Abstract
We assessed physicochemical and biological continuity in an 800-m reach of a small (<2 m wide) headwater stream during 2010 and 2012 in the northcentral U.S. Dense riparian canopy covered the first 600 m of stream reach, was lacking in an approximately 100-m portion due to historical (>10 years previously) agriculture, and returned in the last 100 m of the study area. Reflecting this difference, the forested area had less sunlight penetration to the water level and greater volume of woody debris in the stream. There was no difference in specific conductance or pH among sites. Water temperature was lower in the upstream forested sites and higher in the non-forested site and those downstream of it. Dissolved oxygen exhibited a reciprocal trend with temperature. The non-forested site exhibited an abrupt change in biological continuity as indicated by a decrease in benthic macroinvertebrate and adult caddisfly shredder abundance, an increase in filtering collector and scraper abundance, a decrease in trout abundance, and an increase in both total and warm-water fish species. The stream returned to its previous state when canopy returned downstream. Both years of the study yielded similar results. Our results indicate that abrupt changes in riparian canopy can lead to similarly abrupt changes in biological continuity, often within a relatively short (100 m) distance.
Introduction
Due to their small watershed areas, small streams are easily affected by minor differences in riparian conditions (Meyer et al. Citation2007). Moreover, even though such streams individually constitute only a small fraction of a large watershed's area, collectively they constitute most of that area (Richardson and Danehy Citation2007). Thus, conditions in small streams will collectively affect downstream habitats (Progar and Moldenke Citation2002). Unfortunately, despite their abundance and importance, headwater streams are often unknown, unmapped, ignored, and very frequently and easily disturbed (Meyer and Wallace Citation2001; Meyer et al. Citation2007).
The river continuum concept (Vannote et al. Citation1980) describes a predictable pattern of gradual changes in river morphometrics, physicochemistry, and both vertebrate and invertebrate assemblages as width increases and the river interacts with its riparian corridor. Anthropogenic disturbances, however, may disrupt this continuum, particularly in small streams. Agriculture, for example, often leads to removal of riparian canopy cover with subsequent loss of coarse allochthonous input (Warren et al. Citation2007), increased deposition of fine particulate organic matter (Moore and Wondsell Citation2005; MacDonald and Coe Citation2007), and fundamental changes in water physicochemistry (Gravelle and Link Citation2007; Webb et al. Citation2008). Riparian disturbance, with subsequent changes in nutrient and sediment input, has been repeatedly found to be the most widespread stressor of streams in the US generally, in the Plains and Lowlands region of the US, and in Michigan (Paulsen et al. Citation2008; Wang et al. Citation2008).
It is difficult to separate the habitat loss and sediment input components of riparian disturbance as they frequently occur together. Recent studies of agricultural watersheds, however, have noted positive correlations between the amount of intact riparian vegetation and the biological diversity in the adjacent streams (Rios and Bailey Citation2006; Urban et al. Citation2006). More specifically, Houghton et al. (Citation2011a) found that sites in a southern Michigan agricultural stream protected by riparian canopy had three times the number of adult caddisfly species as sites without canopy, even though physicochemistry remained unchanged and typical of agricultural streams.
These studies suggest the hypothesis that both riparian habitat loss and changes in sediment input are individually important for affecting stream biota. The purpose of our study, therefore, was to test this hypothesis by determining the effects of a loss of riparian canopy on the continuity of an otherwise undisturbed small stream. We also assessed if such a stream would return to predicted continuity upon return of canopy cover at downstream sites.
Methods
Study site
Fairbanks Creek is an outflow of a 20-ha mesotrophic lake located in the northwestern portion of the Lower Peninsula of Michigan, USA (N 44°02′, W 85°39′). After leaving the lake, the stream flows indistinctly through a white cedar (Thuja occidentalis) swamp with substantial groundwater upwelling until forming a distinct channel with a silt substrate approximately 100 m downstream. From there it flows through a dense forest dominated by white cedar, with some beech (Fagus grandifolia), red oak (Quercus rubra), and silver maple (Acer saccharinum), where it increases in velocity and develops sandier substrate. Downstream, it enters a meadow habitat without canopy cover. Although the meadow has not been cultivated in nearly 15 years, its banks remain devoid of canopy except for some grasses and sedges (Carex spp.). The meadow habitat runs for approximately 100 m of stream length. Downstream of the meadow, the riparian zone is dominated by a mixed hardwood forest. Except for one road crossing, the entirety of our study area, as well as the overall watershed, is protected by the 300-ha G.H. Gordon Biological Station (Hillsdale College).
Five sites on Fairbanks Creek were sampled during this study. Site 1 was the upper forested portion of the creek where it first formed a channel below the lake; site 2 was where it developed a sandy substrate; site 3 was in the meadow region; and sites 4 and 5 were in the forested area downstream of the meadow (Figure ). All five sites were within a reach of 800 m total stream length. Stream width ranged from 1 to 2 m.
