Abstract
Prey fishes use chemical cues in their predator's diet to cue the context and timing of antipredator behaviors that reduce the risk of predation. They also use dietary cues to learn to recognize and later detect the presence of a predator. Because dietary chemical information decreases hunting success of predators there should be selection on predators to mask or obliterate these cues through biochemical processes in their digestive tract. In this study, we compared response intensity of predator-naïve fathead minnows to the signature odors of two novel predators that had been fed either a minnow diet or a non-minnow diet. Unprocessed minnow mash and dechlorinated blank water were used as positive and negative controls, respectively. One predator was from a relatively ancestral fish group, the order esociformes, while the other predator was from a relatively derived group, the order perciformes. Naïve minnows were able to detect the presence of minnows in the diet of the ancestral and derived predators with equal efficiency and behavioral responses to minnow dietary cues were significantly different from non-minnow dietary treatments. We found no evidence that fish of relatively derived phylogeny have evolved counter-labeling strategies through enhanced biochemical processes during digestion.
Introduction
Chemical cues comprise a major source of information about the temporal and spatial distribution of predation risk in aquatic habitats (Ferrari et al. Citation2010). Different classes of cues include those released before attack (predator odor, cues released by disturbed prey), those released after injury (alarm cues) and after ingestion (dietary cues) (Wisenden 2000). Many aquatic taxa have been shown to detect the presence of conspecifics in the diet of novel predators (Mathis and Smith Citation1993a, Citation1993b; see Chivers and Mirza Citation2001; Ferrari et al. Citation2010 for reviews). For example, pike-naïve minnows increase shelter use and reduce feeding activity when exposed to odor of northern pike (Esox lucius) that had been fed minnows but not to the odor of pike that had been fed breeding male fathead minnows (skin components change during spawning) (Mathis and Smith Citation1993a). In live predation trials, juvenile brook charr (Salvelinus fontinalis) have a significantly higher probability of surviving an encounter with a pike if they are first exposed to odor of pike on a diet of brook charr or rainbow trout, but not if first exposed to odor of pike on a diet of brook stickleback (Culaea inconstans) (Mirza and Chivers Citation2003a). Because prey exploit the presence of dietary alarm cues to reduce their risk of predation (i.e. hunting success of the predator), there should be concomitant selection on predators to counteract these effects. For example, one behavioral example of predator response to chemical labeling by prey may be localized defecation away from the site of foraging (Brown et al. Citation1996).
Post-ingestion cues are intriguing because it is not known if cue chemistry remains intact after passage through the predator's gut or if prey fishes have separate receptors to detect predictable metabolites of conspecific chemical alarm cues. The degree to which dietary cues reduce hunting success should put selection on predators to mask or degrade chemical cues of their prey to reduce detectability. In only one documented case did prey fail to detect the presence of dietary cues of conspecifics (Feminella and Hawkins Citation1994). In this instance, tailed frog tadpoles (Ascaphus truei) responded with anti-predator behavior to tadpole cues in the diet of several predators such as giant salamanders (Dicamptodon spp.), cut-throat trout (Salmo clarki) and brook trout (Salvelinus fontinalis) but not to conspecific dietary cues from shorthead sculpins (Cottus confusus). This study suggested that sculpins may have evolved a way to biochemically breakdown alarm cues into unrecognizable metabolites (Ferrari et al. Citation2008; Wisenden et al. Citation2009) or to chemically mask the presence of these cues (Chivers and Smith Citation1998).
More generally, this study raises the question of whether phylogenetically derived fish species, such as sculpin, are more effective than relatively ancestral fish, such as northern pike, at biochemically degrading alarm cues (Mathis and Smith Citation1993a). In the evolutionary arms race between predators and their prey, prey are selected to become ever better at detecting the presence of predators (e.g. via dietary alarm cues they inadvertently release), while predators should be selected to become ever better at concealing their presence by biochemically degrading or masking dietary alarm cues to render themselves chemically invisible to naïve prey. Moreover, prey learn to recognize predators by the signature odor of the predator itself, independent of diet, by associating predator odor with chemical alarm cues of conspecifics (Mathis and Smith Citation1993b; Chivers and Mirza Citation2001; Ferrari et al. Citation2010). It is not known how much of acquired predator recognition in natural populations occurs through dietary cues versus freshly released alarm cues released during prey capture. If learned recognition occurs primarily via dietary cues, which is quite plausible, the selection gradient promoting biochemical masking of dietary cues may be very steep indeed.
