Abstract
Increasing concerns over climate change have renewed interest in temperature effects on aquatic organisms. The effects of temperature, food concentration, population density, and photoperiod on Daphnia carinata fitness were examined using life-table experiments in a full factorial design. The high temperature enhanced fitness at the high food concentration, but depressed it at the low food concentration. The temperature–food interactions were significant in the four combinations of two population densities and two photoperiods. The temperature–population density interactions were significant in the two combinations of two photoperiods and the high food concentration, in which the high population density masked the temperature effects. The temperature–photoperiod interaction was only significant in one combination with the low population density and the low food concentration, in which the long day length weakened the temperature effects. Such complicated interactions suggest that multiple ecological variables need to be taken into account simultaneously when assessing temperature effects on zooplankton in nature.
Introduction
Distribution, abundance, production, and evolution of zooplankton are regulated by many ecological variables, of which temperature is the most studied and likely the most important (Burns Citation1969; Giebelhausen and Lampert Citation2001; Pajk et al. Citation2012). Increasing temperature usually enhances zooplankton feeding, growth, and reproduction (Burns Citation1969; Korpelainen Citation1986; Achenbach and Lampert Citation1997; Kee and Ebert Citation1996). Increasing temperature also exacerbates the inhibitory effects of toxic cyanobacteria on population growth rates (Claska and Gilbert Citation1998) and decreases salinity tolerance (Hall and Burns Citation2002) in daphniids. Recently, the possibility of a 4 °C increase in average temperatures accompanied by occasional brief extreme events by the end of the twenty-first century due to climate change has reignited interest in temperature effects (Thuiller Citation2007). Global warming may affect zooplankton population dynamics both directly through influencing individual physiology, and indirectly through altering the properties of their habitats, consequently shaping both predator–prey interactions and the control on algal biomass (Wojtal-Frankiewicz Citation2012).
Since zooplankton in nature are exposed to several variables simultaneously and in various combinations, their growth and reproduction depend not only on temperature, but also on other ecological variables, such as food quantity and quality, population density, and photoperiod (Burns Citation2000; Lürling et al. Citation2003; Alekseev and Lampert Citation2004). Phytoplankton biomass within a temperate lake often spans a thousandfold range across temporal and spatial scales (Carpenter and Kitchell Citation1987). Typical reaction norms to food concentration follow a rectangular hyperbolic numerical response (Lampert Citation1978). In addition, considerable temporal and spatial heterogeneities of population density are common for many zooplankton species. For example, filter-feeding daphniids often form dense swarms with typical densities of 103 ind.·L−1 (Kvam and Kleiven Citation1995). Although zooplankton swarms could serve as a refuge to avoid predation, high population density may have deleterious consequences on zooplankton directly by altering behavioral and physiological responses or indirectly by reducing food concentration (Jakobsen and Johnsen Citation1988). Feeding activities of Daphnia are suppressed by infochemicals released by congeners and conspecifics (Lürling et al. Citation2003). Reduced feeding would subsequently result in depressed growth and reproduction in zooplankton (Burns Citation2000). Finally, although light conditions are less often as important as temperature and food in determining zooplankton developmental rates, photoperiod could serve as a cue for seasonal changes and subsequently regulate seasonal life history in zooplankton. Photoperiod can directly or indirectly alter zooplankton feeding, locomotory patterns, molt cycle, and reproduction (Starkweather Citation1976, Citation1983). In combination with food concentration, photoperiod can influence multiple parameters of Daphnia magna life history (Alekseev and Lampert Citation2004).
Predicting future responses of zooplankton to global warming requires an understanding of the interactive effects of temperature and other ecological variables. Only a few studies have considered the combined effects of ecological variables on zooplankton. Significant interactions between increased temperature and food concentration have been observed in several Daphnia species as the temperature effects are more pronounced at high food concentrations (Orcutt and Porter Citation1984; Giebelhausen and Lampert Citation2001). A combination of high temperature and low food level even has a negative influence on growth rate and production in the small freshwater ciliate Urotricha farcta (Weisse et al. Citation2002). In addition to food quantity, interactions between temperature and food quality have recently come into focus. For example, the growth of D. magna did not respond to dietary phosphorus limitation when the temperature was the lowest, but was more depressed with increasing temperature (Persson et al. Citation2011). Pajk et al. Citation(2012) reported that the interactions between temperature and food quality on the fitness parameters of D. magna and Daphnia pulex were influenced by maternal conditions. Compared with some studies looking at the combined effects of temperature and food, there are far fewer studies on the possible interactive effects of temperature and other variables, such as population density and photoperiod. A recent study showed that long day length masked the effect of high temperature on the maximum length and the age at first reproduction of D. magna (Martínez-Jerónimo Citation2012).
