Abstract
Topeka shiners (Notropis topeka) were historically abundant throughout many Great Plains streams but their abundance and distribution declined and the species was listed as federally endangered in 1999. However, few studies have examined how Topeka shiner populations are linked to biotic and abiotic factors. Thus, the objectives of this study were to identify trends in Topeka shiner distribution from 1995 to 2008 and determine which fish assemblage and habitat factors were most associated with Topeka shiner distribution in Kansas streams. Fish and in-stream habitat metrics were collected from 34 sites in northeast Kansas in 1995, 2003, and 2008. Stepwise discriminant function analyses (DFA) were used to determine if fish assemblage percent composition and in-stream and landscape habitat factors could be used to differentiate among years and between sites with and without Topeka shiners. Higher percent composition of rosyface shiner (Notropis rubellus) was generally indicative of sites with Topeka shiners whereas higher relative abundance of central stoneroller (Campostoma anomalum) and largemouth bass (Micropterus salmoides) were associated with sites without Topeka shiners. Sites with Topeka shiners also tended to have more gravel substrate and greater mean stream length, whereas sites without Topeka shiners generally had higher proportional impoundment area and proportional urban land area. These findings suggest that anthropogenic alteration of habitat in and around streams with Topeka shiners may facilitate their declines and establishment of non-native or generalist fishes. Our results may help managers identify habitats with the greatest need for protection or restoration and may help protect Topeka shiner populations from further population declines.
Introduction
Humans have altered many freshwater ecosystems throughout the world (Strayer and Dudgeon Citation2010) and these changes have homogenized habitats and impacted biota of these ecosystems. Human-mediated changes to freshwaters are among the leading causes of declines and extirpations of native fishes (Warren et al. Citation2000; Jelks et al. Citation2008; Strayer and Dudgeon Citation2010). Flow manipulation (Poff et al. Citation1997; Bunn and Arthington Citation2002), habitat fragmentation (Nilsson et al. Citation2005; Perkin and Gido Citation2011), water quality declines (Dudgeon et al. Citation2006; Geist Citation2011), and the introduction of non-native species (Rahel Citation2000; Jelks et al. Citation2008) have been linked to declines in the distribution and abundance of native species and biodiversity of freshwater ecosystems. As a result of human actions, many freshwater organisms are imperiled and biodiversity of freshwater ecosystems is declining worldwide (Xenopoulos et al. Citation2005; Dudgeon et al. Citation2006).
Anthropogenic alterations are especially pervasive in streams of the Great Plains in central United States. Many streams in this region have dynamic hydrology, including frequent flooding and drying (Dodds et al. Citation2004), but Great Plains fishes have adapted to persist in areas with dynamic flows in part by adapting to depend on specific habitats (e.g. refugia) to survive disturbances (Bramblett et al. Citation2005). Suitable reproductive conditions must also return following a disturbance to ensure fish can reproduce before the next disturbance (Fausch and Bestgen Citation1997). Human alterations including the construction of impoundments, agricultural water withdrawals, and eutrophication from run-off associated with row-crop agriculture have limited the abundance and quality of these habitats (Dodds et al. Citation2004). As a result of anthropogenic actions, the distribution and abundance of many species, including Topeka shiner (Notropis topeka) have declined and the biota of these streams are among the most endangered in the United States (Dodds et al. Citation2004).
The Topeka shiner is an endangered cyprinid native to small streams throughout the Great Plains including Iowa, Kansas, Minnesota, Missouri, Nebraska, and South Dakota (Minkley and Cross Citation1959; Tabor Citation2002; Wall and Berry Citation2004), but their abundance has declined and their distribution has been reduced to about 10% of the original size (Cross and Collins Citation1995; Schrank et al. Citation2001; Tabor Citation2002). These declines have been attributed to a variety of human-mediated habitat changes including agricultural runoff (Cross and Moss Citation1987; Pflieger Citation1997), impoundments and habitat fragmentation (Pflieger Citation1997; Schrank et al. Citation2001; Bouska and Paukert Citation2010), urban development (Tabor 2002), and the introduction of non-native species including largemouth bass (Micropterus salmoides) (Schrank et al. Citation2001; Mammoliti Citation2002; Gido et al. Citation2004).
Identifying abiotic and biotic factors associated with species occurrence (e.g. presence or absence) is crucial for developing successful conservation and management strategies for imperiled species (Poff Citation1997). However, few studies have quantitatively examined the relationship between Topeka shiner distribution and abiotic and biotic stream characteristics. In one of the only quantitative examinations of the role of abiotic and biotic stream characteristics on Topeka shiner distribution, Schrank et al. (Citation2001) found that Topeka shiner extirpation in Kansas streams was associated with increase in the relative abundance of largemouth bass, greater number of small impoundments in the watershed, and larger pool lengths. These findings provided valuable information for the conservation and management of Topeka shiner populations in Kansas. However, they only offer insight based on one summer of sampling, and the relationship between abiotic and biotic characteristics and species presence is rarely static, often varying both spatially and temporally (Allen and Starr Citation1982; Poff Citation1997). Additionally, Schrank et al. (Citation2001) relied on species richness and diversity indices to examine the relationship between fish assemblage and Topeka shiner occurrence. Whereas indices are helpful in summarizing data and comparing fish assemblages, they provide little insight on how assemblages vary or the species responsible for such variation (Kwak and Peterson Citation2007).
