Abstract
Road salt application and resulting entrance of salt-laden runoff into streams are common winter events, yet little information exists about their influence on lotic periphyton function. We measured extracellular enzyme and photosynthetic activities of natural winter lotic periphyton communities under various short-term laboratory salt exposures. Short-term exposure to increased concentrations of salt reduced the photosynthetic activity of the periphyton but photosynthetic activity was restored by replacing salt-amended water with ambient stream water. Salt exposure also reduced periphytic β-glucosidase activity but not the activity of phosphatase or leucine aminopeptidase. Elevated, but realistic, short-term salt exposures reduced the functioning of lotic periphyton communities. Further work will be necessary to understand the long-term effects of increased salt concentrations on periphyton and extracellular enzyme activity.
Introduction
Winter roads are often maintained by applications of chemical deicers, usually road salt. Salt lowers the freezing point of water, thus preventing ice formation and encouraging melting (Oškinis and Kasperovičius 2005). In the US, maintenance of winter roads by applying salt was first introduced in the 1930s and has increased greatly since the 1960s (Paschka et al. Citation1999; Jackson and Jobbagy Citation2005). Several salts are used on roads with sodium chloride being the most common (Ramakrishna and Viraraghavan Citation2005).
Aquatic habitats near roadways or other impervious surfaces receive runoff containing road salt (e.g. Paschka et al. Citation1999; Kaushel et al. Citation2005; Oškinis and Kasperovičius 2005; Novotny et al. Citation2008). Winter road salt applications often lead to increased conductivity in streams during winter months (Kaushel et al. Citation2005; Oškinis and Kasperovičius 2005; Corsi et al. Citation2010). Some heavily impacted aquatic habitats can temporarily become extremely salty, with conductivities exceeding 30,000 µS cm−1 (; Silver et al. Citation2009; Corsi et al. Citation2010).
Figure 1. Specific conductance measurements (µS cm−1, n = 1456, 86 sites, 0–3 annual measurements per site, 1995–2006) from the Huron River and its tributaries in Washtenaw County, MI, USA, and surrounding region. Data are courtesy of the Huron River Watershed Council (http://www.HRWC.org).
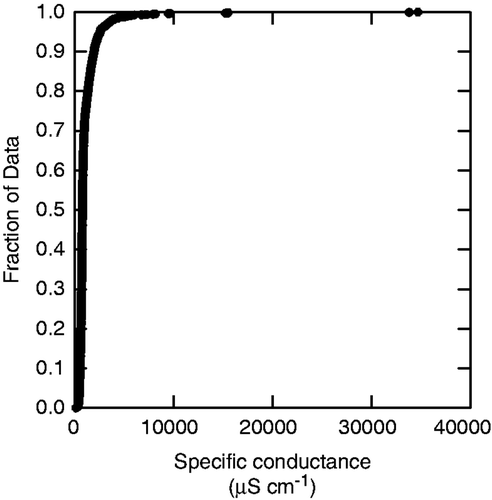
Periphyton is the community of microorganisms that grows attached to surfaces in aquatic environments. Benthic algae are an important component of periphyton in streams; their primary production contributes to the base of the food web (Bott Citation1996; Lamberti Citation1996). Furthermore, they are necessary as chemical modulators, stabilizers of substrata, and providers of habitats for other organisms (Biggs Citation1996; Mulholland 1996). Heterotrophic microorganisms (bacteria and fungi) may also be found in lotic periphyton (Rier et al. Citation2007), where they contribute to decomposition by producing extracellular enzymes to hydrolyze large organic molecules into smaller ones for subsequent uptake and use (Sinsabaugh et al. Citation1994; Allan and Castillo Citation2007).
Little is known about the influence of salinity arising from road salt application on the functioning of stream periphyton. Increased salinity may alter lotic algal biomass (Silva et al. Citation2000) and affect algal community composition (Oškinis and Kasperovičius 2005). Laboratory cultures of freshwater algae exposed to salt display reduced photosynthetic activity, regardless of whether photosynthetic activity was measured via oxygen production or fluorescence-based assays of photosystem II activity (Allakhverdiev et al. Citation2000; Lu and Zhang Citation2000; Sudhir et al. Citation2005). Photosynthesis (oxygen production) of lotic algae was enhanced by moderate ion concentrations (<∼5800 µS cm−1) and reduced by higher levels (∼10,200 µS cm−1; Silva and Davies Citation1999). Experimental salt additions inhibited pooled extracellular enzyme activity (fluorescein diacetate hydrolysis) of wetland microbial communities (Roache et al. Citation2006), suggesting that road salt additions could also inhibit specific extracellular enzyme activities in lotic periphyton.
