Abstract
Length measurements of fishes are commonly used by fishery scientists because of their ease of collection and the profound insight into fish populations that they provide. Specifically, obtaining accurate fish lengths is imperative for obtaining accurate population demographics. Thus, reproducibility and consistency of measuring an individual fish over time can greatly influence population level metrics and ultimately management decisions. To investigate this, we recorded fork lengths of 526 shovelnose sturgeon Scaphirhynchus platorynchus captured, measured, and marked in the field and recaptured within six years. Secondarily, we evaluated 37 shovelnose sturgeon measured in a controlled laboratory setting. We found that in both our field and laboratory evaluation that experienced biologists (between 5 and 20 years experience as fishery biologists) inaccurately measured (greater than 15 mm reduction in length) shovelnose sturgeon in approximately 5% of all cases. Of the approximately 5% erroneous measurements, substantial negative growth (greater than 100 mm reduction in length) occurred in some situations. This suggests that errors in recorded fish lengths exist and because of this, we believe multiple steps should be taken to minimize measurement error. Individual fishery biologists performing measurements should strive to ensure every fish is measured properly (i.e., fish laid completely flat on measuring board) and is recorded accurately. To this end, fishery biologists must accept that measurement error occurs and perform periodic quality assurance/quality control measures to account for these inaccuracies.
Introduction
Fish have indeterminate growth, indicating that fish continue to grow throughout their life (Pflieger Citation1997). Knowing and understanding this allows us to characterize the size structure of a population using a measurement of length. Fishes are measured using several methods including fork length, total length, and standard length. Standard length measures the length from the distal end of the snout to the caudal peduncle. Total length measures the fish from the longest point on the head to the longest lobe of the caudal fin. Fork length measures from the tip of the snout to the center of the fork in the tail. As is the case in this study, primitive fishes with heterocercal tails are generally measured with fork length (Baker et al. Citation2008; Koch et al. Citation2009). However, with any of these measures of length it may be possible to obtain erroneous length measures. With that being understood, obtaining accurate fish lengths is imperative for obtaining accurate population demographics because the reproducibility and consistency of measuring an individual fish over time can greatly influence population level metrics. For example, inaccurate length measurements can lead to erroneous growth rates and inaccurate estimates of size structure. Ultimately, these inconsistencies can lead to poor management decisions which can put a fishery at risk.
Specific to our study, determination of shovelnose sturgeon, Scaphirhynchus platorynchus, growth may be hindered due to conflicting estimates of length leading to false measurements of growth and size structure. Therefore, with the lack of knowledge in the literature that we uncovered, we initiated a study involving length measurements of shovelnose sturgeon. Specifically, we evaluated variability in length from field-based collections and then used an experimental approach to determine the specific mechanism for understanding this variability. Unfortunately, no measurement accuracy data were available, so validation of length was impossible. Therefore, the objectives of our study were to compare length estimates of shovelnose sturgeon captured and subsequently recaptured at a later date in a field setting, assuming negative growth (≥15 mm) was error. Secondly we evaluated variability in length and the specific mechanism for this variability using a mark–recapture approach (over a two-hour period) in a controlled setting.
Methods
Field-based evaluation
Shovelnose sturgeon were captured in the Middle Mississippi River during 2006–2011 using gill nets, trotlines, and bottom trawling. At point of initial capture (i.e., mark) shovelnose sturgeon were measured for fork length by experienced measurers (5–20 years experience as fishery biologists and biologists were unaware that the study was occurring), marked with a floy tag, and released. At any time during the aforementioned period that these shovelnose sturgeon were caught again (recapture), sturgeon were again measured by an experienced reader for fork length and released.
To determine what percentage of fish were measured inaccurately, we assumed that instances of substantial negative growth (i.e., greater than 15 mm) were measurement inaccuracies. The amount of negative growth determined substantial was based on the finding of Huusko et al. (Citation2010); on average their fish shrank naturally by approximately 2.5%. Using this information we suggested that any change less than 2.5% was variability in measuring and anything greater was measurement error. As such, we multiplied the average shovelnose sturgeon length (600 mm) by 2.5% to obtain 15 mm as our threshold for substantial error. Furthermore, to quantitatively determine whether measurement inaccuracy was related to the time period between initial capture measurement and recapture measurement, a one-way analysis of variance (ANOVA) was used. To accommodate the ANOVA model, we categorized time into three categories, less than 100 days between mark and recapture measurements, between 100 and 1000 days between mark and recapture measurements, and greater than 1001 days between mark and recapture.