The aims of this study are to test (1) to what extent temperature, food concentration, population density, and photoperiod interact to determine zooplankton fitness; (2) whether the interactions between temperature and one variable would be affected by another variable; and (3) whether these interactions should be taken into account when predicting zooplankton responses to global warming in natural environments. In an effort to separate the effects of four variables and their interactions on zooplankton fitness, we investigated D. carinata fitness using a full factorial design of temperature, food concentration, population density, and photoperiod. D. carinata is a dominant herbivore in ponds, reservoirs, and lakes of subtropical regions (Hall and Burns Citation2002; Deng et al. Citation2008; Jiang et al. Citation2013a) and is used extensively as a model zooplankton species in toxicological, ecological, and evolutionary studies (Grant and Bayly Citation1981; Cáceres et al. Citation2007; Jiang et al. Citation2013b). The results may contribute to our knowledge of zooplankton responses under global warming scenarios.
Methods
The green algae Scenedesmus obliquus (FACHB-416) was obtained from the Freshwater Algae Culture Collection of the Institute of Hydrobiology, the Chinese Academy of Sciences, People's Republic of China. The algal culture was maintained at 20 °C with a 12:12 light:dark cycle and a light intensity of 50 μmol photons m−2·s−1 and diluted biweekly with autoclaved BG-11 medium. A clone of D. carinata was isolated from Shangyihu Pond in Shanghai, China. Stock cultures of D. carinata were raised in 20-L containers at about 20 °C under dim light. Animals were fed 500 μg·C·L−1 of S. obliquus daily and half the medium was renewed weekly with fresh, filtered tap water with a constant pH (7.8–8.0). Algal suspensions were prepared by measuring the light absorption of a stock culture in a spectrophotometer at 676 nm followed by appropriate dilution with 0.45-μm filtered water using a pre-established linear regression for light absorption versus carbon content.
A full factorial design was used to investigate the interactions between temperature (15 °C and 25 °C), food concentration (100 μg·C·L−1 and 1000 μg·C·L−1), population density (60 ind.·L−1 and 300 ind.·L−1), and photoperiod (8:16 and 16:8 light:dark) with four replicates per treatment. Fifty neonates were collected from stock cultures and were transferred into two 1-L beakers. They were fed 500 μg·C·L−1 of S. obliquus and acclimated to 15 °C and 25 °C, respectively, by changing the incubation temperature at a rate of 1 °C·d−1. Life-table experiments were conducted using flow-through systems driven by peristaltic pumps at a rate of 3 L algal suspension per day. Thirty synchronized neonates from the third brood within 24 h of birth were transferred to 500 mL or 100 mL algal suspensions, which formed the population densities of 60 ind.·L−1 or 300 ind.·L−1, respectively. This procedure assured that all experimental animals were raised at the appropriate temperature for one generation to minimize maternal effects. The number of survivors and newborn neonates was recorded daily.
The intrinsic rate of population increase, r, was calculated iteratively by the Euler–Lotka equation:
where lx is the proportion of survivors at day x and mx is the number of offspring produced at day x. The intrinsic rate of population increase of daphniids is a reliable proxy for their fitness when they live in conditions with low predation pressure (Lampert and Trubetskova Citation1996). A four-way analysis of variance (ANOVA) was conducted to test the effects of the main factors (temperature, food concentration, population density, and photoperiod) and their interactions on D. carinata fitness. Additional two-way ANOVAs were performed separately to analyze the effects of food concentration, population density, and photoperiod on the temperature reaction norms of D. carinata fitness. Effect sizes of main effects, interactions, and error were estimated by η2, which is defined as the proportion of variance in an ANOVA test. Statistical analyses were performed in SPSS 16.0 statistical package.