The purpose of this study was to determine if lotic periphyton photosynthetic activity and extracellular enzyme activity could be influenced by road salt runoff. We hypothesized that both periphytic photosynthesis and enzymatic activity would be reduced by salt exposure. We tested these hypotheses by conducting laboratory experiments that exposed natural winter periphyton communities to realistic salt concentrations. We focused on measurements of periphytic maximum photosystem II efficiency and the activities of three periphytic extracellular enzymes that hydrolyze proteins, β-linked polysaccharides or organically bound organophosphoric esters (leucine aminopeptidase (LAMP), β-glucosidase and phosphatase (PASE), respectively; Chróst Citation1991). Maximum photosystem II efficiency is a relative measure of the maximum possible photosynthetic activity that a particular sample can produce (Kromkamp and Forster Citation2003; Consalvey et al. Citation2005).
Methods
Study site
This study was conducted in Paint Creek (N42.21502, W83.63494) just downstream of the Paint Creek wetland retention basin within the Stony Creek (MI, USA) watershed. Water temperature, specific conductance (YSI model 63), and water depth were recorded during sampling visits (one to six measurements at the sampling site at each sampling visit). Stream water was also collected for experimental use and measurements of total phosphorus (TP), ammonium (), nitrate and nitrite (
+
). Water samples for nutrient assays were frozen until analysis (Lind Citation1985) and samples for
, and
+
analyses were filtered (0.7 µm pore size, glass fiber filters) prior to freezing.
Photosynthesis experiment 1
Glass fiber filters (47 mm diameter) were prepared by sandwiching sets of six filters between two 6 × 43 cm2 plexiglass plates, one of which contained six circular openings (38 mm diameter) through which periphyton colonized the filters (see Kahn and Wetzel Citation1999; Francoeur and Wetzel Citation2003). The plexiglass plates were wired to bricks and placed on the streambed with the exposed surface of the filters facing upward and parallel to the streambed. After four weeks of periphyton growth (6 February 2007), the glass fiber filters were retrieved and returned to the lab for immediate experimentation. Care was taken to avoid losses of periphyton during collection and transport.
In the lab, four sections were cut from the filters using a cork borer (no. 10, 1.5 cm diameter). For this and all subsequent sectioning, obviously damaged areas were avoided, but otherwise sections were cut and allocated to treatments at random. These samples were preserved in 5% glutaraldehyde and stored at 4°C in darkness for later microscopy (transect scans of wet mounts, 400×) to determine division-level algal community composition.
Three replicate sections (each ∼176.7 mm2) of glass fiber filters were collected and frozen for subsequent chlorophyll analysis by the method of Biggs and Kilroy (Citation2000). Chlorophyll was extracted in 90% ethanol (80°C for five minutes) followed by overnight (4°C, darkness) steeping. Samples were then lightly centrifuged (4000–6000 rpm for two to five minutes) to ensure settling of particulate matter, and chlorophyll content was quantified from spectrophotometric absorbance at 665 and 750 nm, with acidification to correct for pheopigments.
The collected stream water was used to generate two salt treatments: ambient (1637.2 µS cm−1, unamended site water), and 35,320 µS cm−1 (site water amended with reagent-grade NaCl). Seven replicate sections (∼176.7 mm2) of glass fiber filter substrata were subjected to each salt treatment. The substrata were immersed in 5.0 mL of the treatment solution for 30 min before the first measurement (zero hours) of maximum photosystem II efficiency (Walz Diving PAM, see Consalvey et al. Citation2005) Additional maximum photosystem II efficiency measurements were collected at 3, 6 and 24 h. For the entire course of the experiment, all samples were held in darkness at 5°C.
Photosynthesis experiment 2
On 13 March 2007, natural rock substrata were collected from the Paint Creek study site and returned to the lab with care to avoid losses of periphyton during transport.
Immediately upon return to the lab, replicate sections (∼100 mm2) of the intact, cohesive periphyton mat were carefully removed from the flat rock surfaces by sectioning and slicing with a razor blade. Algal biomass (as chlorophyll a, n = 3) and community composition (n = 4) were quantified as described above.