Laboratory evaluation
For our laboratory evaluation, 37 shovelnose sturgeon were acquired from the middle Mississippi River using methods described above and subsequently brought back to the Open Rivers and Wetland Field Station (Jackson, MO) and allowed to acclimate in a 350 gallon round livestock watering tank 24 h prior to experimentation. At the onset of the laboratory experiment, shovelnose sturgeon were captured with a metal-framed dipnet, measured for fork length by an experienced measurer (5–20 years as fishery biologists and they were aware that the study was occurring), marked with a pre-labeled floy tag and released back into the tank. After all shovelnose sturgeon had been initially measured, we allowed a two-hour acclimation period and then the sturgeon were subsequently recaptured with the metal-framed dipnet, measured for fork length by an experienced measurer (same as above), and the floy tag number was recorded.
To analyze these data, we used an approach similar to our field-based evaluation described above. We determined what percentage of sturgeon were measured inaccurately, we again assumed that substantial negative growth (i.e., loss greater than 15 mm) was measurement inaccuracy but also included fish that gained more than 15 mm in length. We further assessed variability in length by assuming the first length measurement was accurate and any deviation was used to calculate mean measurement error (MME). Specifically, MME was defined as the sum of the absolute deviations between repeated measurements divided by the number of fish. To further assess this variability, length estimates between the first and second measurements were analyzed by comparing the slope of the length bias plot to a slope of one, an approach similar to that used by Campana et al. (Citation1995).
Results
Field evaluation
Over the course of our six-year field study, 526 shovelnose sturgeon were captured and recaptured. All fork lengths of these shovelnose sturgeon from the initial capture (mean fork length = 575 mm, standard error (SE) = 3.53, range 215–835) and the recapture (mean fork length = 585 mm, SE = 4.14, range 212–786) were used in our analyses. A total of 23 (4.6%) shovelnose sturgeon measurements (based on mark and recapture estimates) were inaccurate (lost more than 15 mm between initial capture measurement and recapture; ). In six of these cases, shovelnose sturgeon lost greater than 100 mm fork length from initial capture to recapture. Of these major inaccuracies, one shovelnose sturgeon lost approximately 241 mm from the point of initial capture to recapture (loss of greater than 50% of its entire body length; ). With regard to the amount of time between initial capture and recapture, similar error variability in erroneous measurements occurred across the three time periods (F 2,20 = 0.22, p = 0.806).
Laboratory evaluation
During our laboratory evaluation, 37 shovelnose sturgeon were acquired from the Middle Mississippi River. All fork lengths of these 37 shovelnose sturgeon from the initial capture (mean fork length = 611 mm, SE = 5.63, range 268–721) and recapture (mean fork length = 611 mm, SE = 6.01, range 430–764) were used in our analyses. A total of 2 (5.4%) shovelnose sturgeon measurements (based on mark and recapture estimates) were inaccurate assuming fish growth is indeterminate (lost or gained more than 15 mm between initial capture measurement and recapture; ). Of these major inaccuracies, one shovelnose sturgeon lost approximately 100 mm while another gained approximately 500 mm from the point of initial capture to recapture. MME across samples was 19.21, suggesting the average fish was inaccurately measured by greater than 19 mm. Furthermore, the slope of the length bias plot was different from zero (t = 2.84, n = 37; p = 0.007); indicating lack of precision in measurement between first and second measurements.
Figure 2. Experimental results of shovelnose sturgeon growth in millimeters (calculated as fork length of second measurement subtracted from fork length at initial measurement) plotted with individual fish identification number. Notes: Dotted line represents perfect agreement (i.e., 1:1) if there were no differences between second fork length measurement and initial fork length measurement. Slope test was used to evaluate whether the slope was different from zero. The number of observations is n. Note an exceedingly high value (+496 mm) and an extremely low value (−100 mm) were present within this data set.
