Abstract
Mitigation wetlands are intended to replace the ecological conditions of wetlands lost to development or drainage, resulting in a theoretical expectation that the replacement wetland will support a species composition and diversity comparable to a reference standard. I analyze a freshwater mitigation wetland in central Ohio, USA to evaluate factors that influence spatial patterns of plant community assembly in terms of floristic quality and diversity. Soil seed bank, bulk density, organic content, and pH are evaluated, along with average depth, exposure time, depth variance, and spatial location within the wetland basins, for their relationship with plant community characteristics in the first three years after wetland creation. The plant community was highly variable during the study period, with general trends toward greater diversity and more hydrophilic vegetation. The spatial distribution of nonnative cover, planted cover, mean coefficient of conservatism, and diversity in the third-year plant community were unrelated to soil and seed bank metrics; however, all were strongly related to hydrologic metrics. Shallow depth (<0.2 m) and moderate depth variance were found to facilitate plant community diversity, while floristic quality is more likely in deep (>0.2 m) and relatively stable water levels (<60% exposure time). Nonnative vegetation cover was particularly high at shallow locations with high depth variance and long exposure times. Geographic analysis of floristic quality and diversity indicates a weak spatial correlation between the two, suggesting that these two measures of the plant community are unlikely to coincide in early succession. The implications are that site design and hydrologic manipulation can drive the early successional vegetation characteristics, and that site design, compliance criteria, and monitoring regimes should seek to concertedly facilitate species composition, community diversity, or both.
Introduction
Over four decades ago, compensatory wetland mitigation was proposed as a regulatory mechanism for wetland conservation in the United States (USACE Citation1973; USEPA Citation1980; Hough & Robertson Citation2009). Since its inception, the mitigation concept has survived a steady barrage of criticism from scientists and practitioners to become firmly entrenched as national policy. Both the policy and practice of wetland mitigation have evolved over the years and a significant milestone was achieved in 2008 with the release of the Final Rule for Compensatory Mitigation for Losses of Aquatic Resources by the Army Corps of Engineers and the United States Environmental Protection Agency (CFR Citation2008). With this attempt to clarify and standardize rules, procedures, and expectations, compensatory mitigation reached a new degree of maturity. Many aspects of the 2008 Ruling have been commented on elsewhere (Gardner et al. Citation2009; Murphy et al. Citation2009); here, my focus is on practical aspects of the Rule's reaffirmation that mitigation compliance is to be evaluated according to ecological condition. While the Rule does not mandate specific metrics, it defines ecological condition as ‘the relative ability of an aquatic resource to support and maintain a community of organisms having a species composition, diversity, and functional organization comparable to reference aquatic resources in the region’ (CFR Citation2008). For many mitigation projects, this means the rapid assembly of appropriate flora. In this study, I analyze wetland attributes that influence the early successional assembly of vegetation composition and diversity.
Plant community composition is a useful indicator of ecosystem integrity (Cronk & Fennessy Citation2001). A suitable community is typically characterized by dominance of plant species of high floristic quality (i.e., native and not aggressive) that occur in natural wetlands of the region. Thus, most mitigation performance criteria include the establishment of a desirable wetland plant community, commonly measured in a transect- or quadrat-based estimate of percent cover and a measure of floristic quality like the coefficient of conservatism (Matthews & Endress Citation2008). The desirable plant community can be facilitated, to some degree, by manipulation of the hydrologic regime (Zedler Citation2000; Simenstad et al. Citation2006), by amendment of substrate and seed bank (Brown & Bedford Citation1997), and by introduction of certain plant species and management of undesirable species (Matthews & Endress Citation2008; Matthews & Spyreas Citation2010). A potential trade-off in this quest for the desirable plant community is the loss of heterogeneity; indeed, establishment and maintenance of a particular plant community can mean regularization of the disturbance regime and minimization of natural recruitment (Zedler Citation2000; Brooks et al. Citation2005).
Vegetation diversity is, in theory, a proxy for a suite of ecological functions such as nutrient processing, habitat provision, functional redundancy, and resilience. Given its relationship with these attributes, it would seem logical for diversity to be a primary performance standard for mitigation wetlands; in practice, however, it rarely is (Matthews & Endress Citation2008). Early successional diversity in mitigation wetlands is often limited by dispersal issues (e.g., landscape isolation), by the paucity of a wetland seed bank, and preemption by aggressive invaders (Zedler Citation2000; Seabloom & van der Valk Citation2003). Furthermore, the relationship between diversity and particular ecosystem services is not entirely clear; some services, in fact, are better supported by low diversity systems (Zedler Citation2000). Consequently, while diversity is not incompatible with the establishment of a specific plant community, conditions that facilitate diversity – variable disturbance regime, spatial and temporal heterogeneity, high rate of species immigration, soil nutrient availability, and organic content – may hinder a short-term mitigation compliance effort and not be worth the risk (Zedler Citation2000).