Three salinity treatments were made by adding NaCl to stream water: 1609.8 µS cm−1 (unamended stream water), 4428 and 32,750 µS cm−1. Seven replicate periphyton sections were each immersed in 5.0 mL of each of the desired salt treatments. Incubation and maximum photosystem II efficiency measurements were conducted as described above, with the exception that after six hours of incubation, all sections were removed from incubation water, rinsed with unamended stream water, and placed into unamended stream water. Incubation resumed and maximum photosystem II efficiency measurements were made at 0.5 h after rinsing, 3 h after rinsing and 21 h after rinsing.
Extracellular enzyme activity experiment
Naturally senesced Typha litter was collected from the Paint Creek wetland <100 m upstream of the sampling site and placed in floating mesh trays (see Francoeur et al. Citation2006). After eight weeks of periphyton growth (6 March 2007), the Typha trays were retrieved and returned to the lab. Care was taken to avoid losses of periphyton during transport.
Immediately upon return to the lab, algal biomass (as chlorophyll a, n = 3) and community composition (n = 4) were quantified as previously described, with the exception that known lengths of Typha litter were cut for quantitative analyses (Francoeur et al. Citation2006). An additional four litter sections were frozen for subsequent analysis of fungal biomass (Francoeur et al. Citation2006; ). Additional samples were preserved in 4% formalin and stored at 4°C in darkness for subsequent analysis of bacterial abundance but were lost prior to assay.
Activities of three microbial extracellular enzymes (LAMP, β-glucosidase and PASE) were compared at two levels of salinity: 2009.2 µS cm−1 (ambient stream water) and ∼60,000 µS cm−1. Six replicates of the Typha litter sections and two litter-free blanks were used for each combination of salt treatment and enzyme type. Replicate litter sections (1.7 cm long) were pre-incubated in ambient or 33,040 µS cm−1 stream water for 1 h (5°C, in darkness) before enzyme activity assays. The assays followed the method and conditions of Francoeur et al. (Citation2006), which are saturating for these microbial communities. The containers containing the litter sections were aspirated of any liquid, then 2 mL of the appropriate 1000 µM enzyme substrate stock solution and 1 mL of 179,300 or 2009.2 µS cm−1 stream water were added to each container. The final concentration of extracellular enzyme substrate was 666 µM and final salt concentrations were approximately 60,000 µS cm−1 (elevated salt treatment) or 2009.2 µS cm−1 (ambient stream water treatment). Samples were incubated for 30 min in the dark at 5°C. At the end of the 30 min incubation, aliquots from each vial were added to a 96-well plate with equal volumes of pH 10 buffer and measured fluorometrically (Fluoroskan Ascent plate reader, 355 nm excitation, 460 nm emission, Thermo Fisher Scientific). Salt-induced changes in activity of a particular enzyme were inferred from differences in relative fluorescence intensity between the two salt treatments.
Statistical analysis
Photosynthesis experiment 1
A repeated-measures ANOVA (RMANOVA) was conducted to test the hypothesis that maximum photosystem II efficiency differed between ambient and elevated salt treatments over the 24 h experiment. Additionally, two-sample separate-variance t-tests were used to test for differences between ambient and elevated salt treatments at each individual measurement period.
Photosynthesis experiment 2
RMANOVA was used to test for differences in maximum photosystem II efficiency between salt treatments over the entire experiment. Bonferroni-based comparisons were used to identify differences between specific elevated salt treatments and the control. One-way ANOVAs with Tukey HSD multiple comparisons were used to test for differences among salt treatments at each individual measurement period. In addition, subsequent RMANOVAs considered only the pre-rinse measurements (0–6 h, when algae were exposed to three distinct salt treatments) or only the post-rinse measurements (6.5–27 h, when all algae were in ambient-salinity water). Finally, paired t-tests were used to test the hypothesis that maximum photosystem II efficiency within individual salt treatments differed between the 6 and 6.5 h measurements (i.e. immediately pre- and post-rinse).
Extracellular enzyme activity experiment
Two sample t-tests were used to test the hypothesis that activities of individual extracellular enzymes differed between ambient and elevated salt treatments.
For all experiments, data were transformed (log X + 1) as necessary to reduce heteroscedasticity. All statistical analyses were conducted using SYSTAT, Version 10 (Systat Software, Point Richmond, CA, USA).