In this study, we evaluated trends in Topeka shiner abundance and distribution over a relatively long temporal scale (1995–2008) and identified abiotic and biotic factors associated with Topeka shiner occurrence. Specifically, we examined the relationship between Topeka shiner occurrence and fish assemblage, in-stream habitat, and landscape-level habitat factors. We then identified the species and habitat metrics linked to the occurrence of Topeka shiner.
Methods
The Mill Creek watershed is located in Wabaunsee County in the Flint Hills region of eastern Kansas (Figure ) and has the highest known extant densities of Topeka shiners in Kansas (Cross and Collins Citation1995; Kerns and Bonneau Citation2002). Streams in this region are characterized by limestone, shale, and gravel substrates; grassland and prairie vegetation; and variable flows (Zimmerman Citation1993; Dodds et al. Citation2004). Historically, the watershed was dominated by tallgrass prairie but is now dominated by cropland and cattle grazing with numerous small impoundments (Dodds et al. Citation2004; Samson et al. Citation2004).
Figure 1 Map of the Mill Creek watershed with the locations of sites sampled in 1995 (diamond), 2003 (circle), and 2008 (triangle)
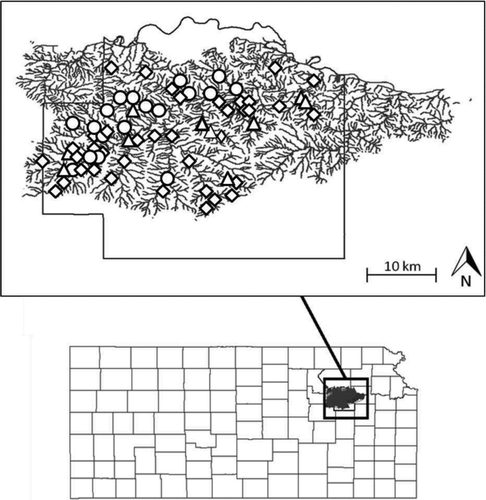
Fish collections and habitat measurements were made at a total of 34 sites in the Mill Creek watershed between May and July of 1995, 2003, and 2008. Sites were selected to ensure they were widely distributed throughout the Mill Creek watershed and to ensure variation in the amount of upstream drainage area controlled by small dams. All sites were less than 1 km long. Landowner permission could not be obtained for all sites located on private property in 2003 and 2008 and, as a result, samples were collected from 34 sites in 1995, 26 sites in 2003, and 28 sites in 2008.
Fishes were collected from three to five pools at each study site by the Kansas Department of Wildlife, Parks, and Tourism Stream Assessment and Monitoring Program using a 6.1 m × 1.2 m (3.2 mm mesh) seine. At least three seine hauls were conducted in each pool. To ensure fish communities were accurately sampled, additional seine hauls were performed in large pools until no new species were collected. All captured fishes were identified, enumerated, and released.
In-stream habitat attributes were measured along three evenly spaced transects at each pool in 2003 and 2008. Pool depth was quantified to the nearest 1.0 cm at three equidistant points along each transect. Mean depths were calculated for each pool by dividing the sum of all depth measurements for each pool by the number of measurements recorded at each pool. Wetted width of each transect and total pool length were measured to the nearest 0.1 m. Mean wetted width was calculated for each pool by dividing the sum of all width measurements for each pool by the number of width measurements at each pool. Mean wetted widths were multiplied by total pool length to calculate the area of each pool. Substrate was visually estimated using a modified Wentworth scale and the most abundant substrate of silt, sand, gravel, pebble, cobble, or bedrock was recorded for each pool (Cummins 1962). Percent dominant substrate was calculated for each site by dividing the number of pools at a site with a given substrate by the total number of pools sampled at a site. Wetted width and pool length were the only in-stream habitat characteristics measured in 1995; consequently, analyses involving in-stream habitat data were only calculated using data collected in 2003 and 2008.
Landscape-level metrics were examined for each site at the catchment level and calculated for 2003 and 2008 using ArcGIS 10.0 (ESRI, Redlands, CA). Catchment boundaries were calculated using the USGS National Hydrography Dataset (NHD). The small impoundments layer associated with the NHD was used to calculate the number and area of small impoundments in each catchment. Land use, measured as the area of agriculture, grassland, forestland, and urban land, was calculated using the National Landcover Database (2006). To control for variation in catchment size, impoundment area and land use areas were converted to proportional areas of the catchment, and number of impoundments and total road length were converted to densities for each catchment by dividing the respective values by total catchment area.