Results
All four main variables (temperature, food concentration, population density, and photoperiod) significantly affected D. carinata fitness (four-way ANOVA, p < 0.01 for all). Many interactive effects among these variables were significant (). Higher fitness values were observed in D. carinata grown in the high temperature (25 °C), high food concentration (1000 μg·C·L−1), and low population density (60 ind.·L−1) treatments. Negative values were observed for the individuals maintained at the low food concentration (100 μg·C·L−1), high population density (300 ind.·L−1), and short day-length (8 h) treatments ().
Table1. Summary of the four-way ANOVA results on the effects of temperature, food concentration, population density, and photoperiod on D. carinata fitness. For clarity, only the F values and their significance (*indicates p < 0.05; ** indicates p < 0.01; *** indicates p < 0.001) are shown.
Figure1. Effects of food concentration on temperature reaction norms of D. carinata fitness in the four combinations of two photoperiods and two population densities.
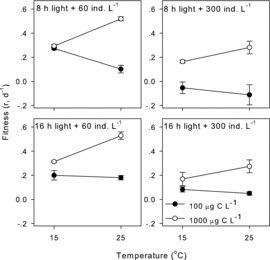
Higher temperature enhanced D. carinata fitness at the food concentration of 1000 μg·C·L−1 but depressed it at the food concentration of 100 μg·C·L−1 (). Higher food concentration significantly increased D. carinata fitness in all four combinations of two population densities and two photoperiods (two-way ANOVA, p < 0.001 for all, ). The temperature–food interactions were significant in those combinations (two-way ANOVA, p < 0.05 for all). The slope of temperature reaction norms switched from positive to negative with a reduction in food concentration from 1000 μg·C·L−1 to 100 μg·C·L−1 (). The η2 indicated that the interactive effect was most pronounced under the 8-h day length and population density of 60 ind.·L−1 (). An increase in day length and population density weakened the effects of temperature–food interactions ().
Table2. Summary of the two-way ANOVA results on the effects of temperature and food concentration on D. carinata fitness.
Higher population density significantly depressed D. carinata fitness at four combinations of two photoperiods and two food concentrations (two-way ANOVA, p < 0.001 for all, ). The interactions between temperature and population density were significant at the high food concentration (1000 μg·C·L−1 with either 8:16 or 16:8 photoperiods, two-way ANOVA, p < 0.05 for both) as the temperature effects were more pronounced at the population density of 60 ind.·L−1 () but not significant at the low food concentration (two-way ANOVA, p > 0.05 for both, ). The η2 indicated that the interactive effects were almost the same between the two photoperiod treatments at 1000 μg·C·L−1 (). The population density shaped the magnitude of temperature reaction norms of D. carinata fitness (the absolute value of the slope) but did not change the nature of temperature reaction norms (the sign of the slope, ).
Table3. Summary of the two-way ANOVA results on the effects of temperature and population density on D. carinata fitness.
Figure2. Effects of population density on temperature reaction norms of D. carinata fitness in the four combinations of two photoperiods and two food concentrations.
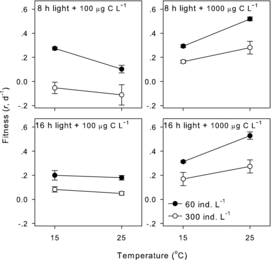
Increasing day length significantly enhanced D. carinata fitness only in one combination of 300 ind.·L−1 and 100 μg·C·L−1 (two-way ANOVA, p < 0.001). It did not significantly affect D. carinata fitness in the other three combinations of two population densities and two food concentrations (two-way ANOVA, p > 0.05 for all, ). The interaction between temperature and photoperiod was only significant in one combination of 60 ind.·L−1 and 100 μg·C·L−1 (two-way ANOVA, p < 0.001). Temperature effects were more pronounced under the 8-h day length, but the nature of temperature reaction norms was not changed by photoperiod ().
Table4. Summary of the two-way ANOVA results on the effects of temperature and photoperiod on D. carinata fitness.