Results
Over the course of the in situ incubations (4, 18 and 29 January 2007; 2 and 22 February 2007; 6 and 13 March 2007) the mean nutrient levels (± 1 SE) in Paint Creek were: nitrate + nitrite = 2.29 ± 0.56 (n = 6), ammonium 0.157 +/− 0.001 mg N L−1 (n = 6), TP = 58.3 ± 29.3 µg L−1 (n = 6). Stream water temperature averaged 4.5°C and specific conductance averaged 1649.8 µS cm−1 across the study period (). Algal biomass was lowest in the communities used for the enzyme activity experiment (), and algal communities were composed exclusively of Bacillariophyta.
Table 1. Environmental conditions at the Paint Creek field site.
Table 2. Mean (±1 SE) algal and fungal biomass of periphyton communities used for the three experiments.
In experiment 1, exposure to salt-amended stream water (35,320 µS cm−1) significantly reduced the maximum photosynthetic efficiency of photosystem II over the entire 24-h experimental period (p < 0.001, ), and at each individual sampling time (p always <0.001).
Figure 2. Effects of salt exposure on maximum photosystem II efficiency in experiment 1 (p < 0.001). Solid line is ambient (1637 µS cm−1) and dashed line is salt-amended (35,320 µS cm−1) stream water. Note: Values are means ± 1 SE (n = 7).
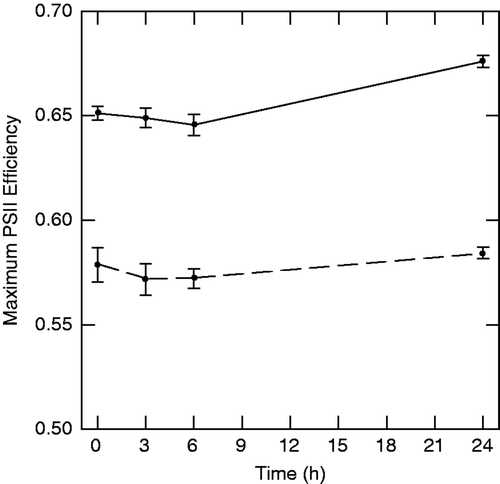
With regard to the full photosynthetic data set of experiment 2, RMANOVA detected significant differences among the salinity levels (p < 0.001), and a strong time × salinity interaction (p < 0.001; ). Both the medium (4428 µS cm−1, p < 0.004) and high (32,750 µS cm−1, p < 0.001) treatments differed from the ambient-salinity control; however, the time × salinity interaction was only apparent when comparing the high salinity and ambient treatments (p < 0.001); the ambient and medium-salinity treatments displayed the same pattern over time (p = 0.201).
Figure 3. Effects of salt exposure on maximum photosystem II efficiency in experiment 2. Solid line is ambient (1609 µS cm−1), dotted (4428 µS cm−1) and dashed (32,750 µS cm−1) lines are salt-amended stream water. The arrow indicates the rinse and replacement of treatment solutions with ambient-salinity stream water. Note: Values are means ± 1 SE (n = 7).
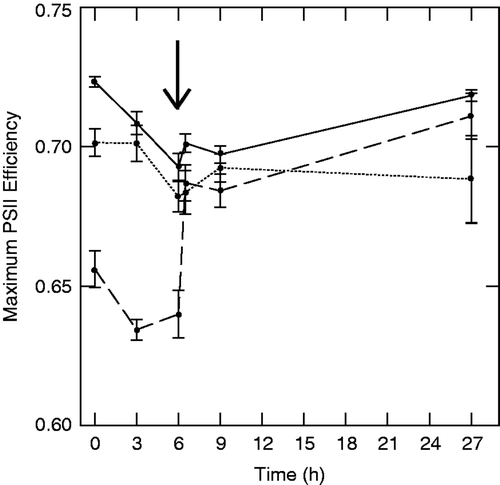
A RMANOVA considering only the pre-rinse sampling periods (i.e. times 0, 3 and 6) detected significant differences among salinity treatments (p < 0.001). Both high (p < 0.001) and medium (p = 0.006) salinity treatments differed from the ambient treatment. Maximum potential photosynthetic efficiency differed significantly among salinity treatments in every pre-rinse (i.e. times 0, 3 and 6) sampling period (one-way ANOVA, p < 0.001). The high salinity treatment was always significantly different from the ambient (p < 0.001) and medium salinity treatments (p < 0.001) at each individual sampling time, however, the ambient and medium-salinity treatments did not differ at individual pre-rinse sampling periods (p > 0.424).