In this study, we compared response intensity of fathead minnows to dietary cues from two different novel predators; the relatively ancestral central mudminnow (Protacanthoptyrgii, Esociformes, Umbridae) and the relatively derived bluegill sunfish (Acanthopterygii, Perciformes, Centrarchidae). Umbrid fossils date back to the Oligocene, ca. 34–23 million years ago (Nelson Citation1994). A notable characteristic of the esociformes is that they lack the pyloric ceca that occur on the anterior region of the intestine of derived teleosts that serve to retain food for more complete digestion. Fossil centrarchid fishes date back only as far as the late Miocene (23–5.3 million years ago) and possess derived traits such as spiny fins (defense), improved locomotion through physoclistic inflation of the gas bladder for buoyancy control, and anterior migration of the pelvic girdle and dorsal migration of the pectoral girdle for greater maneuverability (Nelson Citation1994). We tested if centrarchids show improved efficiency in biochemical breakdown of ingested prey. If selection on foraging efficiency between these two phylogenies included selection for digestive efficiency, then we predict that predator-naïve minnows should respond more intensively to dietary cues produced by mudminnows than to dietary cues produced by sunfish.
Methods
Study organisms
Lab-reared (predator-naïve) fathead minnows were acquired from Wilson Environmental Labs (Duluth, MN) at 30 days of age, housed in 38-L aquaria and maintained on a diet of commercial flake food. Fish were maintained in the lab for at least four weeks before testing began. Our representative perciform was the bluegill sunfish (Lepomis macrochirus). Sunfish were collected from the wild by seine net and held in 190-L lab aquaria. Our representative esociform was the central mudminnow (Umbra limi), which were purchased through an online fish exchange organization (AquaBid) and maintained in 38-L aquaria in the lab. Mudminnows and bluegill sunfish were chosen as test species because they are relatively small in body size and adapt well to lab aquaria relative to other members of their respective clades. In nature, mudminnows consume a diet comprising 70–90% small fish, especially during the winter (Chilton et al. Citation1984; Martin-Bergmann and Gee Citation1985). Bluegill sunfish are mainly planktivorous in nature (Keast Citation1978; Olson et al. Citation2003) although they occasionally eat minnows (Sarker Citation1977) and readily do so in captivity.
Stimulus preparation
We prepared and tested six different test odorants. We prepared a positive control using chemical alarm cues of fathead minnows. To prepare this cue, we humanely killed minnows and placed the whole body into a beaker with 10 ml dechlorinated water and homogenized it with a hand blender. This mixture was filtered through a loose wad of polyester wool, diluted to a final volume of 100 ml. Each dose contained the amount of alarm cue equivalent to one fish (total length ca. 2 cm). We prepared a negative control of blank water by aliquoting dechlorinated water in 100-ml doses and freezing them at −20 °C until needed. Individual sunfish (total length 13.0 ± 1.2 cm, n = 4) and mudminnows (4.8 ± 0.13 cm, n = 10) were maintained on a diet of either fathead minnows or Lumbriculus sp. (an aquatic oligochaete annelid) purchased from Wilson Environmental Labs, Duluth, MN. Fish were fed Lumbriculus diet ad libitum for 2 days, then placed in individual 38-L tanks filled with 19 L for mudminnows or 28 L for sunfish and left for 24 h. Stimulus collection tanks were aerated but not filtered. After 24 h, the fish were moved back to the holding tanks. Water from the stimulus collection tanks was combined together then aliquotted into 100-ml doses and frozen at −20 °C until needed. After 3 days of fasting, the same fish were started on a minnow diet, ad libitum, for 2 days, then placed in separate stimulus collection tanks as described above. After 24 h, this water was collected, combined together and then aliquotted into 100-ml doses and frozen at −20 °C until needed.
