.
The Limekiln Lake field study produced different, although not necessarily contradictory, results. Sediment cores associated with the four macrophytes, including the rootless U. resupinata, had elevated redox measurements compared to bare sediment. Field potentials measured at 4 and 6 cm depths near U. resupinata were significantly lower than those near E. aquaticum or L. dortmanna but U. resupinata's apparent ability to oxidize the sediment was higher than anticipated. Both the greenhouse and field results suggest that U. resupinata's modified stems penetrated and released photosynthetic oxygen into the sediment, producing an effect similar to the rhizosphere oxidation associated with isoetids.
The extent to which E. acicularis oxidized sediment was also unexpected. This isoetid-like macrophyte increased sediment redox potentials in the greenhouse experiment as much as or more than the isoetids. The greenhouse total biomasses of E. acicularis in both sediment types only exceeded E. aquaticum's in Raquette and were never significantly different from L. dortmanna's greenhouse biomasses. Consequently, there is little evidence to suggest that differences in biomass were responsible for the higher redox potentials of sediment containing E. acicularis in the greenhouse. The Limekiln field data, however, indicate that E. acicularis may have a lower root to shoot ratio than the isoetids possess, a conclusion similar to that for U. resupinata.
While redox potentials measured near E. acicularis in the field were always lower than those recorded near isoetids, the mean total and belowground biomasses for E. acicularis were lower than those of the isoetids in Limekiln Lake. The sampled E. acicularis were found at greater water depths than the other investigated macrophytes in the lake. Light attenuation may have limited the growth of this species (Ashton and Bissell Citation1987), reducing the plants’ ability to oxidize the sediment. Nevertheless, the greenhouse and field results explain the existence of iron plaques on the roots of E. acicularis: the biogeochemical effects of isoetids on sediment, including iron oxidation, likely occur in the presence of this species. This may apply to a related macrophyte, Eleocharis sphacelata, that also releases oxygen along its entire root system (Sorrell et al. Citation1993).
Although the differences were not always significant, all species barring E. acicularis in the greenhouse experiment yielded lower mean sediment redox potentials in Raquette Lake sediment than in Big Moose substrate. Total dry biomasses significantly differed between the sediment types for only E. aquaticum. The greater acidity of Big Moose porewater may have contributed to the higher potentials in that sediment type. Acidic conditions adversely affect some fungi and bacteria involved in decomposition (Kelly et al. Citation1984; Hoeniger Citation1986), reducing the consumption of oxygen in the sediment. Bare Big Moose sediment was more acidic than bare Raquette sediment. This suggests that, for at least part of the experiment, microbial decomposition consumed more oxygen in Raquette sediment than in Big Moose substrate, lowering the redox potential in the former.
Declines in sediment porewater pH were expected for the isoetids and U. resupinata and E. acicularis similarly lowered the pH values. These results are consistent with the sediment oxidation by these species. Since sediment oxidation is associated with the acidification of porewater (Wium-Andersen and Andersen Citation1972), the observed porewater pH reductions further demonstrate the biogeochemical sediment modification similarities between isoetids and the two non-isoetid species.
In addition to exhibiting isoetid-like sediment oxidation and porewater acidification, E. acicularis also displayed an ability to decrease sediment CO2 levels. Eleocharis acicularis was the only plant associated with significantly lower CO2 concentrations in Big Moose sediment. One possible explanation for this is that porewater CO2 is an important carbon source for E. acicularis. Since isoetid roots absorb CO2 (Wium-Andersen Citation1971; Pedersen and Sand-Jensen Citation1992; Winkel and Borum Citation2009), it was unexpected that the isoetids did not observably lower porewater CO2. Alternatively, the acidic porewater in the E. acicularis Big Moose treatment may have decreased microbial decomposition in that sediment, limiting CO2 production.
The sediment oxidation and porewater acidification in the greenhouse experiment and field study suggest that E. acicularis and U. resupinata, despite lacking several of the isoetid growth form's morphological features, are capable of extensive sediment modification. This conclusion, combined with the similarities in habitat and appearance between the four examined species, highlights the need for careful classification of aquatic macrophytes: extensive sediment oxidation is not limited to isoetids.
Acknowledgments
We thank Nakita Persaud, Kristine Bova, Alyssa Kulp, and Craig Reynolds for their assistance. We also thank Dr John Titus for constructive comments on an earlier draft of the manuscript and two anonymous reviewers for their helpful recommendations. This research was funded in part by a Grant-In-Aid of Research from the National Academy of Sciences, administered by Sigma Xi, The Scientific Research Society; a Lebanon Valley College Faculty Research Grant; and the Lebanon Valley College Wolf Biology Research Fund.
References
- Ashton , FM and Bissell , SR . 1987 . Influence of temperature and light on dwarf spikerush and slender spikerush growth . Journal of Aquatic Plant Management , 25 : 4 – 7 .
- Borman , SC , Galatowitsch , SM and Newman , RM . 2009 . The effects of species immigrations and changing conditions on isoetid communities . Aquatic Botony , 91 : 143 – 150 .
- Brouwer , E , Bobbink , R and Roelofs , JGM . 2002 . Restoration of aquatic macrophyte vegetation in acidified and eutrophied softwater lakes: an overview . Aquatic Botony , 73 : 405 – 431 .
- Chapin III , FS . 1980 . The mineral nutrition of wild plants . Annual Review of Ecology System , 11 : 233 – 260 .
