Abstract
Few studies of plankton communities address anthropogenic influences on lotic ecosystems using multiple methods. Here we used both morphological and molecular techniques (denaturing gradient gel electrophoresis) to explore the spatial diversity of the plankton communities in a subtropical urban river during the month of March 2011. We identified 64 morphological taxa and 36 molecular taxa. Our results indicated that plankton communities in the Houxi River might be strongly impacted by anthropogenic disturbance. Nutrient concentrations (nitrogen and phosphorus), water temperature, conductivity, and suspended solids increased whereas dissolved oxygen decreased dramatically from upstream to downstream, and these environmental variables were found to be significantly related (p < 0.05) to the plankton community composition. The genera tolerant to organic pollution, such as Cyclotella, Fragilaria and Navicula (Bacillariophyta), and Ankistrodesmus and Scenedesmus (Chlorophyta), were dominant in the river. The comparative (Mantel-type) tests on similarity matrices (RELATE) analysis revealed that the denaturing gradient gel electrophoresis (DGGE) profile was closely related to species composition. In addition, the matching coefficient was higher between the DGGE profile and environmental variables than between species composition and environmental variables. Our results suggested that DGGE fingerprinting was more effective for examining the relationship between the plankton communities and environmental conditions than the morphological method, and they further provided new molecular insight into the community-level assessment of water quality and lotic ecosystem health.
Introduction
In rivers, plankton communities are an important part of the microbial loop and play a basic role in the food web (Shen et al. Citation1990; Pomeroy et al. Citation2007). They are one of the most promising indicators of water quality due to their rapid response to environmental changes relative to larger animals and plants (Stevenson and Smol Citation2003; Jiang and Shen Citation2007). Thus, it is important to investigate the changes in the plankton community for assessing riverine ecosystem health (Liu et al. Citation2011). However, most studies that have investigated plankton assemblages in riverine ecosystems employed only one method (either the morphological method or the molecular method), each of which has limitations. Traditional identifications based on morphology are time-consuming, require expertise in taxonomy, and often fail to distinguish differences among similar planktonic organisms (Savin et al. Citation2004).
Molecular techniques have been applied successfully to investigate phytoplankton communities in some lakes, rivers, and oceans (Yu et al. Citation2008; Sun et al. Citation2010; Liu et al. Citation2011). For example, polymerase chain reaction-denaturing gradient gel electrophoresis (PCR-DGGE) can rapidly determine shifts in microbial communities and is especially suitable for the small and morphologically similar organisms that cannot be distinguished by microscopy. Nevertheless, DGGE will only capture the dominant members of the assemblage (e.g., abundance above 0.3–0.4% of the total targeted cells) and a single DGGE band may contain multiple sequences (Díez et al. Citation2001). As a result, the combination of both methods can provide a more comprehensive and tangible characterization of the plankton community diversity and composition (Savin et al. Citation2004; Yu et al. Citation2008; Sun et al. Citation2010).
Plankton communities are very widespread and ecologically important to aquatic ecosystems, but their spatial diversity within subtropical urban rivers is not well known. In this study, both the molecular (DGGE) and morphological methods were employed to compare plankton communities in different sites along the Houxi River. The main environmental variables were monitored simultaneously. The Houxi River was selected for this study because environmental conditions and the extent of anthropogenic disturbance change dramatically along its length and such changes were hypothesized to alter the plankton communities.
The objectives of this study were: (1) to examine the diversity and composition of the plankton community along a subtropical urban river, (2) to determine if and how the diversity and the composition of the plankton community was affected by anthropogenic disturbance, and (3) to compare plankton communities based on the traditional morphological method and the DGGE molecular method.
Methods
Study area and sampling
The Houxi River is located on the west of Xiamen city (southeast China) with a total length of 23 km and a drainage area of 146.2 km2. It has a distinctive, subtropical oceanic monsoon climate with an annual mean temperature of 21°C and a mean annual precipitation of 1100 mm. The rainfall is concentrated from May to August. To assess the effect of human activity on the composition and diversity of plankton communities, 12 sites were sampled along the Houxi River in March 2011 (). Site 1 was located in the headwater of the Houxi River. This area is dominated by dense forest and is exposed to minimal human activity. Sites 2 and 3 and sites 4 and 5 were located in the Shidou and Bantou reservoirs, respectively. Both reservoirs are important drinking water resources and impacted by light human activities, such as camping, fishing and hiking at low densities and frequencies. Sites 6–8 from the middle of the Houxi River were located in a rural region. This area may be impacted by the nearby road, low-density housing, and intensive agriculture activities. Finally, sites 9–12 were located within a newly urbanized area (Jimei) to the west of Xiamen City.