Site conditions
Extent of canopy cover at each site was determined from digital images taken directly above the stream at a height of 2 m. Six images were taken along 20 m of stream length at each site during a single date in early September 2012, approximately one month before leaf fall. Images were printed on grid paper, and the percentage of overhead canopy relative to visible sky determined.
Sunlight intensity was determined on a series of days in early September 2012 using a light intensity meter (Model 753-002C, SKC, Inc., www.skcinc.com). Twelve samples were taken at water level from representative locations around each site during sunny conditions between 1400 and 1600 h. Another 12 light measurements were collected during overcast conditions, also between 1400 and 1600 h.
The volume of in-stream woody debris was estimated during mid-September 2012 along transects between stream banks. Six transects were assigned at each site by throwing a spool of twine across the stream and then tying both ends to riparian vegetation to hold it steady. The diameters of all pieces of woody debris in the stream and intercepted by each transect were recorded. The overall volume (V) of debris was calculated for each transect using the formula:
where L is the length of the transect and d is the diameter of each individual piece of wood, i (Van Wagner Citation1968; Wallace and Benke Citation1984).
Physicochemical and biological sampling
Physicochemical measurements were made at each site from May to October 2012. Six measurements were made at each site on each date. Specific conductance (ECTestr Low, www.eutechinst.com), pH (AccuMet AP61, www.fishersci.com), dissolved oxygen (YSI-55, www.ysi.com), and temperature (YSI-55, www.ysi.com) measurements were all made on-site. All measurements were made within 2 h of each other to minimize diel fluctuations.
Benthic macroinvertebrates were sampled using Hess samplers with 0.3 m2 areas (Barbour et al. Citation1999). Six Hess samples were taken from each site within a diversity of habitats. Sites 1–3 were sampled in 2010. Sites 1–5 were sampled in 2012. All benthic specimens were identified to the lowest identifiable taxon, typically genus, after Hilsenhoff (Citation1995).
Adult caddisflies were sampled using ultraviolet light traps, which consisted of an 8-watt ultraviolet light placed over a white pan filled with 70% EtOH. Each trap was placed within 2 m of a sampling site at dusk and retrieved approximately 2 h later. To standardize weather conditions, samples were collected only if the peak daytime temperature was >22°C, dusk temperature was >13°C, and there was no noticeable wind or precipitation at dusk. For an in-depth discussion of this technique, see Houghton (Citation2004a). The stream was sampled approximately weekly from June through August 2010 from sites 1–3, for a total of 13 samples per site. Sites 1–5 were sampled approximately weekly from mid-June to mid-August 2012, for a total of nine samples per site. All adult specimens were identified to species after Houghton (Citation2012), except for females of Hydroptilidae, Hydropsychidae, and Polycentropodidae, which lack the characters necessary for doing so. Such specimens were not included in any analyses.
Fish were sampled using unbaited, wire-mesh minnow traps with openings of 3.5 cm. Traps were left overnight at each site for a succession of 6 days, interspersed by 1 day of no trapping, yielding 6 samples at each site. Sites 1 through 3 were sampled in May 2010. Sites 1 through 5 were sampled in May 2012. Fish were identified to species after Page and Burr (Citation1991). All fish were released at the collecting site.
Data analyses
Specimens of both benthic invertebrates and adult caddisflies were placed into trophic functional groups following Merrit et al. (Citation2008). Algal piercers were considered gathering collectors in analyses. Mean percentages of the functional groups informative of stream condition at each site: scrapers, shredders, and filtering collectors (e.g., Allan Citation1995; Houghton Citation2007), were compared to each other by a one-way analysis of variance (ANOVA). Mean percentage of canopy cover, total light intensity, volume of woody debris, number of fish taxa, and number of fish species per site were all also compared using a one-way ANOVA. Mean water physicochemical values were compared using a two-way ANOVA to determine differences among both sampling sites and sampling days. Any percentage data were transformed through an arcsine function before analysis. Analyses of Variance were performed on ranked data on sets violating parametric assumptions (Zar Citation2007).
Sampling sites in both streams were examined for patterns in their fish, benthic macroinvertebrate, and adult caddisfly assemblages with Detrended Correspondence Analysis (DCA) using the program PC-ORD for Windows® (McCune and Grace Citation2002). For caddisfly and benthic macroinvertebrate data, our DCA analysis was performed on a two-dimensional data matrix of sampling sites by taxa relative abundance values. Relative abundances were determined by counting the number of specimens collected at each site and then coding 0 specimens as ‘0’, 1–10 as ‘1’, 11–100 as ‘2’, 101–1000 as ‘3’, and 1001–10,000 as ‘4’. Since data coding accounted for variation in specimen abundance between sites, it was a more powerful measure than simple presence or absence data (Feminella Citation2000; Houghton Citation2004a). Coding on a log10 scale mitigated the effects of outlier samples often associated with light-trapping data, as well as the influence of highly abundant species (Cao et al. Citation1997; Houghton Citation2004a; Houghton et al. Citation2011b). Due to lower numbers, fish specimens were expressed as their actual abundances in DCA analyses. All taxa were weighted equally in each analysis.