Discussion
Confirming general expectations, temperature, food concentration, population density, and photoperiod significantly affected D. carinata fitness in this study, yet the temperature–food concentration interaction was quite intriguing in all combinations of two population densities and two photoperiods. In addition, the temperature–population density and temperature–photoperiod interactions were also significant in some, although not all, combinations tested. These results clearly show that temperature reaction norms of D. carinata fitness were influenced by other variables. Furthermore, the impacts of these variables were not consistent. The complex interactions of four variables (temperature, food concentration, population density, and photoperiod) probably reflect adaptive responses of D. carinata that maximize fitness within multiple conditions of their environments. Consequently, an accurate prediction of warming effects on zooplankton is likely to require an understanding of the interactions between temperature and other ecological variables.
Food concentration not only altered the intensity of temperature reaction norms, but also changed the slope from positive to negative. Such fitness decreases with increasing temperature at the low food concentration is likely due to the increased energy consumption with enhanced temperature. The low food supply (100 μg·C·L−1) that supported D. carinata growth and reproduction at 15 °C might be insufficient at 25 °C. This result is consistent with the previous finding that the combined effect of temperature and low food supply resulted in negative production in the ciliate U. farcta (Weisse et al. Citation2002). Thus, the effect of increased temperature at low food resource may result in a counterintuitive decrease in zooplankton productivity. In addition, the temperature effects on D. carinata fitness were more pronounced at the high food concentration (). Water warming may have a stronger impact on zooplankton in spring when nutritious diatoms and green algae are abundant, in contrast to summer when edible phytoplankton are scarce. Similarly, the effects of water warming on zooplankton may be stronger in eutrophic lakes than oligotrophic lakes due to the interactions between temperatures and food concentrations.
In contrast to food concentration, population density and photoperiod only modified the intensity of temperature reaction norms of D. carinata fitness. The high population density overall resulted in a fitness decrease if considered alone, which agreed with the previous observations (Burns Citation2000; Lürling et al. Citation2003). However, when the population density and temperature were combined, the average response to high population density was to weaken the temperature–food interaction on D. carinata fitness. High population density can reduce zooplankton feeding, growth, and reproduction directly by altering behavioral and physiological responses or indirectly by reducing food resources (Burns Citation2000; Lürling et al. Citation2003). Given that flow-through systems were used to maintain algal concentrations in the present study, the possible reason may be the depression of feeding rates by inhibitory infochemicals rather than the rapid consumption of food resources (Lürling et al. Citation2003). Low food intake caused by high population density may constrain metabolism activity in D. carinata when exposed to high temperatures and low food concentrations.
Although the photoperiod influenced D. carinata fitness, there was only one significant temperature–photoperiod interaction in the four test combinations that reduced the effect of increasing temperature on fitness when D. carinata was grown at the low population density and low food concentration. In this situation, the long day length (16:8 light: dark) masked the temperature effects on D. carinata fitness at the low food concentration. Similarly, Martínez-Jerónimo Citation(2012) reported that a longer photoperiod (16:8) weakened the effect of the higher temperature (25 °C) on the maximum length and age at first reproduction of D. magna. The mechanism of photoperiod effects on daphniid fitness is not clear. It may relate to hormonal regulations that contribute to the shift from parthenogenesis to sexual reproduction (Korpelainen Citation1986; Alekseev and Lampert Citation2004). The reduced temperature effects under the long day length suggest that water warming may have a stronger influence on zooplankton in winter (8:16 light:dark) than summer (16:8 light:dark). The combination of the depressed temperature effects on D. carinata fitness due to the low food concentration or long photoperiod implies that zooplankton in summer, even with the maximum temperature, may not suffer the most pronounced effects of warming.