Water samples for the plankton and physicochemical analysis were taken from the surface of the river (upper 50 cm). Microbial community samples (400 mL water) for the DGGE analysis were collected on 0.22-µm pore size polycarbonate filters (47 mm diameter, Millipore, USA). The filters were stored at −80°C until DNA extraction. About 2.5 L of water samples for the plankton quantitative analysis were fixed with Lugol's solution, allowed to settle for 48 h, and subsequently concentrated to 25 mL.
Environmental variables
Water temperature, conductivity, pH, dissolved oxygen (DO), and chlorophyll a were measured in situ with a multi-parameter water quality analyzer (Hydrolab DS5, Hach Co., USA). Suspended solids (SS) were determined gravimetrically by filtering 350 mL water sample through a pre-weighed filter (pore size of 0.45 µm), then weighing the filter again after drying at 105°C. Concentrations of total carbon (TC), total organic carbon (TOC), total nitrogen (TN), ammonium nitrogen (NH4–N), nitrite and nitrate nitrogen (NO x –N), total phosphorus (TP), and phosphate phosphorus (PO4–P) were determined according to the national standard methods (Wei Citation2002) as soon as possible after sampling.
Morphological analysis
Plankton were identified and counted under an inverted microscope (Motic, China), according to Shen et al. (Citation1990), Zhang and Huang (Citation1991), and Hu and Wei (Citation2006). Three subsamples were examined from each site.
DNA extraction and PCR
Total DNA was extracted using an E.Z.N.A Soil DNA Kit (Omega Bio-Tek, USA) according to the manufacturer's instructions. The extracted DNA was dissolved in 50 µL of TE buffer, quantified by spectrophotometer, and stored at −40°C until further use.
The 18 S rRNA gene fragments were amplified with the eukaryotic primers, Euk1A (5′-CTG GTT GAT CCT GCC AG-3′) and Euk516r-GC (5′-ACC AGA CTT GCC CTC CCG CCC GGG GCG CGC CCC GGG CGG GGC GGG GGC ACG GGG GG-3′; Díez et al. Citation2001). PCR mixtures (50 µL) contained 1 × PCR buffer, 1.5 mM of MgCl2, 200 µM each of deoxynucleoside triphosphate, 0.3 µM of each primer, 2.5 U of Taq DNA polymerase (TaKaRa, Japan), and approximately 40 ng of template DNA. The PCR program included an initial denaturation at 94°C for 5 min, followed by 35 cycles of denaturation at 94°C for 30 s, annealing at 52°C for 30 s, and extension at 72°C for 60 s. During the last cycle of both programs, the length of the extension step was increased to 10 min.
Denaturing gradient gel electrophoresis
DGGE was performed with a DCode mutation detection system (Bio-Rad, USA). Samples containing approximately equal amounts of PCR amplicons were loaded onto 6% (w/v) polyacrylamide gels (37.5 : 1 acrylamide : bisacrylamide) in 1 × Tris-acetate-EDTA (TAE) buffer. The denaturing gradient of 20–50% was applied (100% denaturant is defined as 7 M urea and 40% (v/v) deionized formamide). Electrophoresis was performed at 60°C with a constant voltage of 100 V for 16 h. The DGGE gels were stained with SYBR Green I nucleic acid stain for 30 min in 1 × TAE buffer, rinsed in distilled water, and then visualized with UV radiation using Gel Doc EQ imager (Bio-Rad, USA; ). The DGGE profile was analyzed using Quantity One software (Bio-Rad, USA), as described previously (Schauer et al. Citation2000). The intensity matrix was then constructed, taking into account the relative contribution of the band (as a percentage) to the total intensity of the lane.
Data analysis
Prior to the analysis, the DGGE intensity matrix was square root transformed. Raw abundance and environmental data were log(x + 1) transformed, with the exception of pH, to improve normality and homoscedasticity.
Principal component analysis (PCA) was used to group the samples according to environmental variables using the Pearson correlation coefficient. Redundancy analysis (RDA) was employed to evaluate the effect of environmental factors on the plankton community structure. A forward selection procedure was first applied to all the environmental variables and the significance of the conditional effects was detected each time by 999 Monte Carlo permutations of the residuals of the full regression models (ter Braak and Šmilauer Citation2002). For species data, the significant environmental variables were SS, conductivity, DO, water temperature, NH4–N, NO x –N, PO4–P, TN, and TP. And for DGGE fingerprint, SS, conductivity, DO, water temperature, NH4–N, NO x –N, TN, TP, and pH were significant (p < 0.05).