Results
Mean overhead canopy cover was higher at sites 1, 2, 4, and 5 than at site 3 (one-way ANOVA with post hoc Tukey test, df = 4, F = 24.1, p < 0.001; Figure ). Sunlight intensity was lower at the forested sites than at the non-forested site (one-way ANOVA with post hoc Tukey test, df = 4, F = 13.5, p < 0.001; Figure ). Mean volume of in-stream woody debris was higher at the forested sites than at the non-forested site (one-way ANOVA with post hoc Tukey test, df = 4, F = 8.6, p < 0.001; Figure ).
Figure 2 Mean (+SE) values and associated p values for (a) percentage of area above the stream covered by riparian canopy, and (b) the volume of wood (m2) estimated at each stream site. Lowercase letters denote statistically distinct groups (one-way ANOVA with post hoc Tukey test)
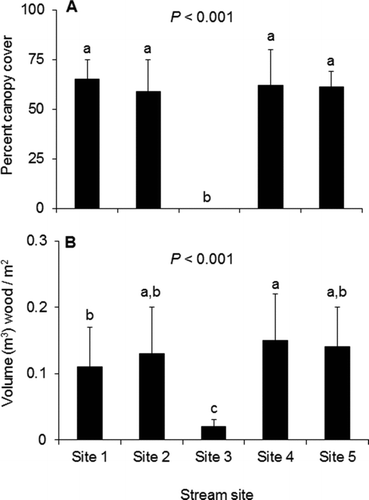
Figure 3 Mean (+SE) sunlight intensity and associated p values measured above each stream site during overcast and sunny conditions. Lowercase letters denote statistically distinct groups (one-way ANOVA with post hoc Tukey test)
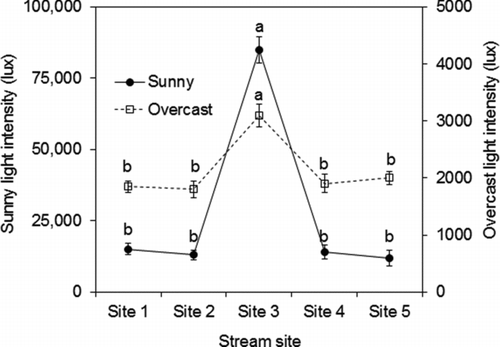
Temperature and dissolved oxygen both exhibited differences between sites and between dates (Figure ). Seasonal temperatures followed a binomial trend, increasing from May to July, and then decreasing into September; sites 1 and 2 had lower temperatures than sites 3 through 5 (two-way ANOVA with post hoc Tukey, df = 16, 4, F 4,16 = 42.9, 55.7; p < 0.001 for both). Dissolved oxygen exhibited a reciprocal seasonal trend, although seasonal overlap between sites rendered site distinction impossible (two-way ANOVA with post hoc Tukey, df = 16, 4, F = 22.1, 7.6; p < 0.001 for both). No difference in pH was detected either among sites or among dates (two-way ANOVA, df = 16, 4; F = 0.9, 1.6; p = 0.6, 0.45). Specific conductance increased throughout the summer (p < 0.001) and exhibited no difference among sites (p = 0.7) (two-way ANOVA, df = 16, 4. F = 87.3, 0.5) (Figure ).
Figure 4 Mean values, trendlines, and associated p values for (a) specific conductance and (b) pH (linear trendline), (c) dissolved oxygen and (d) temperature (binomial trendline) for the five sites of Fairbanks Creek from May to October 2012 (two-way Analysis of Variance). Error bars omitted for clarity
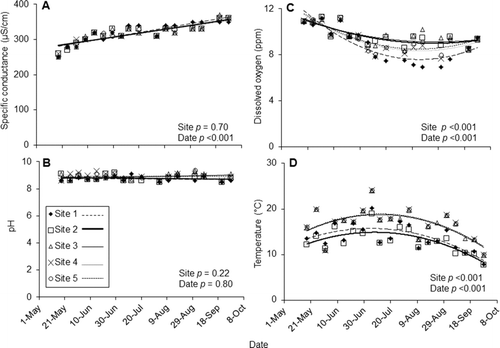
Assemblages of fish, adult caddisflies and benthic invertebrates all appeared different between forested and non-forested sites (Figure ). Assemblages from 2010 and 2012 did not appear different from each other. During both years, mean relative abundance of benthic macroinvertebrate shredders was higher at the forested sites and lower at the non-forested site, whereas filtering collector and scraper abundances were lower at forested sites and higher at the non-forested site (Figure ). Adult caddisfly assemblages exhibited the same trend, except that scraper abundance was not different between sites. The most abundant individual caddisfly species at the forested sites were all shredders, whereas filtering collector species were most abundant at the non-forested site (Table ).