Behavioral assay protocol
Pairs of fathead minnows were placed in 38-L aquaria filled with fresh dechlorinated water and a thin layer of naturally colored gravel and filtered by an air-powered sponge filter. Fish were given at least 24 h to adjust to the new tank before collection of behavioral data. A second air line tube wedged into the rigid plastic vent tube of the sponge filter allowed for surreptitious introduction of test odorants into the turbulent stream of air and water exiting the filter, which masked minor pressure changes associated with cue injection and also dispersed the test odorant in the test aquarium. A 5 × 5 cm grid was drawn on the outside of the front pane of each test aquarium to monitor the activity and vertical distribution of test fish. Behavioral responses to predation risk (via alarm cues or dietary cues) by fathead minnows are a reduction in activity and movement to the bottom (Lawrence and Smith Citation1989). Each trial began with a 5-min pre-stimulus observation period in which activity by both fish was recorded as the total number of grid lines crossed over the observation period. Vertical distribution was recorded as the horizontal row of the grid each fish occupied at 15-s intervals over the 5-min observation period. The vertical distribution was recorded as the sum of these numbers. Immediately following the pre-stimulus period, one of the six test odorants was introduced via the injection hose wedged into the outlet of the sponge filter. Stimulus injection was by syringe attached to the injection hose. Sixty ml of previously retained tank water was used to flush the test cue from the injection line into the test tank. A 5-min post-stimulus observation period followed immediately after stimulus injection in which activity and vertical distribution was recorded as before. We conducted 16 trials for each test odorant (n = 96 trials). Each pair of fish was tested only once. Injection lines were replaced and we drained and refilled test aquaria after each trial.
Data analysis
Behavioral change in response to introduction of test cue (post-stimulus minus pre-stimulus) was found to be normally distributed for change in activity (Kolmogorov-Smirnov test, Z = 1.068, p = 0.205) and change in vertical distribution (Kolmogorov-Smirnov test, Z = 0.672, p = 0.758). We subsequently analyzed the data with a 3 × 2 factorial analysis of variance(ANOVA) using cue source (mudminnow, sunfish, control) and minnow cue (present or absent) as categorical predictors.
Results
There was a significant effect (p < 0.05) of the presence of minnow skin (as raw skin extract [positive control] or in the diet of the two phylogenetically disparate predator species) compared to the absence of minnow chemical cue (as blank water [negative control] or predator odor on Lumbriculus diet). When alarm cues were present (as raw alarm cues or as digested dietary cues) minnows reduced activity (Figure ) and increased time at the bottom (Figure , Table ). There was no effect of the cue source (control versus mudminnow versus sunfish) and there was no significant interaction between alarm cue source and ability of fathead minnows to detect it (p > 0.05, Table ).
Table 1. Statistical results from 3 × 2 factorial analysis of variance on behavioral responses (change in activity, change in vertical distribution) to test cues from three different sources (sunfish, mudminnow or controls), whether or not minnow alarm cue was present (alarm cue control from minnow mash, or sunfish or mudminnow fed a diet of fathead minnows) or absent (water control or sunfish or mudminnow fed a diet of Lumbriculus worms), and the statistical interaction between these two predictors.
Figure 1. Mean (±1 SE) change (post-minus pre-stimulus) in activity of fathead minnows after cue injection of test cues that contained minnow chemical alarm cues (gray fill: raw skin extract control, odor of mudminnows and sunfish fed a diet of fathead minnows) or did not contain minnow chemical alarm cues (open fill; blank water control, odor of mudminnows or sunfish fed a diet of Lumbriculus).
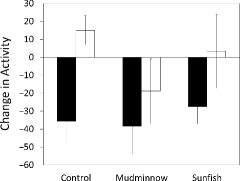
Figure 2. Mean (± SE) change (post-minus pre-stimulus) in vertical distribution of fathead minnows after cue injection for test cues that contained minnow chemical alarm cues (gray fill: raw skin extract control, odor of mudminnows and sunfish fed a diet of fathead minnows) or did not contain minnow chemical alarm cues (open fill; blank water control, odor of mudminnows or sunfish fed a diet of Lumbriculus).