As two desired outcomes of compensatory mitigation, plant community composition and diversity are different ends that may be achieved with different means. In this study, I evaluate factors that potentially influence vegetation establishment in early succession and I assess the degree to which the desirable outcomes of floristic quality and diversity are compatible within the constraints of these factors. The study is based on the first three years of vegetation development in the Dutch Fork Wetland, a constructed freshwater mitigation wetland in central Ohio, USA. I use the spatial variability of the developing plant community and potentially influential factors to test three hypotheses. First, do desirable characteristics of the plant community differ spatially according to soil conditions, hydrologic conditions, seed bank conditions, or proximity to basin inflow? Second, do plant community characteristics differ by degree of hydrologic variation? And finally, do high-quality community composition and diversity coincide spatially within the wetland basins?
Methods
Site description
The Dutch Fork Wetland is comprised of six freshwater emergent basins of about 5 ha total at Dawes Arboretum in Licking County, Ohio (39° 59′ 41.92′′ N, 82° 25′ 07.27′′ W). The wetland complex was created in 2006 on former agricultural land to compensate for the loss of various wetlands in the expansion of State Route 161 by the Ohio Department of Transportation. The soils of the wetland basins are primarily Luray silty clay loam, but also include Ockley silt loam, Bennington silt loam, and Centerburg silt loam. The basins are separated by raised berms (approximately 1 m above average water level) and are connected sequentially in a gravity flow-through design. Basin 1 (0.53 ha) has two inflows; one conveys overflow from a groundwater- and runoff-fed pond to the north of the wetlands and the other is a ditch that receives runoff from and tile drainage from the northeast. Basins 2 (0.44 ha) and 3 (0.88 ha) receive water, in turn, through subsurface corrugated pipes with adjustable weirs. Basins 4–6 were flooded only sporadically during the study and are not considered in this analysis.
The wetland basins were planted with more than 40,000 seedlings and rootstock in 2006 and 2007. Spot management of invasive species by mechanical removal was also conducted during the study period. Dominant native species during the study period included Schoenoplectus tabernaemontani (C.C. Gmel.) Palla (softstem bulrush), Sagittaria latifolia Willd. (broadleaf arrowhead), and Alisma subcordatum Raf. (American water plantain), while common invasive species included Marsilea quadrifolia L. (European waterclover), Typha xglauca Godr. (cattail), and Polygonum persicaria L. (spotted ladysthumb).
Soil and hydrologic measurements
Sampling points were established in a grid of approximately 10 m while conforming to the shape of each basin, resulting in 73 sampling points in Basin 1, 46 in Basin 2, and 84 in Basin 3 (). In 2007, 2 cm diameter soil cores were taken to a depth of 10 cm at each grid point. Soil samples were dried in a Model 40 GC Lab Oven at 100 °C for 24 hr. To determine bulk density (g/cm3), each dried sample was individually weighed and divided by soil volume (31.42 cm3). Soil samples were then ground, homogenized, and divided into 2- and 10-g subsamples. The 2-g samples were used to measure percent organic matter and the 10-g samples were used to measure soil pH. Percent organic matter was determined by placing samples in porcelain crucibles in a muffle furnace and bringing them first to 375 °C for 1 hr, then raising the temperature to 550 °C for 16–20 hr (Carter Citation1993). Cooled soil was weighed and percent organic matter was determined based on mass lost. Soil pH was determined by adding 20 mL of CaCl2 to the soil samples in 200-mL beakers. The samples were stirred using an orbital shaker for 30 min at 150 rpm and then allowed to settle for 1 hr following Carter Citation(1993). A calibrated model IQ240 pH meter (Hach Co., http://www.hach.com) was immersed into the supernatant and soil pH was recorded.
Figure 1. Morphology of Basins 1–3 of the Dutch Fork Wetland, Licking County, Ohio. Points indicate sampling locations for soil, vegetation, and hydrologic metrics. Contour lines (0.2 m interval) indicate mean depth, 2007–2009.
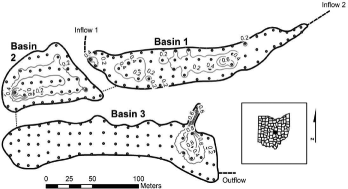
The water level of each basin was recorded hourly from 1 January 2007 through 9 November 2009 with Solinst Leveloggers® (Solinst Canada Ltd., http://www.solinst.com/index.html), calibrated to grid point sampling locations with a staff gage in each basin and depth measurements at each grid point. For each grid point, I then calculated the average depth over this period (), the percentage of time exposed (depth <1 cm), the depth variance (S2), expressed as
, and the coefficient of variance,
.