Table 1 The 10 most abundant caddisfly species from five sites in Fairbanks Creek, Michigan, USA from 2010 and 2012 combined. Sites 1 and 2 are forested and the furthest upstream, site 3 is non-forested, and sites 4 and 5 are downstream of the non-forested site.
Figure 5 Detrended correspondence analysis ordination of (a) fish, (b) adult caddisfly, and macroinvertebrate benthic invertebrate samples from Fairbanks Creek during 2010 and 2012
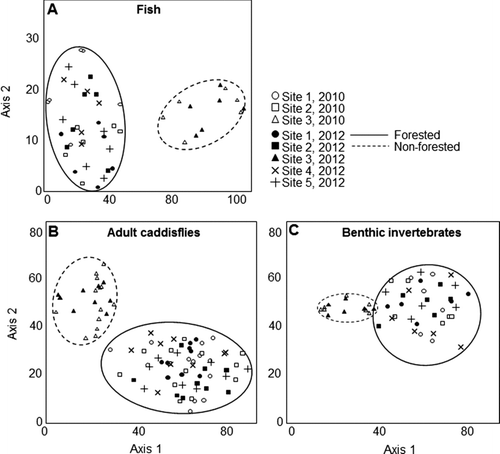
Fish species richness and specimen abundance were both highest at the non-forested site (Figure ). The forested sites contained 90–100% brook, brown, and rainbow trout. The non-forested site had more warm-water species, such as bluegill (Lepomis macrochirus) and central mudminnow (Umbra limi) (Table ). Trout still comprised approximately 40% of the fauna of the non-forested site.
Table 2 Relative abundances of fish from the five sites in Fairbanks Creek from 2010 and 2012 combined.
Figure 6 Mean (+SE) values and associated p values of benthic invertebrate and adult caddisfly A: filtering collectors, B: scrapers, and C: shredders from 2010, and D: filtering collectors, E: scrapers, and F: shredders from 2012. Lowercase letters denote statistically distinct groups (one-way ANOVA with post hoc Tukey test). n.s. = not significant
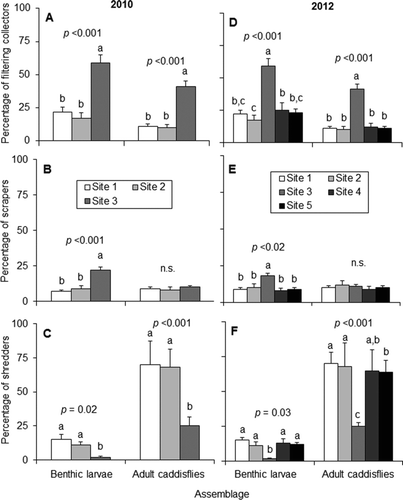
Discussion
While there have been many critiques and modifications of stream continuity theory (most notably Thorp et al. Citation2006), small woodland streams such as Fairbanks Creek do tend to follow the general predicted patterns if they are undisturbed. Conversely, disturbed small woodland streams do not follow predicted patterns. Instead, they typically exhibit abundant filtering collectors and warm-water fish species, and few shredders (Pringle et al. Citation1993; Delong and Brusven Citation1998; Houghton Citation2006, Citation2007). The abundance of filtering collectors alone accounted for nearly 70% of the watershed disturbance variation of small and medium streams in Minnesota (Houghton Citation2006).
In Fairbanks Creek, the lack of riparian canopy did not appear to have important effects on water chemistry, as indicated by our static pH and specific conductance values between sites. These two variables have been found to explain large amounts of the watershed disturbance variation between sites of watersheds in New Jersey, and were also associated with differences in the assemblages of organisms (Zampella and Laidig Citation1997; Dow and Zampella Citation2000). Specific conductance, in particular, is often used as a preliminary indicator of nutrient, sediment, and organic matter concentrations. Such concentrations accumulate naturally in larger rivers or anthropogenically in disturbed streams (Allan Citation2004). Michigan streams disturbed by agriculture have levels two to three times that of Fairbanks Creek (Castillo et al. Citation2000; Houghton et al. Citation2011a). Our low specific conductance values, as well as the lack of difference in both variables between study sites, suggested no important differences in natural or anthropogenic sediment input throughout the continuum of the stream.