We used the Bray–Curtis coefficient as the measure of ordination distance among the plankton communities (Bray and Curtis Citation1957). Then, the multidimensional scaling (MDS) ordination was used to investigate differences in the plankton communities among the sites (Clarke and Gorley Citation2001). The significant differences (p < 0.01) between the groups were evaluated using the randomization/permutation procedure –analysis of similarities (ANOSIM). The global R statistic ranges from 0 to 1 and indicates the overall degree of separation between the groups of sites and no separation is indicated by R = 0, whereas R = 1 suggests complete separation.
RELATE works by relating the species composition similarity matrix to the DGGE intensity similarity matrix, the species composition similarity matrix to the environmental variables similarity matrix, and the DGGE intensity similarity matrix to the environmental variables similarity matrix by calculating rank correlation between the two matrices, thus creating a significance test with the matching coefficient ρ m, which is equivalent to Mantel's test (Clarke and Gorley Citation2001). We referred to positive and negative correlation levels between 0.5 and 1 as strong relationships at p ≤ 0.01. All data analyses were performed with the PRIMER 5.0 and the CANOCO 4.5 software packages.
Results
Environmental characteristics
Water temperature, conductivity, SS, and nutrients increased significantly from site 1 to site 12 (). The water temperature varied between 16.73°C and 19.76°C, conductivity ranged from 22.2 to 822.0 µS/cm, SS ranged from 1.3 to 116.7 mg/L, and TN, TP, NH4–N, NO x –N, and PO4–P concentrations ranged from 2.32 to 17.33 mg/L, 0.003 to 0.808 mg/L, 0.837 to 8.28 mg/L, 0.483 to 3.93 mg/L, and 0.001 to 0.540 mg/L, respectively. In contrast, pH (ranging from 7.45 to 8.25) and DO (ranging from 3.40 to 9.50 mg/L) decreased gradually from the upper to the lower river. Ranges for TC, TOC, TN/TP, and chlorophyll a were 0.449 to 1.049 mg/L, 0.247 to 0.725 mg/L, 16.8 to 1500 and 0.30 to 8.80 μg/L, respectively.
Table 1. The environmental parameters of the study sites in the Houxi River watershed in March 2011(see for the location of sampling sites).
The first PCA axis explained a large proportion of the total variance (79.2%) and the proportion increased to 90.8% when the second axis was added (). The environmental variables contributing most to the first axis were NH4–N (r = 0.95), conductivity (r = 0.95), NO x –N (r = 0.93), TN (r = 0.93), SS (r = 0.89), TP (r = 0.82), DO (r = − 0.75), water temperature (r = 0.64), and PO4–P (r = 0.62). Whereas, there were no environmental variables significantly (p < 0.05) correlated with the second axis.
Morphological diversity
A total of 46 phytoplankton taxa belonging to eight phyla (Bacillariophyta, Chlorophyta, Chrysophyta, Cryptophyta, Cyanophyta, Euglenophyta, Pyrrophyta, and Xanthophyta) were identified in the 12 sites. Phytoplankton abundance showed an increase from the upper to the lower surface of the Houxi River (A). The maximum phytoplankton abundance (10,747.2 individuals/L) was noted at site 12, while the lowest abundance (107.2 individuals/L) was observed at site 1. The greatest contributors to total phytoplankton abundance were Bacillariophyta (mean relative abundance = 38.66%), Chlorophyta (24.15%), Cyanophyta (20.99%), and Cryptophyta (14.43%).
Figure 4. Abundance of different phytoplankton (A) and zooplankton (B) groups at 12 sampling sites within the Houxi River watershed.
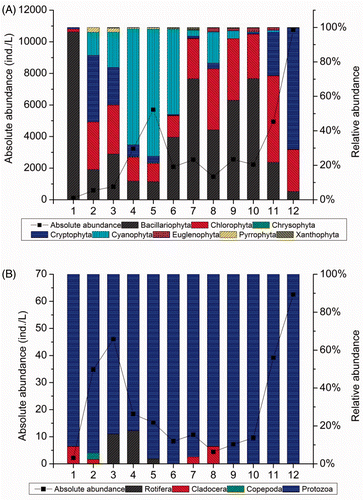
The zooplankton included Protozoa, Rotifera, Cladocera, and Copepoda among a total of 18 zooplankton taxa identified in the Houxi River. The abundance of zooplankton ranged from 2.2 individuals/L at site 1 to 62.5 individuals/L at site 12. Protozoa was the dominant group at all sampling sites and accounted for 94.72% of the zooplankton density in the Houxi River (B).