Comparison of immediately pre-rinse (6 h) and immediately post-rinse (6.5 h) measurements showed that maximum photosystem II efficiency in the ambient water (1609.8 µS cm−1) and low salinity (4428 µS cm−1) treatments did not increase significantly (p = 0.071 and p = 0.797, respectively). However, the high salinity treatment (32,750 µS cm−1) did increase significantly during this timeframe (p < 0.001, ).
A RMANOVA considering only the post-rinse sampling period (i.e. times 6.5, 9 and 27) did not differ significantly among salinity treatments (p = 0.060) and each of the individual post-rinse sampling periods reflected this similarity among salt treatments (one-way ANOVA, p > 0.086).
PASE and LAMP activities were not affected by high (∼ 60,000 µS cm−1) salinity (p = 0.413 and p = 0.902, respectively). In contrast, salt exposure reduced β-glucosidase (BG) activity by ∼60% (p = 0.018, ).
Figure 4. Activities of periphytic LAMP, PASE and BG in ambient (2009.2 µS cm−1) and salt-amended stream water (∼60,000 µS cm−1). Notes: *indicates a significant difference between ambient and salt-amended treatments. Values are means of the fluorescence of hydrolysis products (unitless) ±1 SE (n = 6).
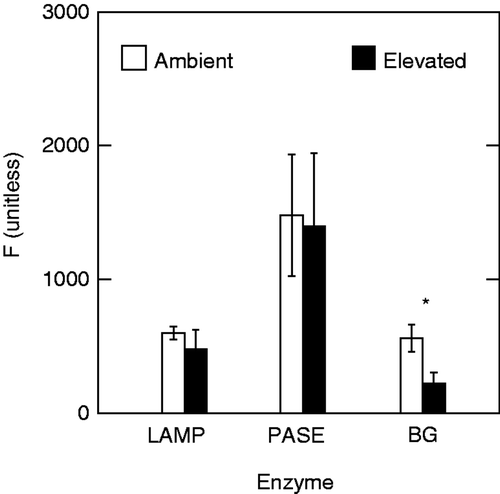
Discussion
The purpose of this study was to determine the short-term effects of salt stress on lotic microbial function in order to evaluate possible ecological effects from road salt runoff. We approached this task by exposing natural periphyton communities to simulated salting events. Laboratory conditions were calibrated to local environmental conditions in order to provide realistic salt concentrations and temperatures.
Potential photosynthetic activity showed a rapid reduction of activity when exposed to high, but realistic, salt concentrations. When high salt exposure samples were rinsed with ambient-salinity stream water, a rapid recovery of photosynthetic activity was observed. In a Canadian gravel-bed river, Silva and Davis (Citation1999) reported stimulation of natural lotic algal productivity by slightly increased salinity (up to ∼6000 µS cm−1). In contrast to Silva and Davis (Citation1999), we did not observe any stimulation of photosynthetic activity at medium levels of salinity. Our data indicated that these medium salinity levels had either no effect or were slightly inhibitory. In a Michigan, USA river, Steinman et al. (Citation2011) found no effect of moderate salinity (1500 µS cm−1) on benthic algal photosynthesis. The reduction in photosynthetic activity caused by high salinity in our study is similar to that reported by Silva and Davis (Citation1999), despite their high salinity treatment (∼10,500 µS cm−1) being well below our high salt concentration (∼ 29,900–35,320 µS cm−1).
We observed both a rapid salt-induced decline in potential photosynthetic ability and also a rapid recovery when high concentrations of salt were rinsed and replaced with unamended stream water. This general pattern of rapid decline and recovery is superficially similar to the results of Lu and Zhang (Citation2000), who observed instantaneous decline, then recovery of photosynthetic activity of laboratory cultures of the cyanobacterium Spirulina platensis during salt exposure. However, the recovery from salt stress in their experiment was spontaneous as it did not require removal of salt from the medium, perhaps the result of sodium being actively excluded from the cyanobacterial cells (Lu and Zhang Citation2000) In contrast, in our experiment recovery was due to removal of algae from the salt solution. Lu and Zhang (Citation2000) also reported that after four hours, there was a second, light-mediated depression of photosystem II activity by salt. This phenomenon was something that would not have been observed in our experiment as the samples were incubated in darkness. However, since spontaneous recovery of photosynthetic activity was not observed during our experiments, there is no way that a secondary inhibition could have occurred.