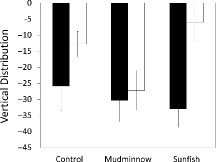
Discussion
Fathead minnows were able to detect and respond to the presence of chemical cues from damaged conspecifics in all sources presented. The effect of a minnow diet was the same for both predator species tested, and neither predator species cues produced response intensities that differed from that of the undigested alarm cue control treatment. Our data do not provide any support for the hypothesis that phylogenetically derived fish, such as a perciform sunfish, are any better at evading chemical labeling by fathead minnows than a relatively ancestral fish, in this case an esociform mudminnow. Perciform (sunfish, bass, walleye, perch, crappies) and esociform (pike, muskellunge, pickerel, mudminnows) phylogenies are both dominated by piscivorous predators. This finding leads to several possible conclusions. The first possibility is that chemical labeling of predators with dietary cues does not exert sufficient selection pressure on predators to result in biochemical innovations in the digestive system to keep this information from prey. The numerous studies clearly demonstrating the importance of dietary cues for guiding antipredator behavioral responses (e.g. Mirza and Chivers Citation2001, Citation2003a, Citation2003b; Chivers et al. Citation2002, reviewed by Chivers and Mirza et al. Citation2001) are strong evidence that dietary cues are indeed important to prey. If prey have evolved responses to this information, then shielding this information from prey should be important to predators.
A second possibility is that the chemical nature of minnow skin is too stable to degrade. A third possibility is that alarm cue is too diverse (comprising multiple redundant components in a signature mixture [Wyatt Citation2010]); the mixture remains recognizable even when one component of the mixture is biochemically degraded). A multicomponent alarm cue therefore would be resilient against biochemical countermeasures in a predator's digestive system. Assessing this hypothesis is hampered by the limited knowledge of the chemistry of this system. The best work to date is that of Mathuru et al. (Citation2012), who showed that glycosaminoglycan (GAG) chondroitin is both present in the skin of members of the minnow family and elicits a behavioral fright reaction similar to that of skin extract and, moreover, stimulates the same regions of the olfactory system as does skin extract. GAG chondroitin is biochemically accessible to a variety of enzymatic pathways that limits the duration of biological activity. At 18 °C, minnow skin extract no longer elicits a behavioral response in the lab or field after 3 h (Wisenden et al. Citation2009). The dietary cues used in this study were at least 24 h old and had been exposed to biochemical digestive processes and yet still evoked a behavioral response. This suggests that minnows may respond to metabolites of skin extract in a way that is not time sensitive or that biochemical by-products of skin extract degradation remain biologically active when accompanied by signature odor of another fish species.
A fourth possibility is that prey species, such as fathead minnows, evolve antipredator responses at a pace faster than the rate at which predators can evolve biochemical strategies for chemical stealth. The benefit of evading predation is much greater for prey (life) than the benefit of stealthy hunting (lunch) causing prey to adapt more quickly to changes in predator digestive chemistry than the rate at which predators can adapt to improved detection abilities of their prey.
Acknowledgements
Funding for this work was provided by a student research grant to RS and a faculty research grant to BDW, both from the College of Social and Natural Sciences, Minnesota State University Moorhead, MN. All procedures used for the housing of test subjects and collection of data were approved by the Minnesota State University Moorhead Institutional Animal Care and Use Committee under protocol 10-T-Biol-009-N-N-C/D.
References
- Brown GE, Chivers DP, Smith RJF. 1996. The effects of diet on localized defecation by northern pike (Esox lucius). Journal of Chemical Ecology. 22:467–475.
- Chilton G, Martin KA, Gee JH. 1984. Winter feeding: an adaptive strategy broadening the niche of the central mudminnow Umbra limi. Environmental Biology Fishes. 10:215–219.
- Chivers DP, Mirza RS. 2001. Predator diet cues and the assessment of predation risk by aquatic vertebrates: a review and prospectus. In: Marchlewska-Koj A, Lepri JJ, Müller-Schwarze D, editors. Chemical Signals in Vertebrates. Vol. 9: New York (NY): Plenum Press. p. 277–284.