The plankton species number increased from 22 to 36 along the river. The Shannon–Wiener diversity index for plankton, which ranged from 1.22 to 2.54, decreased from site 1 to 5, and then increased from site 5 to 8. However, it decreased again from site 8 to 12 (A).
Molecular diversity
In total, 36 distinct bands were detected on the DGGE gels. Five bands were common to all sites (). The band number and the Shannon–Wiener diversity index increased from the upper to the lower surface of the Houxi River and varied from 12 and 2.30 (site 1) to 26 and 3.16 (site 9) with a mean of 19 and 2.82, respectively (B).
Correlation between morphological and molecular methods
The species composition was closely related to the DGGE profile, which was confirmed by the RELATE analysis (ρ m = 0.611, p = 0.001). In addition, the MDS ordination generated by comparing the species composition with the DGGE profile displayed similar patterns in general. Four groups were distinguished by both methods. Group I was composed of the headwater site 1, which exhibited the lowest species richness. Group II consisted of the four reservoir sites (sites 2–5) with less N and P pollution. Group III was located in a rural region and characterized by communities from more N- and P-polluted sites (6–8). Group IV was distributed in a newly urbanized area and impacted by most human activities (). The ANOSIM showed a global R value of 0.799 for species composition and 0.799 for the DGGE profile at p < 0.01, indicating a good separation between the four groups.
Relationship between plankton community and environmental gradients
The RDA ordination revealed that the significant environmental variables that were related to species composition of the plankton communities were water temperature, conductivity, DO, SS, TN, TP, NH4–N, NO x –N, and PO4–P at p < 0.05. Similarly, water temperature, conductivity, DO, SS, TN, TP, NH4–N, NO x –N, and pH were found to be significantly (p < 0.05) related to genetic diversity of the plankton communities. Along the first RDA axis, species composition and DGGE fingerprint patterns gradually and continuously changed from upstream to downstream with an increase of nutrients, conductivity, water temperature, and SS, and a decrease of DO. The cumulative variances of the species–environment relation explained by the first two axes were 67.0% and 57.0% for species and DGGE data, respectively ().
Figure 7. The RDA ordination showing the microbial community in the Houxi River in relation to the statistically significant environmental factors (p < 0.05), as revealed by species composition (A) and DGGE fingerprint (B). The numbers indicate the sampling sites.
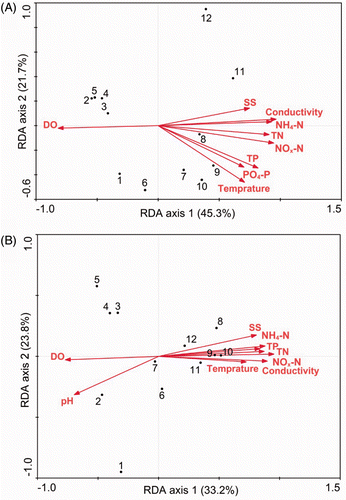
The RELATE analysis showed the matching coefficient ρ m of 0.431 between species composition and environmental variables, and 0.506 between the DGGE profile and environmental variables at P < 0.01, respectively.
Discussion
Influence of anthropogenic disturbance
Rivers are an important link between terrestrial environments, lakes, and the ocean and represent dynamic systems with highly variable conditions (Leff Citation1994). They are frequently subjected to anthropogenic impact (Tiquia Citation2010). Our results showed that the nutrient concentrations increased significantly from upstream sites to downstream sites. Moreover, the TN in all sites and TP in four downstream sites (sites 7–10) exceeded 2.0 and 0.4 mg/L, respectively, which are the Chinese standard limits for grade V surface water (Environmental Quality Standards for Surface Water, China GB3838-2002). In fact, cultural or anthropogenic eutrophication substantially degrades water quality of the middle to lower Houxi River due to high inputs of N and P from domestic sources and extensive agricultural and industrial activities ().
Further, our results showed that the plankton community composition differed among site 1, sites 2–5, sites 6–8, and sites 9–12 based on the ANOSIM. This suggests that the changing anthropogenic disturbance in the Houxi River may have affected the composition of the plankton community and that plankton also have the potential to serve as indicators of aquatic ecosystem health.