In addition to the significant effects of food concentration, population density, and photoperiod on temperature reaction norms, our results clearly demonstrate that the interactions between temperature and one variable were affected by the other variables. The temperature–food concentration interactions on D. carinata fitness were significant in the four treatments of two population densities and two photoperiods but their interactive intensity greatly varied. The temperature–population density interactions were significant in two of the four food concentration–photoperiod treatments. Similarly, the temperature–photoperiod interaction was significant only in one of the four treatments of two population densities and two photoperiods. Therefore, the effects of food concentration, population density, and photoperiod on temperature reaction norms may depend on other components of aquatic systems and considering the interactions between two variables may not adequately reflect the real zooplankton responses to global warming in the field. The previous studies have revealed the combined effects of two variables on zooplankton fitness and implied the importance of incorporating more variables (Orcutt and Porter Citation1984; Giebelhausen and Lampert Citation2001; Martínez-Jerónimo Citation2012). However, there are few studies considering three or more variables simultaneously in the literature. One exception is Pajk et al. Citation(2012) who demonstrated that Daphnia fitness was affected not only by assay food quality and assay temperature, but also by maternal food quality and maternal temperature. These results suggest that simultaneously investigating multiple variables would provide more insights into understanding zooplankton responses to environmental changes.
Studies on the interactive effects of temperature and food on zooplankton fitness (Orcutt and Porter Citation1984; Giebelhausen and Lampert Citation2001; Persson et al. Citation2011), even an unexpected decrease in zooplankton growth with the increasing temperature at low food levels (Weisse et al. Citation2002), have provided more knowledge for the accurate prediction of zooplankton responses to global warming (Thuiller Citation2007). We further investigated the effects of more ecological variables on temperature reaction norms and more complicated interactions among three and four variables. However, there are some limitations to this study. First, we recognize that our results are based on two points of each variable and lack the precise resolution to adequately assess the entire shape of the numerical response. Thus, our suggestions on the effects of food concentration, population density, and photoperiod on temperature reaction norms are speculative and need further investigation. Second, only one clone of D. carinata was tested. Considerable evidence exists for clonal- and species-specific responses in daphniids (Jiang et al. forthcoming Citation2013). For example, the responses of physiological variables to temperature–food quality interactions reported by McFeeters and Frost Citation(2011) were restricted to one of the two Daphnia species studied. Further studies are needed to investigate whether the effects of ecological variables in temperature reaction norms are clonal and/or species specific. Finally, although four variables were tested simultaneously, many important factors have not been considered in this study, for example, pH, dissolved oxygen, pollutants, competition, or predation. The real effect of increasing temperature on zooplankton in nature will depend on complicated interactions of a whole suite of environmental factors. Considering the importance of zooplankton in aquatic food webs and the ongoing interest in global warming, there is clearly a need for further investigation into the combined effects of temperature with other ecological variables.
Acknowledgements
This paper was supported by the Special Fund for Agro-Scientific Research in the Public Interest (201203065), the National Science Foundation of China (31272307 and 31172043), and the Fundamental Research Funds for the Central Universities.
References
- Achenbach L, Lampert W. 1997. Effects of elevated temperatures on threshold food concentrations and possible competitive abilities of differently sized cladoceran species. OIKOS. 79:469–476.
- Alekseev V, Lampert W. 2004. Maternal effects of photoperiod and food level on life history characteristics of the cladoceran Daphnia pulicaria Forbes. Hydrobiologia. 526:225–230.
- Burns CW. 1969. Relation between filtering rate, temperature, and body size in four species of Daphnia. Limnology and Oceanography. 14:693–700.
- Burns CW. 2000. Crowding-induced changes in growth, reproduction and morphology of Daphnia. Freshwater Biology. 43:19–29.
- Cáceres T, He W, Naidu R, Megharaj M. 2007. Toxicity of chlorpyrifos and TGP alone and in combination to Daphnia carinata: the influence of microbial degradation in natural water. Water Research. 41:4497–4503.
- Carpenter SR, Kitchell JF. 1987. The temporal scale of variance in limnetic primary production. American Naturalist. 129:417–433.
- Claska ME, Gilbert JJ. 1998. The effect of temperature on the response of Daphnia to toxic cyanobacteria. Freshwater Biology. 39:221–232.
- Deng D, Xie P, Zhou Q, Yang H, Guo L, Geng H. 2008. Field and experimental studies on the combined impacts of cyanobacterial blooms and small algae on crustacean zooplankton in a large, eutrophic, subtropical, Chinese lake. Limnology. 9:1–11.
- Giebelhausen B, Lampert W. 2001. Temperature reaction norms of Daphnia magna: the effect of food concentration. Freshwater Biology. 46:281–289.