Data analysis
Sites were differentiated a priori into two groups based on Topeka shiner occurrence (presence or absence) to facilitate comparisons between sites with and without Topeka shiners. Percent composition was calculated for each fish species by dividing the abundance of each species by the total abundance at each site. Prior to analyses, percent compositions (Table ) were arcsine square root transformed and all continuous variables (Table ) were log(x + 1) transformed. Species accounting for <1% of the overall catch among all years of the study were excluded from further analyses except for largemouth bass. As Topeka shiner occurrence was used to determine site status, they were excluded from assemblage analyses. An analysis of variance (ANOVA) was used to examine if mean Topeka shiner relative abundance differed among years. We used a significance level of 0.05 to determine the significance for all analyses.
Table 1 Mean relative abundance (standard errors in parentheses) for fishes representing greater than 1% of the overall catch and largemouth bass. Percent composition for all years combined is given as % Abundance. ‘TS status’ indicates if values were calculated from sites where Topeka shiners were present or sites where they were absent
Table 2 Percent dominant substrate and mean (standard errors in parentheses) for dominant substrate and in-stream, landscape, and anthropogenic habitat factors at sites with and without Topeka shiners in 2003 and 2008. ‘TS status’ indicates if values were calculated from sites where Topeka shiners were present or sites where they were absent
The percent similarity index was calculated to compare fish assemblages between sites with and without Topeka shiner and among years. The percent similarity index uses percent composition values from each site to create similarity index values from 0 (no similar species) to 100 (all species in common; Ludwig and Reynolds Citation1989; Kwak and Peterson Citation2007). Species richness and Shannon diversity indices (Krebs Citation1989) were also calculated for each site to further compare fish assemblages between sites with and without Topeka shiners. Two-way factorial ANOVA was used to determine if mean species richness and diversity differed by site status (presence or absence of Topeka shiners) and among years. We used a chi-square test to determine if the proportion of sites with Topeka shiners varied among years.
We used two stepwise discriminant function analyses (DFA) to determine if (1) variation in fish assemblages and (2) variation in in-stream and landscape habitats could be used to differentiate between sites with or without Topeka shiners. Standardized discriminant coefficients were calculated for both the fish and habitat DFA and used to identify which fish species or habitat variables were most important for differentiating between sites with and without Topeka shiner. Wilks’ lambda was used to identify variable significance for both DFAs.
Results
A total of 48,855 fish representing 31 species, including Topeka shiner, were captured among all three years of sampling. Twenty-two species were captured at least once during each year of sampling. Nine species, including Topeka shiner, accounted for at least 1% of the overall catch (Table ). Common shiner (Luxilus cornutus) was the most abundant species among all years and the five most abundant species accounted for 75.1% of the total capture among years (Table ).
A total of 690 Topeka shiners were captured between 1995 and 2008. Topeka shiner was the ninth most abundant species and represented 1.6% of all the fishes caught (Table ). Mean Topeka shiner relative abundance varied from 0.8% of the catch in 1995 to 2.6% in 2003 but did not differ among years (ANOVA, p = 0.076, Figure ). The proportion of sites where Topeka shiners were captured ranged from 50.0% (17/34) in 1995 to 76.9% (20/26) in 2003, but did not differ among years (chi-square, p = 0.086; Figure ).
Figure 2 (a) Mean Topeka shiner relative abundance (±SE) among the three years of the study. (b) Total number of sites sampled during each year of the study with the number of Topeka shiner present (grey) and Topeka shiner absent (white) indicated for each year
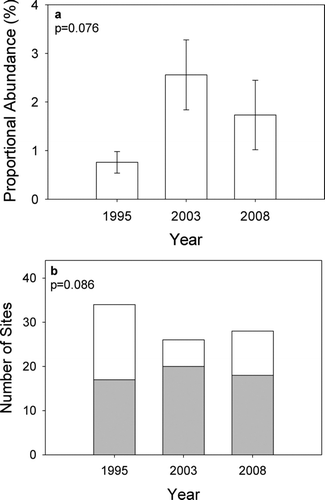
Over the course of the study, fish assemblages at sites with and without Topeka shiner were 80.7% similar as determined using the percent similarity index. Some species were common among sites with and without Topeka shiner, including central stoneroller (Campostoma anomalum), creek chub (Semotilus atromaculatus), and N. cornutus which were all found at more than 90% of all sites. Despite these similarities, fish assemblages at sites where Topeka shiners were present had higher species richness (p < 0.001) and diversity (p < 0.001) compared to sites without Topeka shiners (Table ).
Table 3 Mean (standard errors in parentheses) yearly and overall species richness and species diversity values for sites with and without Topeka shiners. ‘TS status’ indicates if values were calculated from sites where Topeka shiners were present or sites where they were absent
The first axis of the fish assemblage DFA explained 47.8% of the variance between sites with and without Topeka shiner (Figure ). Relative abundance for three (central stoneroller, largemouth bass, and rosyface shiner (Notropis rubellus)) of the eight dominant species were significant contributors for the first axis of the fish assemblage DFA (Table ). The first discriminant primarily differentiated Topeka shiner present sites from Topeka shiner absent sites (Figure ). Relative abundance of rosyface shiner tended to be higher at sites with Topeka shiner whereas relative abundance of central stoneroller and largemouth bass were generally higher at sites without Topeka shiners (Table ).