The increase in temperature at the downstream sites was almost certainly caused by a lack of canopy cover and resulting increased sunlight penetration to the stream surface at site 3 (Richardson and Danehy Citation2007). Although the sedges and grasses at site 3 may have provided some limited shading from low-angle sunlight, the stream was otherwise completely exposed (Figure ). Sunlight penetration to the stream surface at the other sites was greatly limited due to the high prevalence of riparian canopy cover. Sites 4 and 5 probably maintained the warmer temperatures from site 3 due to their close proximity (approximately 100 m) to site 3. Dissolved oxygen typically exhibits a reciprocal relationship with temperature; thus, it was lower where temperature was higher (Allan Citation1995).
The assemblages of organisms in Fairbanks Creek suggested biological discontinuity due to the loss of riparian canopy. The gradual or even unnoticeable change in the downstream fauna (from sites 1 and 2 to site 3) that would be predicted by typical continuity was instead an abrupt change. Within approximately 600 m of stream length, fish and invertebrate assemblages went from those predicted in the headwaters: high abundance of shredders, low abundance of filtering collectors and scrapers, and high abundance of trout; to those predicted in a medium-large river: low abundance of shredders, high abundance of filtering collectors and shredders, and higher abundance of warm-water fish. More specifically, the relative abundances of adult caddisfly shredders and filtering collectors at the <2 m wide, non-forested site corresponded to those of 10–20 m wide Minnesota rivers (Houghton Citation2007). When the canopy returned 100 m downstream, however, continuity was reversed and the fauna changed back to that of a headwaters stream.
Even though Fairbanks Creek was dominated by filtering collectors at the non-forested site, these assemblages did not appear to indicate an ecosystem disturbed by excess sediment or nutrient input. Typically, a small stream with high levels of anthropogenic disturbance has an abundance of the specific filtering collector species that are normally found in large rivers. These species increase in disturbed small streams due to an abundance of fine particulate organic matter that they can utilize as a food source (Allan Citation1995; Barbour et al. Citation1999; Allan Citation2004; Houghton Citation2007). For example, the hydropsychid species Cheumatopsyche campyla, Hydropsyche simulans, and Potamyia flava are all typically found in large rivers of Minnesota, and only rarely in undisturbed small streams (Houghton Citation2012). In small agricultural streams, however, these same species were abundant and constituted significant indicators of disturbance statewide (Houghton Citation2004b). In Fairbanks Creek, all of the abundant filtering collectors (Table ) were those naturally found in small woodland streams of the north-central US (Houghton et al. Citation2011b; Houghton Citation2012), albeit at lower relative abundances. Common Fairbanks Creek filtering collector species like Brachycentrus americanus and Dolophilodes distinctus are highly intolerant of organic pollution (Hilsenhoff Citation1987), suggesting a lack of such input even at the non-forested site. Moreover, these species feed on the larger (1–4 mm) particles typically dislodged by fast-moving headwaters current, not the small (<0.25 mm) particles associated with large rivers (Cummins Citation1974; Boiling et al. Citation1975; Wiggins Citation1996).
Thus, instead of an increase in small-particle filtering collectors associated with large rivers and polluted small streams, our non-forested site suggested a shift in the relative abundances of invertebrate assemblages already present, due to the lack of canopy cover and its associated effects. These effects primarily appeared to be an increase in sunlight penetration and, especially, the loss of coarse allochthonous woody debris input. In the case of the former, increased sunlight penetration at the non-forested site probably increased growth of the periphyton consumed by scrapers, although we did not quantify this growth. In the case of the latter, the decreased volume of in-stream woody debris provided less food for shredders at the non-forested site. Since we did not quantify the volume of allochthonous leaves, we may have actually underestimated the difference in CPOM between the forested and non-forested sites. It is difficult to judge the importance of temperature and dissolved oxygen as contributing effects, since warm temperatures were maintained at sites 4 and 5, yet faunal assemblages at these sites were similar to those of the colder sites. The normalization of continuity within a short distance after canopy returned also suggested the local effect of small-scale canopy loss, rather than systemic disturbance, such as sediment input or canopy removal on a large scale.
Our results also suggest relative strengths and weaknesses of using benthic macroinvertebrates, adult caddisflies, and fish to assess stream conditions. In the case of benthic macroinvertebrates, the difficulty of sampling microhabitats (e.g., large woody debris) representatively may lead to samples that are non-representative of the overall assemblage. In our study, the ease of collecting gathering collectors in Hess samples taken from shallow riffles led to samples dominated by these ubiquitous and non-informative taxa, thus decreasing the ‘signal’ relative to the ‘noise’ of a sample (Cao and Hawkins Citation2011; Whiting et al. Citation2011). In the case of adult caddisflies, the lack of response from the scraper functional group has been noted in previous studies (Houghton Citation2006, Citation2007). While scraper taxonomic richness may be proportional within the Trichoptera (e.g., Wiggins Citation1996), scraper specimens are not abundant in blacklight samples relative to filtering collectors or shredders, or in benthic samples compared to scraper taxa of other orders (Houghton Citation2011a). Thus, the metric may not be abundant enough to be informative of stream conditions when using Trichoptera exclusively. In the case of fish, minnow trap placements in specific microhabitats, behavior of the fish species, and size of the minnow trap openings all effect the number and relative abundance of species caught (Pot et al. Citation1984; He and Lodge Citation1990; Rozas and Minello Citation1997; Layman and Smith Citation2001). In particular, larger piscivorous fish that can be informative about stream conditions are often under-sampled by minnow traps (e.g., Barbour et al. Citation1999).