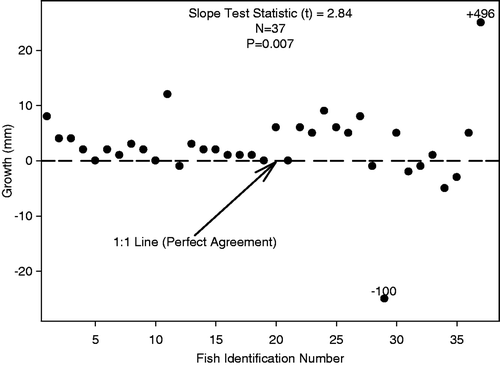
Discussion
Based on the results uncovered in this study, errors are occurring when measuring and recording shovelnose sturgeon lengths. It is apparent that shovelnose sturgeon measurement inaccuracies could lead observers to believe that sturgeon are shrinking. However, if the shrinking fish theory was indeed true, this should not be apparent over as short a time period as used in our laboratory experiment and it does not seem feasible that fish from our field observations could lose approximately 250 mm. Furthermore, we are unaware of any study in the fisheries peer-reviewed literature that has shown that shovelnose sturgeon shrink with an increase in age. As such, we believe that the negative growth uncovered in this study was simply measurement error and we are inclined to follow the numerous published research studies that suggest that fish growth is indeterminate (Sebens Citation1987; Baylis et al. Citation1993; Pflieger Citation1997; Lester et al. Citation2004).
Variations in fork length measurements may be attributable to several human induced factors. For example, when measuring fork length, determination of where the fork occurs depends on where the individual reader believes the fork is. Other sources of inaccuracies include damage to the tail fin (e.g., tail broke off), inadequate measuring technique, unreadable measuring board, or fish not being flat and extended during measuring process. Other factors could include miscommunication between the individual measuring the fish and the data recorder. Because of these potential measurement inaccuracies, enforcement personnel are likely going to be faced with greater challenges in upholding regulations. Furthermore, if protecting the resource will rely on length-limit-based regulations, we suggest that fishery managers be conservative; that is, err on the side of caution in constructing their minimum size limit and use a slightly lower minimum length limit to account for error.
To this end, we believe multiple steps can be taken to minimize measurement error. Individual fishery biologists performing measurements should strive to ensure care is taken with each measurement and that every fish is measured properly (i.e., fish laying completely flat on measuring board). Individuals recording data need to pay close attention to the individual measuring the fish and have clear penmanship to ensure the data are properly recorded. In the case where other individuals are involved during fish measurement and recording; these individuals should double check measurements and bring attention to suspected erroneous lengths. Furthermore, adding an additional metric to the length data such as weight acts as another verification tool to ensure true measurements. These steps may help to minimize erroneous length values present in data sets. To this end, fishery biologists must accept that there can be discrepancies in fork length measurement and perhaps do periodic quality assurance/quality control to account for it (e.g., double/triple measures and blind measures).
References
- Baker , D W , Peake , S J and Keiffer , J D . 2008 . The effect of capture, handling, and tagging on hematological variables in wild adult lake sturgeon . North American Journal of Fisheries Management , 28 : 296 – 300 . doi: 10.1577/M06-255.1
- Baylis , J R , Wiegmann , D D and Hoff , M H . 1993 . Alternating life histories of smallmouth bass . Transactions of the American Fisheries Society , 122 : 500 – 510 . doi: 10.1577/1548-8659(1993)122<0500:ALHOSB>2.3.CO;2
- Campana , S E , Annand , M C and McMillan , J I . 1995 . Graphical and statistical methods for determining the consistency of age determinations . Transactions of the American Fisheries Society , 124 : 131 – 138 . doi: 10.1577/1548-8659(1995)124<0131:GASMFD>2.3.CO;2
- Huusko , A , Maki-Petays , A , Stickler , M and Mykra , H . 2010 . Fish can shrink under harsh living conditions . Functional Ecology , 12 : 163 – 170 .
- Koch , J D , Quist , M C , Pierce , C L , Hanson , K A and Steuck , M J . 2009 . Effects of commercial harvest on shovelnose sturgeon populations in the upper Mississippi River . North American Journal of Fisheries Management , 29 : 84 – 100 . doi: 10.1577/M08-115.1
- Lester , N P , Shuter , B J and Abrams , P A . 2004 . Interpreting the von Bertalanffy model of somatic growth in fishes: the cost of reproduction . Proceedings of the Royal Society B. , 271 : 1625 – 1631 . doi: 10.1098/rspb.2004.2778
- Pflieger , W L . 1997 . The fishes of Missouri , 372pp Jefferson City , MO : Missouri Department of Conservation .
- Sebens , K P . 1987 . The ecology of indeterminate growth in animals . Annual Review of Ecology, Evolution, and Systematics , 18 : 371 – 407 . doi: 10.1146/annurev.es.18.110187.002103