- Christensen , KK , Jensen , HS , Andersen , FØ , Wigand , C and Holmer , M . 1998 . Interferences between root plaque formation and phosphorus availability for isoetids in sediments of oligotrophic lakes . Biogeochemistry , 43 : 107 – 128 .
- Christensen , PB and Sørensen , J . 1986 . Temporal variation of denitrification activity in plant-covered, littoral sediment from Lake Hampen, Denmark . Applied and Environmental Microbiology , 51 : 1174 – 1179 .
- Crow , GE and Hellquist , CB . 2000a . Aquatic and wetland plants of northeastern North America: angiosperms: monocotyledons , Vol. 2 , Madison , WI : University of Wisconsin Press .
- Crow , GE and Hellquist , CB . 2000b . Aquatic and wetland plants of northeastern North America: pteridophytes, gymnosperms, and angiosperms: dicotyledons , Vol. 1 , Madison , WI : University of Wisconsin Press .
- Gleason , HA and Cronquist , A . 1991 . Manual of vascular plants of Northeastern United States and adjacent Canada , New York , NY : Botanical Garden Press .
- Herberich , E , Sikorski , J and Hothorn , T . 2010 . A robust procedure for comparing multiple means under heteroscedasticity in unbalanced designs . PLoS One , 5 ( 3 ) : e9788
- Hoeniger , JFM . 1986 . Decomposition studies in two central Ontario lakes having surficial pHs of 4.6 and 6.6 . Applied and Environmental Microbiology , 52 ( 3 ) : 489 – 497 .
- Holm , S . 1979 . A simple sequentially rejective multiple test procedure . Scandinavian Journal of Statistics , 6 ( 2 ) : 65 – 70 .
- Holmer , M , Jensen , HS , Christensen , KK , Wigand , C and Andersen , FØ . 1998 . Sulfate reduction in lake sediments inhabited by the isoetid macrophytes Littorella uniflora and Isoetes lacustris . Aquatic Botony , 60 : 307 – 324 .
- Hutchinson , GE . 1975 . A treatise on limnology: limnological botany , Vol. 3 , New York , NY : John Wiley .
- Kelly , CA , Rudd , JWM , Furutani , A and Schindler , DW . 1984 . Effects of lake acidification on rates of organic matter decomposition in sediments . Limnology and Oceanography , 29 ( 4 ) : 687 – 694 .
- Long , JS and Ervin , LH . 2000 . Using heteroscedasticity consistent standard errors in the linear regression model . The American Statistician , 54 ( 3 ) : 217 – 224 .
- Pedersen , O and Sand-Jensen , K . 1992 . Adaptations of submerged Lobelia dortmanna to aerial life form: morphology, carbon sources and oxygen dynamics . Oikos , 65 : 89 – 96 .
- Pedersen , O , Sand-Jensen , K and Revsbech , NP . 1995 . Diel pulses of O2 and CO2 in sandy lake sediments inhabited by Lobelia dortmanna . Ecology , 76 ( 5 ) : 1536 – 1545 .
- Raven , JA , Handley , LL , MacFarlane , JJ , McInroy , S , McKenzie , L , Richards , JH and Samuelsson , G . 1988 . The role of CO2 uptake by roots and CAM in acquisition of inorganic C by plants of the isoetid life form: a review, with new data on Eriocaulon decangulare L . New Phytologist , 108 ( 2 ) : 125 – 148 .
- Rhoads , AF and Block , TA . 2007 . The plants of Pennsylvania, , 2nd , Philadelphia , PA : University of Pennsylvania Press .
- Richardson , K , Griffiths , H , Reed , ML , Raven , JA and Griffiths , NM . 1984 . Inorganic carbon assimilation in the isoetids, Isoetes lacustris L . and Lobelia dortmanna L. Oecologia , 61 ( 1 ) : 115 – 121 .
- Risgaard-Petersen , N and Jensen , K . 1997 . Nitrification and denitrification in the rhizosphere of the aquatic macrophyte Lobelia dortmanna L . Limnology and Oceanography , 42 ( 3 ) : 529 – 537 .
- Rothrock , PE and Wagner , RH . 1975 . The autecology of an acid tolerant sedge . Castanea , 40 ( 4 ) : 279 – 289 .
- Sand-Jensen , K , Prahl , C and Stokholm , H . 1982 . Oxygen release from roots of submerged aquatic macrophytes . Oikos , 38 ( 3 ) : 349 – 354 .
- Sorrell , BK , Brix , H and Orr , PT . 1993 . Oxygen exchange by entire root systems of Cyperus involucratus and Eleocharis sphacelata . Journal of Aquatic Plant Management , 31 : 24 – 28 .
- Stumm , W and Morgan , JJ . 1981 . Aquatic chemistry: an introduction emphasizing chemical equilibria in natural waters, , 2nd , New York , NY : Wiley-Interscience .
- Winkel , A and Borum , J . 2009 . Use of sediment CO2 by submersed rooted plants . Annals of Botany , 103 : 1015 – 1023 .
- Wium-Andersen , S . 1971 . Photosynthetic uptake of free CO2 by the roots of Lobelia dortmanna . Physiologica Plantarum , 25 : 245 – 248 .
- Wium-Andersen , S and Andersen , JM . 1972 . The influence of vegetation on the redox profile of the sediment of Grane Langsø, a Danish Lobelia lake . Limnology and Oceanography , 17 : 948 – 952 .