All of our assemblages individually and collectively reinforce the assertion that a loss of riparian habitat from more than 10 years ago can still impact stream biota (Harding et al. Citation1998). The precise mechanisms of this impact – such as decrease in allochthonous CPOM, increase in allochthonous FPOM, increased growth of periphyton, changes in substrate composition, increase in flooding, or competition between functional groups – still need further study. Likewise, further study is needed on the potential impacts of headwater habitat loss on overall river systems, such as the loss of cold-water refuge habitats; the loss of fish spawning grounds; and the loss of coarse allochthonous input, processing, and export (Cuffney et al. Citation1990). Ultimately, the biotic integrity of entire river systems is greatly dependent on the collective impacts to individual small streams such as Fairbanks Creek (Meyer et al. Citation2007).
Acknowledgements
Research costs were paid by the Mossberg Conservation Fund, a Hillsdale College LAUREATES grant to JLW, and by Hillsdale College Biology Department funds. We thank Connie Brandin, Chris Bowyer, Kelsey Brakel, Ron McCarty, Mark Nussbaum, Sarah Rogers, and Logan Shoup for assistance in the field and laboratory. A collecting permit was provided by Thomas Goniea of the Michigan Department of Natural Resources and Environment. The valuable comments of Matt Whiles, Rebecca Houghton, and two anonymous reviewers improved earlier versions of the manuscript. This is paper #7 of the G.H. Gordon BioStation Research Series.
References
- Allan , J D . 1995 . Stream ecology: structure and function of running water , London : Chapman and Hall .
- Allan , J D . 2004 . Landscapes and riverscapes: the influence of land use on stream ecosystems . The Annual Review of Ecology, Evolution, and Systematics , 35 : 257 – 284 . doi: 10.1146/annurev.ecolsys.35.120202.110122
- Barbour , M T , Gerritsen , J , Snyder , B D and Stribling , J B . 1999 . Rapid bioassessment protocols for use in streams and rivers: periphyton, benthic macroinvertebrates, and fish , 2nd ed , Washington (DC) : Office of Water, U.S. Environmental Protection Agency . EPA 841-B-99-002
- Boiling , R H , Goodman , E D , VanSickle , J A , Zimmer , J O , Cummins , K W , Petersen , R C and Reice , S R . 1975 . Towards a model of detritus processing in a woodland stream . Ecology , 56 : 141 – 151 . doi: 10.2307/1935306
- Cao , Y , Bark , A W and Williams , W P . 1997 . A comparison of clustering methods for river benthic community analysis . Hydrobiologia , 347 : 25 – 40 . doi: 10.1023/A:1002938721135
- Cao , Y and Hawkins , C P . 2011 . The comparability of bioassessments: a review of conceptual and methodological issues . Journal of the North American Benthological Society , 30 : 680 – 701 . doi: 10.1899/10-067.1
- Castillo , M M , Allan , J D and Brunzell , S . 2000 . Nutrient concentrations and discharges in a Midwestern agricultural catchment . Journal of Environmental Quality , 29 : 1142 – 1151 . doi: 10.2134/jeq2000.00472425002900040015x
- Cuffney , T F , Wallace , J B and Lughart , G J . 1990 . Experimental evidence quantifying the role of benthic invertebrates in organic matter dynamics of headwater streams . Freshwater Biology , 23 : 281 – 299 . doi: 10.1111/j.1365-2427.1990.tb00272.x
- Cummins , K W . 1974 . Structure and function of aquatic ecosystems . BioScience. , 24 : 631 – 641 . doi: 10.2307/1296676
- Delong , M D and Brusven , M A . 1998 . Macroinvertebrate community structure along the longitudinal gradient of an agriculturally impacted stream . Environmental Management. , 22 : 445 – 457 . doi: 10.1007/s002679900118
- Dow , C L and Zampella , R A . 2000 . Specific conductance and pH as indicators of watershed disturbance in streams of the New Jersey pinelands, USA . Environmental Management. , 26 : 437 – 445 . doi: 10.1007/s002670010101
- Feminella , J W . 2000 . Correspondence between stream macroinvertebrate assemblages and 4 ecoregions of the southeastern United States . Journal of the North American Benthological Society. , 19 : 442 – 461 . doi: 10.2307/1468106
- Gravelle , J A and Link , T E . 2007 . Influence of timber harvesting on headwater peak temperatures in a northern Idaho watershed . Forest Science. , 53 : 189 – 205 .