However, from upstream to downstream the plankton richness and diversity did not decrease significantly with anthropogenic pollutants (). The reason may be that there was a suite of planktonic organisms which were able to tolerate the water degradation associated with anthropogenic disturbance. Bacillariophyta (Cyclotella, Fragilaria and Navicula) and Chlorophyta (Ankistrodesmus and Scenedesmus) were the dominant groups at almost all sampling sites. These genera are known to be resistant to organic pollution because they have also been frequently recorded in waters that are nutrient rich and poorly oxygenated with high electrical conductivity (Shen et al. 1990; Bere and Tundisi Citation2011). Moreover, the plankton communities in the reservoir sites (sites 4 and 5, Bantou reservoir) and site 6 (outlet of the Bantou reservoir) were clearly dominated by Aphanizomenon (Cyanophyta), which is a common bloom-forming genus of cyanobacteria (Sellner Citation1997). During the dry season in 2010, we witnessed several mild cyanobacteria blooms in the Shidou and Bantou reservoirs. Yang et al. (Citation2012) investigated the algae community characteristics and trophic state for typical subtropical reservoirs in southeast Fujian, and indicated that the Shidou and Bantou reservoirs were eutrophic and significantly impacted by interactions among agriculture and urban factors. Therefore, it is urgent to improve the water management and water quality assessment in the Houxi River to protect water supplies in Xiamen.
Comparison of DGGE and morphological methods
Modern molecular fingerprinting methods allow the study of the diversity and dynamics of plankton communities in a rapid and rather accurate way (Díez et al. Citation2001). Among all of the available fingerprinting methods, PCR-DGGE is one of the more powerful methods. DGGE fingerprinting can more effectively measure the diversity of the plankton community than the morphological method (Yu et al. Citation2008) and has provided new insights into the community-level assessment of the water quality and ecosystem health in urban rivers. Our results support this view because our DGGE profile was closely related to species composition and both methods yielded, in general, similar estimates of the significance of environmental factors (). In addition, the matching coefficient ρ m was higher between the DGGE profile and environmental variables than between species composition and environmental variables (0.506 versus 0.431). This suggests that DGGE is a more sensitive technique for examining the relationship between the composition of plankton communities and the environmental conditions.
However, the DGGE method also has some limitations. For instance, the total number of species distinguished by microscopy was substantially larger than the number of distinct DGGE bands (64 versus 36). This could be due, in part, to the technical limitations of DGGE. It will only capture the dominate members of the microbial assemblage and a single band may contain multiple sequences. In addition, only eukaryotic primers were used in this study, so the prokaryotic cyanobacteria were excluded from the DGGE profile.
Conclusion
The plankton communities in the Houxi River were strongly related to the anthropogenic disturbance. The genera tolerant to organic pollution (e.g., Cyclotella, Fragilaria and Navicula of Bacillariophyta and Ankistrodesmus and Scenedesmus of Chlorophyta) were dominant in the river. The DGGE profile was closely related to species composition and both the methods indicated that the nutrient concentrations, water temperature, conductivity, SS, and DO had significant influence on the spatial variation of plankton communities. Moreover, compared to the species composition, the DGGE profile was more closely related to the environmental variables. This suggests that DGGE is a more powerful tool for assessing water quality and aquatic ecosystem health in river ecosystems.
Acknowledgments
This research was supported by the Knowledge Innovation Program of the Chinese Academy of Sciences (KZCX2-YW-QN401), the Xiamen Project of Science and Technology for Distinguished Young Scholars (3502Z20116006), the Natural Science Foundation for Distinguished Young Scholars of Fujian Province (2012J06009), the National Natural Science Foundation of China (31172114 and U1133601), and the China International Science and Technology Cooperation Program (2011DFB91710).
References
- Bere , T and Tundisi , J G . 2011 . Influence of ionic strength and conductivity on benthic diatom communities in a tropical river (Monjolinho), São Carlos-SP, Brazil . Hydrobiologia , 661 : 261 – 276 . doi: 10.1007/s10750-010-0532-0
- Bray , J R and Curtis , J T . 1957 . An ordination of the upland forest communities of southern Wisconsin . Ecological Monographs , 27 : 325 – 349 . doi: 10.2307/1942268
- Clarke , K R and Gorley , R N . 2001 . PRIMER v5: user manual/tutorial , Plymouth : PRIMER-E .