Table 4 Standardized discriminant function coefficients for species and habitat factors retained in discriminant function analysis (DFA) examining variation in fish assemblage or habitats among years and between sites with and without Topeka shiners. Values in bold are significant and were retained for each axis
Figure 3 Canonical discriminant function analysis of fish assemblage at Topeka shiner present and absent sites in 1995 (circles), 2003 (triangles), and 2008 (squares). Solid symbols represent sites without Topeka shiners whereas hollow shapes indicate sites where Topeka shiners were captured
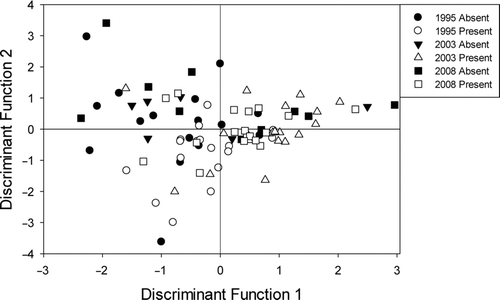
The second axis of the fish assemblage DFA explained the 29.1% of variation between sites with and without Topeka shiner. Bluntnose minnow (Pimephales notatus) and redfin shiner (Lythrurus umbratilis) relative abundance were the only factors that were significant contributors in the second axis of the fish assemblage DFA, which primarily differentiated among years (Table ; Figure ). Relative abundance of redfin shiner tended to be higher in 2003 and 2008 than in 1995. In contrast, relative abundance of bluntnose minnow was higher in 1995 than 2003 and 2008. The misclassification rate of the DFA was relatively high among all samples (56.3%); however, most misclassifications resulted in sites being classified into the correct site status category (presence or absence of Topeka shiners) but with wrong year. Specifically, 82.8% of the misclassified sites where Topeka shiner were present were misclassified as a present site of a different year whereas 66.7% of the misclassified absent sites were misclassified as absent sites of a different year.
The first axis of the habitat DFA explained 56.8% of the variance among sites with and without Topeka shiners and among years (Figure ). Among all habitat variables only bedrock substrate, pebble substrate, and pool length were significant contributors for axis one (Table ) which primarily differentiated between samples collected in 2003 and samples collected in 2008 (Figure ). Pool lengths tended to be longer, bedrock substrate was more common, and pebble substrates were less common in 2008 than 2003. The second axis of the habitat DFA explained 32.3% of the variance among sites and primarily differentiated between sites with and without Topeka shiners (Figure ). In general, sites with Topeka shiners had more gravel substrate and longer pool length whereas sites without Topeka shiners had more urban land use and greater impoundment area (Table ; Figure ). The overall misclassification rate of the habitat DFA was 31.5% (17/54). Misclassifications in the habitat DFA predominantly resulted in sites being classified into the correct year but the wrong site status (Topeka shiner presence or absence). Specifically, 70.6% (12/17) of the misclassified sites were assigned to the correct year but wrong site status.
Figure 4 Canonical discriminant function analysis for the in-stream and landscape level habitat variables at Topeka shiner present and absent sites in 2003 (triangles), and 2008 (squares). Large triangles and squares indicate group centroids for 2003 and 2008 respectively. Solid symbols represent sites without Topeka shiners whereas hollow shapes indicate sites where Topeka shiners were captured
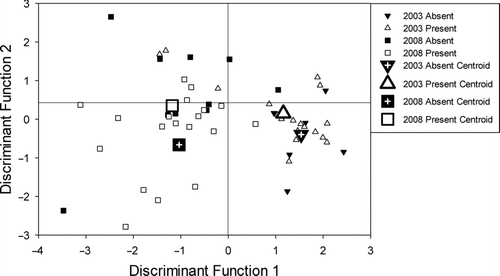
Discussion
Fish assemblages at sites with and without Topeka shiners shared many common species that were found in high abundances at most sites. Many of these species (e.g. common shiner, central stoneroller) were habitat generalists that can tolerate a range of habitat conditions (Pflieger Citation1997). The presence of ubiquitous species, especially generalist species, among all sites is common at relatively small spatial scales and is likely an indication that the streams we sampled had many similar attributes (Jackson et al. Citation2001). Despite these similarities, we did identify differences among fish assemblages at sites with and without Topeka shiners. Specifically, we found that sites with Topeka shiners had higher relative abundances of rosyface shiners whereas sites without Topeka shiners had higher relative abundances of central stonerollers and non-native largemouth bass.