- Harding , J S , Benfield , E F , Bolstad , P V , Helfman , G S and Jones , E BP . 1998 . Stream biodiversity: the ghost of land use past . Proceedings of the National Academy of Sciences of the United States of America , 95 : 14843 – 14847 . doi: 10.1073/pnas.95.25.14843
- He , X and Lodge , D M . 1990 . Using minnow traps to estimate fish population size: the importance of spatial distribution and relative species abundance . Hydrobiologia. , 190 : 9 – 14 . doi: 10.1007/BF00020683
- Hilsenhoff , W L . 1987 . An improved biotic index of organic stream pollution . Great Lakes Entomologist. , 20 : 31 – 39 .
- Hilsenhoff , W L . 1995 . Aquatic insects of Wisconsin: generic keys and notes on biology, ecology and distribution , Madison (WI) : Wisconsin department of Natural Resources . Technical bulletin, Wisconsin Dept. of Natural Resources, No. 89
- Houghton , D C . 2004a . Minnesota caddisfly biodiversity (Insecta: Trichoptera): delineation and characterization of regions . Environmental Monitoring and Assessment. , 95 : 153 – 181 . doi: 10.1023/B:EMAS.0000029890.07995.90
- Houghton , D C . 2004b . Utility of caddisflies (Insecta: Trichoptera) as indicators of habitat disturbance in Minnesota . Journal of Freshwater Ecology. , 19 : 97 – 108 . doi: 10.1080/02705060.2004.9664517
- Houghton , D C . 2006 . The ability of common water quality metrics to predict habitat disturbance when biomonitoring with adult caddisflies (Insecta: Trichoptera) . Journal of Freshwater Ecology. , 21 : 705 – 716 . doi: 10.1080/02705060.2006.9664134
- Houghton , D C . 2007 . The effects of landscape-level disturbance on the composition of caddisfly (Insecta: Trichoptera) trophic functional groups: evidence for ecosystem homogenization . Environmental Monitoring and Assessment. , 135 : 253 – 264 . doi: 10.1007/s10661-007-9647-9
- Houghton , D C . 2012 . Biodiversity of Minnesota caddisflies (Trichoptera): identification, distributions, and habitat affinities of the fauna . ZooKeys Special Issues. , 189 : 1 – 389 . doi: 10.3897/zookeys.189.2043
- Houghton , D C , Berry , E A , Gilchrist , A , Thompson , J and Nussbaum , M A . 2011a . Biological changes along the continuum of an agricultural stream: influence of a small terrestrial preserve and use of adult caddisflies in biomonitoring . Journal of Freshwater Ecology. , 26 : 381 – 397 . doi: 10.1080/02705060.2011.563513
- Houghton , D C , Brandin , C M and Brakel , K A . 2011b . Analysis of the caddisflies (Trichoptera) of the Manistee River watershed, Michigan . Great Lakes Entomologist. , 44 : 1 – 15 .
- Layman , C A and Smith , D E . 2001 . Sampling bias of minnow traps in shallow aquatic habitats on the eastern shores of Virginia . Wetlands. , 21 : 145 – 154 . doi: 10.1672/0277-5212(2001)021[0145:SBOMTI]2.0.CO;2
- MacDonald , L H and Coe , D . 2007 . Influence of headwater streams on downstream reaches in forested areas . Forest Science. , 53 : 148 – 168 .
- McCune , B and Grace , J B . 2002 . Analysis of ecological communities , 2nd ed , Gleneden Beach , OR : MjM Software Design .
- Merrit , R W , Cummins , K W and Berg , M B . 2008 . An introduction to the aquatic insects of North America , 4th ed , Dubuque , IA : Kendall/Hunt .
- Meyer , J L , Strayer , D L , Wallace , J B , Eggert , S L , Helfman , G S and Leonard , N E . 2007 . The contribution of headwater streams to biodiversity in river networks . Journal of the American Water Resources Association. , 43 : 86 – 103 . doi: 10.1111/j.1752-1688.2007.00008.x
- Meyer , J L and Wallace , J B . 2001 . “ Lost linkages and lotic ecosystems: rediscovering small streams ” . In Ecology: achievement and challenge , Edited by: Huntly , N J , Levin , S and Press , M C . 295 – 317 . Malden , MA : Blackwell Science .
- Moore , R D and Wondsell , S M . 2005 . Physical hydrology and the effects of forest harvesting in the Pacific Northwest: a review . Journal of American Water Resources Association. , 41 : 763 – 784 .