It is likely that the large differences in algal community composition between our experiments (natural algal communities almost exclusively composed of diatoms) and the purely cyanobacterial cultures of Lu and Zhang (Citation2000) caused this disparity in spontaneous photosystem II recovery. The fact that the cyanobacterial spontaneous photosystem II recovery observed by Lu and Zhang (Citation2000) was later reversed by light exposure strongly suggests that this apparent spontaneous recovery was dependent on the use of alternative photosynthetic electron pathways unique to cyanobacteria (Lu and Zhang Citation2000). Such pathways are lacking in plants and eukaryotic algae (see Campbell et al. Citation1998) and thus this phenomenon would not be expected to occur in algal communities dominated by eukaryotic diatoms.
A more critical finding of Lu and Zhang (Citation2000) is the decoupling of photosystem II efficiency and photosynthetic oxygen evolution by salt exposure. Should this decoupling be a common phenomenon, then rapid fluorometric measures of photosystem II efficiency would not be good proxies for traditional photosynthesis assays under these conditions. However, the discrepancy observed by Lu and Zhang (Citation2000) appears to entirely result from the apparent spontaneous recovery of photosystem II efficiency. As discussed above, this phenomenon is likely limited to cyanobacteria. Furthermore, this apparent spontaneous recovery can be easily distinguished by its sensitivity to light. Exposure to 100 µmol m−2 s−1 of light for several hours completely eliminated apparent spontaneous photosystem II recovery (Lu and Zhang Citation2000). Thus, rapid, PAM-based measurement of photosystem II efficiency should generally provide an adequate estimate of eukaryotic algal photosynthetic performance under salt stress.
A similar pattern of decrease and recovery of photosynthetic oxygen production by Synechococcus spp. exposed to salt was observed by Allakhverdiev et al. (Citation2000). In these experiments, the cyanobacteria were rinsed after 0.5, 1 or 5 h of exposure to 0.5 M NaCl and the oxygen activity was measured. The samples that were rinsed after 0.5 h of salt exposure were able to fully recover to pre-exposure levels of photosynthetic activity but the sample rinsed after 5 h of salt exposure did not recover photosynthetic ability. In contrast, our study showed full recovery of photosynthetic activity in a diatom-dominated periphyton community following a six hour exposure to salt. Taken together, these studies suggest that more work is required to understand the effects of salt on algae as different species may react differently when exposed to various levels of salt.
PASE and LAMP activities showed no significant response to salt exposure. This could imply that there was no immediate effect of salt on hydrolysis of organic phosphorus or amino acid chains. Increased salinity is not always associated with reduced enzyme activity in aquatic environments (Cunha et al. Citation2010). Perhaps the PASE and LAMP enzyme molecules in our periphyton communities were relatively insensitive to salt stress or were bound to surfaces within the periphyton community (Espeland et al. Citation2002), thereby buffering their activity against the short-term salt exposure of our experiments. In contrast, BG activity was reduced by salt exposure, suggesting that decomposition of cellulose-rich organic matter could have been inhibited. These results are consistent with the findings of Roache et al. (Citation2006), who observed that experimental salt additions similar to those used in our study inhibited microbial enzyme activity and plant litter decomposition in a freshwater wetland. The salt exposure used in our enzyme experiment was approximately double the highest recorded winter salt exposures in local streams (), making direct extrapolation of our results somewhat difficult.
Large but realistic, short-term salt exposures immediately reduced the maximum potential photosynthetic efficiency of lotic periphyton; this photosynthetic impairment was rapidly and completely reversible by rinsing with ambient stream water. Some, but not all, extracellular enzyme activities were reduced by high salt exposure. Thus, individual short-term salt exposures appear to temporarily depress the functioning of lotic periphyton communities.
Acknowledgments
Kevin Johnson, Mark Schaecher, Audrey Johnson, and Sarah Whorley assisted with field sampling and laboratory analyses. Drs Peggy Liggit and Gary Hannan provided comments on a draft of this article, and Dr Kevin A. Kuehn analyzed the fungal biomass samples. The Washtenaw County Office of the Water Resources allowed access to the Paint Creek research site and the Huron River Watershed Council provided local stream conductivity data for . This research was supported by funding from the Eastern Michigan University Center for Aquatic Microbial Ecology, the EMU Graduate School, and a Meta Hellwig Scholarship from the EMU Biology Department.