Increased relative abundance of largemouth bass, a top predator, at sites without Topeka shiners is consistent with the findings of other studies (Schrank et al. Citation2001; Winston Citation2002) and is consistent with hypothesized relationships between introduced largemouth bass and declines in susceptible prey (Tabor 2002; Mammoliti Citation2002). Knight and Gido (Citation2005) found that largemouth bass did not preferentially forage on Topeka shiners over other native cyprinids; however, because Topeka shiners generally have lower mean abundances than other native cyprinids it may take less predation pressure to cause their extirpations relative to other cyprinids (Rahel Citation2007). Surprisingly, no largemouth bass were captured from the seven sites without Topeka shiners in 2003 suggesting the absence of Topeka shiners may not be a direct result of largemouth bass presence and predation. Instead, relative abundance of largemouth bass, along with rosyface shiner and central stoneroller, may vary between sites with and without Topeka shiners for other reasons such as habitat differences or sedimentation tolerances. Topeka shiners and rosyface shiners both prefer low turbidity streams with clean sand, gravel, and bedrock substrates, and are intolerant to sedimentation (Cross and Collins Citation1995; Pflieger Citation1997). In contrast, central stoneroller and largemouth bass occur in a variety of habitat conditions and are more tolerant to turbidity and sedimentation (Cross and Collins Citation1995; Etnier and Starnes Citation1993; Pflieger Citation1997). The variation in relative abundance of these species between sites with and without Topeka shiners may be an indication of habitat differences among these sites.
We found that habitat varied among sites with and without Topeka shiners and that Topeka shiners absence was associated with increased proportional urban land use, increased proportional impoundment area, and greater mean stream width. Habitat changes are among the most common forms of stream degradation and the habitat of many streams we sampled have been altered by humans either directly (e.g. impoundments), indirectly (e.g. urban runoff), or both. The relationship between proportional impoundment area and stream morphology are similar to the results of Schrank et al. (Citation2001) but our study is the first, to our knowledge, to quantitatively link Topeka shiner distribution to urban land use.
Urban land use can impact stream ecosystems in many ways, including changes to many factors that have been linked with Topeka shiner distribution, such as water quality (Hatt et al. Citation2004; Walsh et al. Citation2005), stream hydrology (Hirsch et al. Citation1990; Roy et al. Citation2005), and channel morphology (MacRae and Rowney Citation1992; Paul and Meyer Citation2001). Because urban land use can alter many aspects of stream habitat and function, it may lead to extirpations of native species and widespread shifts in fish assemblage structure and function (Roy et al. Citation2005; Wang and Lyons Citation2003). Although the amount of urban land use is relatively low (<2%) in the Mill Creek watershed, it was a significant factor associated with the absence of Topeka shiners. These results were consistent with other studies that found that rare and sensitive species are more susceptible to changes in urban land use than other fishes and may decline or become extirpated with watershed proportional urban land use of 5% or less (Angermeier et al. Citation2004; Roy et al. Citation2005). As proportional urban land use approaches 10%, widespread declines in non-tolerant native species may occur (Angermeier et al. Citation2004; Marchetti et al. Citation2006), leading to lower species richness and diversity in watersheds where urban land is more prevalent (Roy et al. Citation2005; Wang and Lyons Citation2003). We found that fish community species richness and diversity were both higher at sites with Topeka shiners compared to the absent sites potentially indicating that urban land use is impacting other fishes in the Mill Creek watershed.
Sites without Topeka shiners had higher proportional impoundment area than sites with Topeka shiners. Increased proportional impoundment area may affect Topeka shiner populations by transforming habitats, increasing fragmentation, and altering stream hydrology (Winston et al. Citation1991; Nilsson et al. Citation2005). Additionally, increased impoundment area may compound the effect of urbanization and pose an additional pressure to native fishes by facilitating the establishment and abundance of largemouth bass and other non-native species (Boet et al. Citation1999; Poff et al. Citation2007). The relationship between increased proportional impoundment area and Topeka shiner absence is similar to the findings of studies examining Topeka shiners (Schrank et al. Citation2001) and other native Great Plains fishes in Illinois (Taylor et al. Citation2001), Oklahoma (Wilde and Ostrand Citation1999), and Texas (Winston et al. Citation1991). Conservation and management efforts targeting Topeka shiners may need to consider the distribution and abundance of impoundments and the possible limitations that may be associated with their presence.
Topeka shiner populations in Kansas have been reduced to about 10% of their historical area (Cross and Collins Citation1995; Schrank et al. Citation2001; Tabor Citation2002) but our results suggest that Topeka shiner population distribution may be stable in the Mill Creek watershed. Topeka shiner was the ninth most abundant species captured over the course of the study and Topeka shiner relative abundance was similar among all years of this study, suggesting that Topeka shiner relative abundance may not be decreasing in the Mill Creek watershed. Whereas our results indicate that Topeka shiner populations are relatively stable in the Mill Creek watershed, monitoring of populations outside the Mill Creek watershed may be needed to see if similar trends are occurring in other reaches. Topeka shiners in Kansas streams have declined greatly in the last 100 years (Cross and Collins Citation1995; Schrank et al. Citation2001; Tabor Citation2002) and continued monitoring of Topeka shiners throughout their range may help thwart future declines and help Topeka shiners repatriate areas where they were once common.
The results of this study are built on the study by Schrank et al. (Citation2001) to include data to detect long-term trends in Topeka shiner abundance. Our findings support the results of the previous short-term (one summer) study by Schrank et al. (Citation2001), but we found similar patterns evident over a 13-year temporal scale suggesting that the effects of land use and non-native fishes are prevalent over long time periods. Stream habitats and fish assemblages can vary substantially over time (Allen and Starr Citation1982; Poff Citation1997), and repeated sampling over longer temporal scales may lead to better information for management and conservation decisions. The consistent importance of small impoundments and largemouth bass presence indicates that anthropogenic actions may be one of the primary factors determining Topeka shiner distribution. Additionally, we found that sites with Topeka shiners had lower proportional area of urban land use in their watershed, further indicating that anthropogenic habitat changes may affect Topeka shiner abundance.