- Díez , B C , Marsh , P TL and Massana , R . 2001 . Application of denaturing gradient gel electrophoresis (DGGE) to study the diversity of marine picoeukaryotic assemblages and comparison of DGGE with other molecular techniques . Applied and Environmental Microbiology , 67 : 2942 – 2951 .
- Hu , H J and Wei , Y X . 2006 . The freshwater algae of China: systematics, taxonomy and ecology , Beijing : Science Press . (in Chinese)
- Jiang , J G and Shen , Y F . 2007 . Development of the microbial communities in Lake Donghu in relation to water quality . Environmental Monitoring and Assessment , 127 : 227 – 236 . doi: 10.1007/s10661-006-9275-9
- Leff , L G . 1994 . Stream bacterial ecology: a neglected field? . American Society for Microbiology News , 60 : 135 – 138 .
- Liu , L M , Yang , J and Zhang , Y Y . 2011 . Genetic diversity patterns of microbial communities in a subtropical riverine ecosystem (Jiulong River, southeast China) . Hydrobiologia , 678 : 113 – 125 . doi: 10.1007/s10750-011-0834-x
- Pomeroy , L R , Williams , P J , Azam , F and Hobbie , J E . 2007 . The microbial loop . Oceanography , 20 : 28 – 33 . doi: 10.5670/oceanog.2007.45
- Savin , M C , Martin , J L , Le Gresley , M , Giewat , M and Rooney-Varga , J . 2004 . Plankton diversity in the Bay of Fundy as measured by morphological and molecular methods . Microbial Ecology , 48 : 51 – 65 . doi: 10.1007/s00248-003-1033-8
- Schauer , M , Massana , R and Pedros-Alio , C . 2000 . Spatial differences in bacterioplankton composition along the Catalan coast (NW Mediterranean) assessed by molecular fingerprinting . FEMS Microbiology Ecology , 33 : 51 – 59 . doi: 10.1111/j.1574-6941.2000.tb00726.x
- Sellner , K G . 1997 . Physiology, ecology, and toxic properties of marine cyanobacteria blooms . Limnology and Oceanography , 42 : 1089 – 1104 . doi: 10.4319/lo.1997.42.5_part_2.1089
- Shen , Y F , Zhang , Z S , Gong , X J , Gu , M R , Shi , Z X and Wei , Y X . 1990 . Modern biomonitoring techniques using freshwater microbiota , Beijing : China Architecture & Building Press . (in Chinese)
- Stevenson , R J and Smol , J P . 2003 . “ Use of algae in environmental assessments ” . In Freshwater algae of North America ecology and classification , Edited by: Wehr , J D and Sheath , R G . 775 – 804 . Amsterdam : Academic Press .
- Sun , J , Yu , Z G , Gao , Y H , Zhou , Q Q , Zhen , Y , Chen , H T , Zhao , L Y , Yao , Q Z and Mi , T Z . 2010 . Phytoplankton diversity in the East China Sea and Yellow Sea measured by PCR-DGGE and its relationships with environmental factors . Chinese Journal of Oceanology and Limnology , 28 : 315 – 322 . doi: 10.1007/s00343-010-9285-x
- ter Braak , C and Šmilauer , P . 2002 . CANOCO reference manual and CanoDraw for windows user's guide-software for canonical community ordination (Version 4.5) , Ithac , NY : Microcomputer Power .
- Tiquia , S M . 2010 . Metabolic diversity of the heterotrophic microorganisms and potential link to pollution of the Rouge River . Environmental Pollution , 158 : 1435 – 1443 . doi: 10.1016/j.envpol.2009.12.035
- Wei , F S . 2002 . Monitoring and analysis methods of water and wastewater , 4th ed. , Beijing : China Environmental Science Press . (in Chinese)
- Yang , J , Yu , X Q , Liu , L M , Zhang , W J and Guo , P Y . 2012 . Algae community and trophic state of subtropical reservoirs in southeast Fujian, China . Environmental Science and Pollution Research , 19 : 1432 – 1442 . doi: 10.1007/s11356-011-0683-1
- Yu , Y H , Yan , Q Y and Feng , W S . 2008 . Spatiotemporal heterogeneity of plankton communities in Lake Donghu, China, as revealed by PCR-denaturing gradient gel electrophoresis and its relation to biotic and abiotic factors . FEMS Microbial Ecology , 63 : 328 – 337 . doi: 10.1111/j.1574-6941.2007.00430.x
- Zhang , Z S and Huang , X F . 1991 . Method for study on freshwater plankton , Beijing : Science Press . (in Chinese)