References
- Allakhverdiev , SI Jr , Sakamoto , A , Nishiyama , Y , Inaba , M and Murata , N . 2000 . Ionic and osmotic effects of NaCl-induced inactivation of photosystems I and II in Synechococcus sp . Plant Physiology , 123 : 1047 – 1056 . doi: 10.1104/pp.123.3.1047
- Allan , JD and Castillo , MM . 2007 . Stream ecology: structure and function of running waters , 2nd , Dordrecht : Springer .
- Biggs , BJF . 1996 . “ Patterns in benthic algae of streams ” . In Algal ecology: freshwater benthic ecosystems , Edited by: Stevenson , RJ , Bothwell , ML and Lowe , RL . 31 – 56 . San Diego (CA) : Academic Press .
- Biggs , BJF and Kilroy , C . 2000 . Stream periphyton monitoring manual , Christchurch, New Zealand : National Institute of Water and Atmospheric Research (NIWA) .
- Bott , TL . 1996 . “ Algae in microscopic food webs ” . In Algal ecology: freshwater benthic ecosystems , Edited by: Stevenson , RJ , Bothwell , ML and Lowe , RL . 573 – 608 . San Diego (CA) : Academic Press .
- Campbell , D , Hurry , V , Clarke , AK , Gustafsson , P and Oquist , G . 1998 . Chlorophyll fluorescence analysis of cyanobacterial photosynthesis and acclimation . Microbiology and Molecular Biology Reviews , 62 : 667 – 683 .
- Chróst , RJ . 1991 . “ Environmental control of the synthesis and activity of aquatic microbial ectoenzymes ” . In Microbial enzymes in aquatic environments , Edited by: Chróst , RJ . 29 – 59 . New York (NY) : Springer Verlag .
- Consalvey , M , Perkins , RG , Paterson , DM and Underwood , GJC . 2005 . PAM fluorescence: a beginners guide for benthic diatoms . Diatom Research , 20 : 1 – 22 . doi: 10.1080/0269249X.2005.9705619
- Corsi , SR , Graczyk , DJ , Geis , SW , Booth , NL and Richards , KD . 2010 . A fresh look at road salt; aquatic toxicology and water-quality impacts on local, regional, and national scales . Environmental Science and Technology , 44 : 7376 – 7382 . doi: 10.1021/es101333u
- Cunha , A , Almeida , A , Coelho , FJRC , Gomes , NCM , Oliveira , V and Santos , AL . 2010 . “ Bacterial extracellular enzymatic activity in globally changing aquatic ecosystems ” . In Current research technology and education topics in applied microbiology and microbial biotechnology. Microbiology Series 2, Volume 1 , Edited by: Mendez-Vilas , A . 124 – 135 . Spain : FORMATEX .
- Espeland , EM , Francoeur , SN and Wetzel , RG . 2002 . Microbial phosphatase in biofilms: a comparison of whole community enzyme activity and individual bacterial cell-surface phosphatase expression . Archiv für Hydrobiologie , 153 : 581 – 593 .
- Francoeur , SN , Schaecher , M , Neely , RK and Kuehn , KA . 2006 . Periphytic photosynthetic stimulation of extracellular enzyme activity in aquatic microbial communities associated with decaying Typha litter . Microbial Ecology , 52 : 662 – 669 . doi: 10.1007/s00248-006-9084-2
- Francoeur , SN and Wetzel , RG . 2003 . Regulation of periphytic leucine-aminopeptidase activity . Aquatic Microbial Ecology , 31 : 249 – 258 . doi: 10.3354/ame031249
- Jackson , RB and Jobbagy , EG . 2005 . From icy roads to salty streams . Proceedings of the National Academy of Sciences , 102 : 14487 – 14488 . doi: 10.1073/pnas.0507389102
- Kahn , WE and Wetzel , RG . 1999 . Effects of microscale water level fluctuations and altered ultraviolet radiation on periphytic microbiota . Microbial Ecology , 38 : 253 – 263 . doi: 10.1007/s002489900175
- Kaushel , SS , Groffman , PM , Likens , GE , Belt , KT , Stack , WP , Kelly , VR , Band , LE and Fisher , GT . 2005 . Increasing salinization of fresh water in the northeastern United States . Proceedings of the National Academy of Sciences , 102 : 13517 – 13520 . doi: 10.1073/pnas.0506414102
- Kromkamp , JC and Forster , RM . 2003 . The use of variable fluorescence measurements in aquatic ecosystems: differences between multiple and single turnover measuring protocols and suggested terminology . European Journal of Phycology , 38 : 103 – 112 . doi: 10.1080/0967026031000094094
- Lamberti , GA . 1996 . “ Role of periphyton in benthic food webs ” . In Algal ecology: freshwater benthic ecosystems , Edited by: Stevenson , RJ , Bothwell , ML and Lowe , RL . 533 – 572 . San Diego (CA) : Academic Press .