- Grant JWG, Bayly IAE. 1981. Predator induction of crests in morphs of the Daphnia carinata King complex. Limnology and Oceanography. 26:201–218.
- Hall CJ, Burns CW. 2002. Mortality and growth responses of Daphnia carinata to increases in temperature and salinity. Freshwater Biology. 47:451–458.
- Jakobsen PJ, Johnsen GH. 1988. The influence of food limitation on swarming behavior in the waterflea Bosmina longispina. Animal Behaviour. 36:991–995.
- Jiang X, Liang H, Yang W, Zhang J, Zhao Y, Chen L, Zhao S, Jing X. 2013a. Fitness benefits and costs of induced defenses in Daphnia carinata (Cladocera: Daphnidae) exposed to cyanobacteria. Hydrobiologia. 702:105–113.
- Jiang X, Yang W, Zhao S, Liang H, Zhao Y, Chen L, Li R. 2013b. Maternal effects of inducible tolerance against the toxic cyanobacterium Microcystis aeruginosa in the grazer Daphnia carinata. Environmental Pollution 178:142–146.
- Jiang X, Zhang L, Liang H, Li Q, Zhao Y, Chen L, Yang W, Zhao S. Forthcoming 2013. Resistance variation within a Daphnia pulex population against toxic cyanobacteria. Journal of Plankton Research. doi:10.1093/plankt/fbt062
- Kvam OV, Kleiven OT. 1995. Diel horizontal migration and swarm formation in Daphnia in response to Chaoborus. Hydrobiologia. 307:177–184.
- Kee DM, Ebert D. 1996. The effect of temperature on maturation threshold body-length in Daphnia magna. Oecologia. 108:627–630.
- Korpelainen H. 1986. The effects of temperature and photoperiod on life history parameters of Daphnia magna (Crustacea: Cladocera). Freshwater Biology. 16:615–620.
- Lampert W. 1978. A field study on the dependence of the fecundity of Daphnia spec. on food concentration. Oecologia. 36:363–369.
- Lampert W, Trubetskova I. 1996. Juvenile growth rate as a measure of fitness in Daphnia. Functional Ecology. 10:631–635.
- Lürling M, Roozen F, van Donk E, Goser B. 2003. Response of Daphnia to substances released from crowded congeners and conspecifics. Journal of Plankton Research. 25:967–978.
- Martínez-Jerónimo F. 2012. Description of the individual growth of Daphnia magna (Crustacea: Cladocera) through the von Bertalanffy growth equation. Effect of photoperiod and temperature. Limnology. 13:65–71.
- McFeeters BJ, Frost PC. 2011. Temperature and the effects of elemental food quality on Daphnia. Freshwater Biology. 56:1447–1455.
- Orcutt JD, Porter KG. 1984. The synergistic effects of temperature and food concentration on life history parameters of Daphnia. Oecologia. 63:300–306.
- Pajk F, von Elert E, Fink P. 2012. Interaction of changes in food quality and temperature reveals maternal effects on fitness parameters of a keystone aquatic herbivore. Limnology and Oceanography. 57:281–292.
- Persson J, Wojewodzic MW, Hessen DO, Andersen T. 2011. Increased risk of phosphorus limitation at higher temperatures for Daphnia magna. Oecologia. 165:123–129.
- Starkweather PL. 1976. Influences of light regime on postembryonic development in two strains of Daphnia pulex. Limnology and Oceanography. 21:830–837.
- Starkweather PL. 1983. Daily patterns of feeding behavior in Daphnia and related microcrustacea: implications for cladoceran autecology and the zooplankton community. Hydrobiologia. 100:203–221.
- Thuiller W. 2007. Climate change and the ecologist. Nature. 448:550–552.
- Weisse T, Stadler P, Lindström ES, Kimmance SA, Montagnes DJS. 2002. Interactive effect of temperature and food concentration on growth rate: a test case using the small freshwater ciliate Urotricha farcta. Limnology and Oceanography. 47:1447–1455.
- Wojtal-Frankiewicz A. 2012. The effects of global warming on Daphnia spp. population dynamics: A review. Aquatic Ecology. 46:37–53.