Although we found that metrics associated with human activity in and around streams (e.g. urban land and impoundments) are linked to the absence of Topeka shiners, conservation and rehabilitation efforts may help minimize the anthropogenic impact on Topeka shiners and other native fishes in these streams. For example, establishing riparian buffers around streams may reduce the impact of urbanization and agricultural practices on these streams (Wang et al. Citation2001; Herringshaw et al. Citation2010). By identifying factors associated with the presence and absence of Topeka shiner populations this study may help managers conserve current Topeka shiner populations and identify sites for future reclamation and conservation efforts. Further, our results indicate that anthropogenic actions alter Topeka shiner presence and the inclusion of such factors may be useful when developing conservation and management strategies designed to protect Topeka shiners and other native fishes from further declines.
Acknowledgements
This study was funded by the US Fish and Wildlife Service, Kansas Department of Wildlife, Parks, and Tourism, and the US Geological Survey. Fish and habitat samples were collected by members of the Kansas Department of Wildlife, Parks, and Tourism Stream and Monitoring Program. We are grateful to the private landowners for providing access to study sites located on private property. We are thankful for comments provided by Mike Quist and an anonymous reviewer, who provided valuable feedback and improved earlier versions of this work. The Kansas Cooperative Fish and Wildlife Research Unit is jointly sponsored by the Kansas Department of Wildlife, Parks, and Tourism, Kansas State University, the US Geological Survey, and the Wildlife Management Institute. The Missouri Cooperative Fish and Wildlife Research Unit is jointly sponsored by the Missouri Department of Conservation, the University of Missouri, the US Geological Survey, the US Fish and Wildlife Service, and the Wildlife Management Institute.
This work was authored as part of the Contributor's official duties as an Employee of the United States Government and is therefore a work of the United States Government. In accordance with 17 USC. 105, no copyright protection is available for such works under US Law.
References
- Allen , T FH and Starr , T B . 1982 . Hierarchy: perspectives for ecological complexity , 1st ed , Chicago , IL : University of Chicago Press .
- Angermeier , P L , Wheeler , A P and Rosenberger , A E . 2004 . A conceptual framework for assessing the impacts of roads on aquatic biota . Fisheries , 29 : 19 – 29 . doi: 10.1577/1548-8446(2004)29[19:ACFFAI]2.0.CO;2
- Boet , P , Belliard , J , Berrebi-dit-Thomas , R and Tales , E . 1999 . Multiple human impacts by the city of Paris on fish communities in the Seine River basin, France . Hydrobiologia , 410 : 59 – 68 . doi: 10.1023/A:1003747528595
- Bouska , W W and Paukert , C P . 2010 . Road crossing designs and their impact on fish assemblages of Great Plains Streams . American Fisheries Society , 139 : 214 – 222 . doi: 10.1577/T09-040.1
- Bramblett , R G , Johnson , T R , Zale , A V and Heggem , D G . 2005 . Development and evaluation of fish assemblage index of biotic integrity for northwestern Great Plains streams . American Fisheries Society , 134 : 624 – 640 . doi: 10.1577/T04-051.1
- Bunn , S E and Arthington , A H . 2002 . Basic principles and ecological consequences of altered flow regimes for aquatic biodiversity . Environmental Management , 30 : 492 – 507 . doi: 10.1007/s00267-002-2737-0
- Cross , F B and Collins , J T . 1995 . Fishes in Kansas , 2nd ed , Lawrence , KS : University of Kansas . University of Kansas Natural History Museum Public Education Series No. 14.
- Cross , F B and Moss , R E . 1987 . “ Historic changes in fish communities and aquatic habitats in plains streams of Kansas ” . In Community and evolutionary ecology of North American stream fishes , Edited by: Mathews , W J and Heids , D C . 155 – 165 . Norman , OK : University of Oklahoma Press .
- Cummins , K W . 1962 . An evaluation of some techniques for the collection and analysis of benthic samples and special emphasis on lotic waters . American Midland Naturalist , 67 : 477 – 504 . doi: 10.2307/2422722
- Dodds , W K , Gido , K B , Whiles , M R , Fritz , K M and Matthews , W J . 2004 . Life on the edge: the ecology of Great Plains prairie streams . Bioscience , 54 : 205 – 216 . doi: 10.1641/0006-3568(2004)054[0205:LOTETE]2.0.CO;2
- Dudgeon , D , Arthington , A H , Gessner , M O , Kawabata , Z , Knowler , D J , Leveque , C , Naiman , R J , Prieur-Richard , A , Soto , D Stiassny , M LJ . 2006 . Freshwater biodiversity: importance, threats, status and conservation challenges . Biology Reviews , 81 : 163 – 182 . doi: 10.1017/S1464793105006950
- Etnier , D A and Starnes , W E . 1993 . The fishes of Tennessee , Knoxville , TN : University of Tennessee Press .