- Page , L M and Burr , B M . 1991 . A peterson field guide to the freshwater fishes , 2nd edition , Boston , MA : Houghton Mifflin Harcourt .
- Paulsen , S G , Mayio , A , Peck , D V , Stoddard , J L , Tarquinio , E , Holdsworth , S M , Van Sickle , J , Yuan , L L , Hawkins , C P Herlihy , A T . 2008 . Condition of stream ecosystems in the U.S.: an overview of the first national assessment . Journal of the North American Benthological Society. , 27 : 812 – 821 . doi: 10.1899/08-098.1
- Pot , W D , Noakes , L G , Ferguson , M M and Coker , G . 1984 . Quantitative sampling of fishes in a simple system: failure of conventional methods . Hydrobiologia. , 114 : 249 – 254 . doi: 10.1007/BF00031876
- Pringle , C , Vellidis , G , Heliotis , F , Bandacu , D and Cristofor , S . 1993 . Environmental problems for the Danube delta . American Scientist. , 81 : 350 – 361 .
- Progar , R A and Moldenke , A R . 2002 . Insect production from temporary and perennial flowing headwater streams in western Oregon . Journal of Freshwater Ecology. , 17 : 391 – 407 . doi: 10.1080/02705060.2002.9663913
- Richardson , J S and Danehy , R J . 2007 . A synthesis of the ecology of headwater streams and their riparian zones in temperate forests . Forest Science. , 53 : 131 – 147 .
- Rios , S L and Bailey , R C . 2006 . Relationship between riparian vegetation and stream benthic communities at three spatial scales . Hydrobiologia. , 553 : 153 – 160 . doi: 10.1007/s10750-005-0868-z
- Rozas , L P and Minello , T J . 1997 . Estimating densities of fishes and decapod crustaceans in shallow estuarine habitats: a review of sampling design with a focus on gear selection . Estuaries. , 20 : 199 – 213 . doi: 10.2307/1352731
- Thorp , J H , Thoms , M C and Delong , M D . 2006 . The riverine ecosystem synthesis: biocomplexity in river networks across space and time . River Research and Applications. , 22 : 123 – 147 . doi: 10.1002/rra.901
- Urban , M C , Skelly , D K , Burchsted , D , Price , W and Lowry , S . 2006 . Stream communities across a rural–urban landscape gradient . Diversity and Distributions. , 12 : 337 – 350 . doi: 10.1111/j.1366-9516.2005.00226.x
- Van Wagner , C E . 1968 . The line intersect method in forest fuel sampling . Forest Science. , 14 : 20 – 26 .
- Vannote , R L , Minshall , G W , Cummins , K W , Sedell , J R and Cushing , C E . 1980 . The river continuum concept . Canadian Journal of Fisheries and Aquatic Sciences. , 37 : 130 – 137 . doi: 10.1139/f80-017
- Wallace , J B and Benke , A C . 1984 . Quantification of wood habitat in subtropical coastal plain streams . Canadian Journal of Fisheries and Aquatic Sciences. , 41 : 1643 – 1652 . doi: 10.1139/f84-203
- Wang , L , Brenden , T , Seelbach , P , Cooper , A , Allan , D , Clark , R and Wiley , M . 2008 . Landscape based identification of human disturbance gradients and reference conditions for Michigan streams . Environmental Monitoring and Assessment. , 141 : 1 – 17 . doi: 10.1007/s10661-006-9510-4
- Warren , D R , Bernhardt , E S , Hall , R O and Likes , G E . 2007 . Forest age, wood, and nutrient dynamics in headwater streams of the Hubbard Brook Experimental Forest, NH . Earth Surface Processes and Landforms. , 32 : 1154 – 1163 . doi: 10.1002/esp.1548
- Webb , B W , Hannah , D M , Moore , R D , Brown , L E and Nobilis , F . 2008 . Recent advances in stream and river temperature research . Hydrological Processes. , 22 : 902 – 918 . doi: 10.1002/hyp.6994
- Whiting , D P , Whiles , M R and Stone , M L . 2011 . Patterns of macroinvertebrate production, trophic structure, and energy flow along a tallgrass prairie stream continuum . Limnology and Oceanography. , 56 : 887 – 898 . doi: 10.4319/lo.2011.56.3.0887
- Wiggins , G B . 1996 . Larvae of the North American caddisfly genera (Trichoptera) , 2nd ed , Ontario : University of Toronto Press .
- Zampella , R A and Laidig , K J . 1997 . Effect of watershed disturbance on pinelands stream vegetation . Journal of the Torrey Botanical Society. , 124 : 52 – 66 . doi: 10.2307/2996598
- Zar , J H . 2007 . Biostatistical analysis , 5th ed , Englewood Cliffs , NJ : Prentice-Hall .