- Chivers DP, Mirza RS, Johnston J. 2002. Learned recognition of heterospecific alarm cues enhances survival during encounters with predators. Behaviour. 139:929–938.
- Chivers DP, Smith RJF. 1998. Chemical alarm signalling in aquatic predator-prey systems: a review and prospectus. Écoscience. 5:338–352.
- Feminella JW, Hawkins CP. 1994. Tailed frog tadpoles differentially alter their feeding behavior in response to non-visual cues from four predators. Journal of the North American Benthological Society. 13:310–320.
- Ferrari MCO, Messier F, Chivers DP. 2008. Degradation of alarm cues under natural conditions: risk assessment by larval amphibians. Chemoecology. 17:263–266.
- Ferrari MCO, Wisenden BD, Chivers DP. 2010. Chemical ecology of predator-prey interactions in aquatic ecosystems: a review and prospectus. Canadian Journal of Zoology. 88:698–724.
- Keast A. 1978. Feeding interactions between age-groups of pumpkinseeds (Lepomis gibbosus) and comparisons with bluegill (L. macrochirus). Journal of the Fisheries Research Board of Canada. 35:12–27.
- Lawrence BJ, Smith RJF. 1989. Behavioural response of solitary fathead minnows, Pimephales promelas, to alarm substance. Journal of Chemical Ecology. 15:209–219.
- Martin-Bergmann KA, Gee JH. 1985. The central mudminnow, Umbra limi (Kirtland), a habitat specialist and resource generalist. Canadian Journal of Zoology. 63:1753–1764.
- Mathis A, Smith RJF. 1993a. Chemical labelling of northern pike (Esox lucius) by the alarm pheromone of fathead minnows (Pimephales promelas). Journal of Chemical Ecology. 19:1967–1979.
- Mathis A, Smith RJF. 1993b. Fathead minnows, Pimephales promelas, learn to recognize northern pike, Esox lucius, as predators on the basis of chemical stimuli from minnows in the pike's diet. Animal Behaviour. 46:645–656.
- Mathuru AS, Kibat C, Cheong WF, Shui G, Wenk MR, Friedrich RW, Jesuthasan S. 2012. Chondroitin fragments are odorants that trigger fear behavior in fish. Current Biology. 22: 1–7. 6:45 11th
- Mirza RS, Chivers DP. 2001. Learned recognition of heterospecific alarm signals: the importance of a mixed predator diet. Ethology. 107:1007–1018.
- Mirza RS, Chivers DP. 2003a. Fathead minnows learn to recognize heterospecific alarm cues they detect in the diet of a known predator. Behaviour. 140:1359–1370.
- Mirza RS, Chivers DP. 2003b. Predator diet cues and the assessment of predation risk by juvenile brook charr: do diet cues enhance survival? Canadian Journal of Zoology. 81:126–132.
- Nelson JS. 1994. Fishes of the world, 3rd ed. New York (NY): Wiley.
- Olson NW, Paukert CP, Willis DW, Klammer JA. 2003. Prey selection and diets of bluegill Lepomis macrochirus with differing population characteristics in two Nebraska natural lakes. Fisheries Management and Ecology. 10:31–40.
- Sarker AL. 1977. Feeding ecology of the bluegill, Lepomis macrochirus, in two heated reservoirs of Texas. III. Time of day and patterns of feeding. Transactions of the American Fisheries Society. 106:596–601.
- Wisenden BD. 2000. Olfactory assessment of predation risk. Philosophical Transactions of the Royal Society. 355:1205–1208.
- Wisenden BD, Rugg ML, Korpi NL, Fuselier LC. 2009. Estimates of active time of chemical alarm cues in a cyprinid fish and an amphipod crustacean. Behaviour. 146:1423–1442.
- Wyatt TD. 2010. Pheromones and signature mixtures: defining species-wide signals and variable cues for identity in both invertebrates and vertebrates. Journal of Comparative Physiology. A196:685–700.