- Fausch , K D and Bestgen , K R . 1997 . “ Ecology of fishes indigenous to the central and southwestern Great Plains ” . In Ecology and conservation of great plains vertebrates , Edited by: Knopf , F L and Samson , F B . 131 – 166 . New York , NY : Springer-Verlag .
- Geist , J. 2011 . Integrative freshwater ecology and biodiversity conservation . Ecological Indicators , 11 : 1507 – 1516 . doi: 10.1016/j.ecolind.2011.04.002
- Gido , K B , Schaefer , J F and Pigg , J . 2004 . Patterns of fish invasions in the Great Plains . Biological Conservation , 118 : 121 – 131 . doi: 10.1016/j.biocon.2003.07.015
- Hatt , B E , Fletcher , T D , Walsh , C J and Taylor , S L . 2004 . The influence of urban density and drainage infrastructure on the concentrations and loads of pollutants in small streams . Environmental Management , 34 : 112 – 124 . doi: 10.1007/s00267-004-0221-8
- Herringshaw , C J , Thompson , J R and Stewart , T W . 2010 . Learning about restoration of urban ecosystems: a case study integrating public perception, stormwater management, and ecological research . Urban Ecosystems , 13 : 535 – 562 . doi: 10.1007/s11252-010-0134-7
- Hirsch , R M , Walker , J F , Day , J C and Kallio , R . 1990 . “ The influence of man on hydrologic systems ” . In In surface hydrology , Edited by: Wolman , M G and Riggs , H C . 329 – 359 . Boulder , CO : Geological Society of America .
- Jackson , D A , Peres-Neto , P R and Olden , J D . 2001 . What controls who is where in freshwater fish communities – the roles of biotic, abiotic, and spatial factors . Canadian Journal of Fisheries and Aquatic Sciences , 58 : 157 – 170 .
- Jelks , H L , Walsh , S J , Burkhead , N M , Contreras-Balderas , S , Diaz-Pardo , E , Hendrickson , D A , Lyons , D A , Mandrak , N E , McCormick , F Nelson , J S . 2008 . Conservation status of imperiled North American freshwater and diadromous fishes . Fisheries , 33 : 372 – 386 . doi: 10.1577/1548-8446-33.8.372
- Kerns , H A and Bonneau , J L . 2002 . Aspects of the life history and feeding habits of the Topeka shiner (Notropis Topeka) . Transactions of the Kansas Academy of Science , 105 : 125 – 142 . doi: 10.1660/0022-8443(2002)105[0125:AOTLHA]2.0.CO;2
- Krebs , C J . 1989 . Ecological methodology , New York , NY : Harper and Row Publishers .
- Knight , G L and Gido , K B . 2005 . Habitat use and susceptibility to predation of four prairie stream fishes: implications for conservation of the endangered Topeka shiner . Copeia , 2005 : 38 – 47 . doi: 10.1643/CE-04-226R1
- Kwak , T J and Peterson , J T . 2007 . “ Community indices, parameters, and comparisons ” . In Analysis and interpretation of freshwater fisheries data , Edited by: Guy , C S and Brown , M L . 677 – 763 . Bethesda , MD : American Fisheries Society .
- Ludwig , J A and Reynolds , J F . 1989 . Statistical ecology , New York , NY : John Wiley & Sons .
- MacRae , C R and Rowney , A C . 1992 . “ The role of moderate flow events and bank structure in the determination of channel response to urbanization ” . In Resolving conflicts and uncertainty in water management , Edited by: Shrubsole , D . 12.1 – 12.21 . Kingston , Ontario : Canadian Water Resources Association .
- Mammoliti , C S . 2002 . The effects of small watershed impoundments on native stream fishes: a focus on the Topeka Shiner and Hornyhead Chub . Transactions of the Kansas Academy of Science , 105 : 219 – 231 . doi: 10.1660/0022-8443(2002)105[0219:TEOSWI]2.0.CO;2
- Marchetti , M P , Lockwood , J L and Light , T . 2006 . Effects of urbanization on California's fish diversity: differentiation, homogenization, and the influence of spatial scale . Biological Conservation , 127 : 310 – 318 . doi: 10.1016/j.biocon.2005.04.025
- Minkley , W L and Cross , F B . 1959 . Distribution, habitat, and abundance of the Topeka shiner Notropis topeka (Gilbert) in Kansas . American Midland Naturalist , 61 : 210 – 217 . doi: 10.2307/2422352
- Nilsson , C , Reidy , C A , Dynesius , M and Revenga , C . 2005 . Fragmentation and flow regulation of the world's large river systems . Science , 308 : 405 – 408 . doi: 10.1126/science.1107887
- Paul , M J and Meyer , J L . 2001 . Streams in an urban landscape . Annual Review of Ecology and Systematics , 32 : 333 – 365 . doi: 10.1146/annurev.ecolsys.32.081501.114040
- Perkin , J S and Gido , K B . 2011 . Stream fragmentation thresholds for a reproductive guild of Great Plains fishes . Fisheries , 36 : 371 – 383 . doi: 10.1080/03632415.2011.597666
- Pflieger , W L . 1997 . The fishes of Missouri , Jefferson City , MO : Missouri Department of Conservation .