- Lind , OT . 1985 . Handbook of common methods in limnology , 2nd , Dubuque (IA) : Kendall/hunt .
- Lu , C and Zhang , J . 2000 . Role of light in the response of PSII photochemistry to salt stress in the cyanobacterium Spirulina platensis . Journal of Experimental Biology , 51 : 911 – 917 .
- Mulholland , PJ . 1996 . “ Role in nutrient cycling in streams ” . In Algal ecology: freshwater benthic ecosystems , Edited by: Stevenson , RJ , Bothwell , ML and Lowe , RL . 609 – 639 . San Diego (CA) : Academic Press .
- Novotny , EV , Murphy , D and Stefan , HG . 2008 . Increase of urban lake salinity by road deicing salt . Science of the Total Environment , 406 : 131 – 144 . doi: 10.1016/j.scitotenv.2008.07.037
- Oškinis , V and Kasperovičius , T . 2005 . Impact of road maintenance salts on water ecosystems according to diatom flora investigation . Journal of Environmental Engineering and Landscape Management , 13 : 51 – 55 .
- Paschka , MG , Ghosh , RS and Dzombak , DA . 1999 . Potential water-quality effects from iron cyanide anticaking agents in road salt . Water Environment Research , 71 : 1235 – 1239 . doi: 10.2175/106143096X122410
- Ramakrishna , DM and Viraraghavan , T . 2005 . Environmental impact of chemical deicers – a review . Water, Air, and Soil Pollution , 166 : 49 – 63 . doi: 10.1007/s11270-005-8265-9
- Rier , ST , Kuehn , KA and Francoeur , SN . 2007 . Algal regulation of extracellular enzyme activity in stream microbial communities associated with inert substrata and detritus . Journal of the North American Benthological Society , 26 : 439 – 449 . doi: 10.1899/06-080.1
- Roache , MC , Bailey , PC and Boon , PI . 2006 . Effects of salinity on the decay of the freshwater macrophyte, Triglochin procerum . Aquatic Botany , 84 : 45 – 52 . doi: 10.1016/j.aquabot.2005.07.014
- Silva , EIL and Davies , RW . 1999 . The effects of simulated irrigation induced changes in salinity on metabolism of lotic biota . Hydrobiologia , 416 : 193 – 202 . doi: 10.1023/A:1003827807547
- Silva , EIL , Shimizu , A and Matsunami , H . 2000 . Salt pollution in a Japanese stream and its effects on water chemistry and epilithic algal chlorophyll-a . Hydrobiologia , 437 : 139 – 148 . doi: 10.1023/A:1026598723329
- Silver , P , Rupprecht , SM and Stauffer , MF . 2009 . Temperature-dependent effects of road deicing salt on chironomid larvae . Wetlands , 29 : 942 – 951 . doi: 10.1672/08-212.1
- Sinsabaugh , RL , Osgood , MP and Findlay , S . 1994 . Enzymatic models for estimating decomposition rates of particulate detritus . Journal of the North American Benthological Society , 13 : 160 – 169 . doi: 10.2307/1467235
- Steinman , AD , Ogdahl , ME , Wessel , K , Biddanda , B , Kendall , S and Nold , S . 2011 . Periphyton response to simulated nonpoint source pollution: local over regional control . Aquatic Ecology , 45 : 439 – 454 . doi: 10.1007/s10452-011-9366-8
- Sudhir , P , Pogorylelov , D , Kovacs , L , Garab , G and Murthy , SDS . 2005 . The effects of salt stress on photosynthetic electron transport and thylakoid membrane proteins in the cyanobacterium Spirulina platensis . Journal of Biochemistry and Molecular Biology , 38 : 481 – 485 . doi: 10.5483/BMBRep.2005.38.4.481