- Poff , N L . 1997 . Landscape filters and species traits: towards mechanistic understanding and prediction in stream ecology . Journal of the Acoustical Society of America , 16 : 391 – 409 .
- Poff , N L , Olden , J D , Merritt , D M and Pepin , D M . 2007 . Homogenization of regional river dynamics by dams and global biodiversity implications . Proceedings of the National Academy of Sciences of the United States , 104 : 5732 – 5737 . doi: 10.1073/pnas.0609812104
- Rahel , F J . 2000 . Homogenization of fish faunas across the United States . Science , 288 : 854 – 856 . doi: 10.1126/science.288.5467.854
- Rahel , F J . 2007 . Biogeographic barriers, connectivity and homogenization of freshwater faunas: it's a small world after all . Freshwater Biology , 52 : 696 – 710 . doi: 10.1111/j.1365-2427.2006.01708.x
- Roy , A H , Freeman , M C , Freeman , B J , Wenger , S J , Ensign , W E and Meyer , J L . 2005 . Investigating hydrologic alteration as a mechanism of fish assemblage shifts in urbanizing streams . Journal of the Acoustical Society of America , 24 : 656 – 678 .
- Samson , F B , Knopf , F L and Ostlie , W R . 2004 . Great Plains ecosystems: past, present, and future . Wildlife Society B , 32 : 6 – 15 . doi: 10.2193/0091-7648(2004)32[6:GPEPPA]2.0.CO;2
- Schrank , S J , Guy , C S , Whiles , M R and Brock , B L . 2001 . Influence of instream and landscape-level factors on the distribution of Topeka shiners (Notropis topeka) in Kansas streams . Copeia , 2001 : 413 – 421 . doi: 10.1643/0045-8511(2001)001[0413:IOIALL]2.0.CO;2
- Strayer , D L and Dudgeon , D . 2010 . Freshwater biodiversity conservation: recent progress and future challenges . Journal of the Acoustical Society of America , 29 : 344 – 358 .
- Tabor , V M . 2002 . Endangered and threatened wildlife and plants; designation of critical habitat for the Topeka Shiner. . Fort Snelling (MN): Federal Register , 67 : 54262 – 54292 .
- Taylor , C A , Knouft , J H and Hiland , T M . 2001 . Consequences of stream impoundment on fish communities in a small North American drainage . Regulated Rivers , 17 : 687 – 698 .
- Wall , S S and Berry , Jr. CR . 2004 . Road culverts across streams with the endangered Topeka shiner, (Notropis topeka), in the James, Vermillion, and Big Sioux River basins . Proceedings of the South Dakota Academy of Science , 83 : 125 – 135 .
- Walsh , C J , Roy , A H , Feminella , J W , Cottingham , P D , Groffman , P M and Morgan , R P . 2005 . The urban stream syndrome: current knowledge and the search for a cure . Journal of the Acoustical Society of America , 24 : 706 – 723 .
- Wang , L , Lyons , J , Kanehl , P and Bannerman , R . 2001 . Impacts of urbanization on stream habitat and fish across multiple spatial scales . Environmental Management , 28 : 255 – 266 .
- Wang , L and Lyons , J . 2003 . “ Fish and benthic macroinvertebrate assemblages as indicators of stream degradation in urbanizing watersheds ” . In Biological response signatures. Indicator patterns using aquatic communities , Edited by: Simon , T P . 227 – 249 . Boca Raton , FL : CRC Press .
- Warren , M L Jr. , Burr , B M , Walsh , S J , Bart , Jr. HL , Cashner , R C , Etnier , D A , Freeman , B J , Kuhajda , B R , Mayden , R L Robison , H W . 2000 . Diversity, distribution, and conservation status of the native freshwater fishes of the southern United States . Fisheries , 25 : 7 – 31 .
- Wilde , G R and Ostrand , K G . 1999 . Changes in the fish assemblage of an intermittent stream upstream from a Texas impoundment . Texas Journal of Science , 51 : 203 – 210 .
- Winston , M R . 2002 . Spatial and temporal species associations with the Topeka Shiner (Notropis topeka) in Missouri . Journal of Freshwater Ecology , 17 : 249 – 261 .
- Winston , M R , Taylor , C M and Pigg , J . 1991 . Upstream extirpation of four minnow species due to damming of a prairie stream . American Fisheries Society , 120 : 98 – 105 .
- Xenopoulos , M A , Lodge , D M , Alcamo , J , Marker , M , Schulze , K and VanVuurens , D P . 2005 . Scenarios of freshwater fish extinctions from climate change and water withdrawal . Global Change Biology , 11 : 1157 – 1164 .
- Zimmerman , J L . 1993 . The birds of Konza: the avian ecology of the tallgrass prairie , Lawrence , KS : University Press of Kansas .