Macrophyte and seed bank measurements
In July 2007–2009, plant species were identified and percent cover estimated within a 5 m2 circle (1.26 m radius) centered on each grid point. Plant cover estimation was stratified so that the total percent cover could exceed 100%. Plant specimens were identified to genus and species when possible, according to Crow and Hellquist Citation(2000), Fassett Citation(1957), and Newcomb Citation(1977). Plants were also categorized as ‘planted’ according to the list of introduced species provided by Dawes Arboretum and as native or nonnative according to Andreas et al. Citation(2004). For each grid point in each year, I determined the prevalence index (PI), the average coefficient of conservatism (), and Simpson's diversity index (1 − D). PI is a cover-weighted average of wetland indicator status, where Obligate Wetland = 1, Facultative Wetland = 2, Facultative = 3, Facultative Upland = 4, Obligate Upland = 5 (Wentworth et al. Citation1988; Balcombe et al. Citation2005); indicator status was assigned according to Reed Citation(1988). The coefficients of conservatism are regionally specific numeric rankings of the capacity of each plant species to tolerate environmental perturbation, ranging from zero for invasive species and all nonnative species to 10 for native species that are tolerant only to a narrow, specialized niche (Andreas & Lichvar Citation1995). I used the plant species
list developed for Ohio by Andreas et al. Citation(2004). I calculated the diversity of each sampling location in each year according to Simpson Citation(1949), expressed as 1 − D, where D = Σ(pi)2 and pi is the proportion of the ith taxon of each collection event.
In June 2008, four 50-cm3 soil samples were taken in each basin (at the inflow, outflow, shallow location, and deep location). The soil samples were taken from within the top 10 cm of soil following Bigwood and Inouye Citation(1988) and Touzard et al. Citation(2002). The samples were kept in a cooler immediately after they were taken in order to protect them from the sunlight and intense heat. Soil samples were brought back to the lab and run through a sieve in order to concentrate the seeds following Ter Heerdt et al. Citation(1996), with sieve size mesh openings of 4.75, 1.52, 1.02, 0.76, and 0.38 mm. Each sample was planted with potting soil in small trays and placed in the Denison University Greenhouse to germinate. This process was repeated five times for each sample site; all repetitions were conducted from 28 May to 30 June 2008. Each sample was kept in the greenhouse for two weeks. All seedlings were allowed to grow until they could be identified according to Fassett Citation(1957), Symonds Citation(1963), Newcomb (1977), and Crow and Hellquist Citation(2000).
Statistical and spatial methods
Mean PI, 1 − D, , percent planted cover, percent native cover, and average depth were compared among years in each basin with one-way analysis of variance (ANOVA) and Tukey's pairwise comparison. Four parameters of the 2009 plant community – percent nonnative cover, planted percent cover, 1 − D, and cover-weighted
– were evaluated according to 2007–2009 hydrologic classifications for average depth (<0.05 m, 0.05–0.2 m, >0.2 m), percent time exposed (<20%, 20–60%, >60%), and coefficient of variance (<0.05, 0.05–0.1, >0.1), and according to 2007 soil classifications for bulk density (<1.25 g/cm3, 1.25–.75 g/cm3, >1.75 g/cm3), pH (<5.5, 5.5–6.5, >6.5), and percent organic matter (<5.5, 5.5–7.5, >7.5). Plant community characteristics in these categories were compared with one-way ANOVA and Tukey's pairwise comparison. Seed bank samples (2008) were pooled for each basin and compared with the 2009 macrophyte community with Sørenson's similarity index (Sørenson Citation1948). ANOVAs were performed with SPSS® v.19 and α = 0.05. Significant differences in all ANOVA tests were confirmed with the nonparametric Kruskal–Wallis H test, and pairwise comparisons were confirmed with Dunnett's C post hoc test, which does not assume equal variances. Spatial analysis of the 2009 plant community was performed with ArcMap® 10.1 using spatial analyst and inverse distance weighted interpolation. Simpson's 1 − D and cover-weighted
were compared for the 2009 plant community of each basin with the geographically weighted regression function of ArcGIS 10.1.
Results
Descriptive statistics
Average depths in the three basins ranged seasonally from approximately 0.05 to 0.6 m (). Water levels were significantly higher in 2008 than in 2007 for all basins (). Due to basin morphology (), sampling locations in Basin 2 were on average the least exposed and the least variable in terms of depth, while those of Basin 3 were the most exposed and most variable (). The soils of Basin 3 were higher in organic matter and lower in bulk density and pH than those of Basins 1 and 2 (). The macrophyte communities of all three basins varied significantly from year to year; general trends were for increasing diversity and decreasing PI from 2007 to 2009 (). Floristic quality trends were not as clear, as cover of planted species and nonnative species changed dramatically over the study period. The 2008 seed bank was composed of more nonnative and facultative species than the 2009 macrophyte community, making the seed bank and macrophyte communities quite dissimilar ().
Table 1. Vegetation, soil, hydrologic, and seed bank metrics for Basins 1–3 of the Dutch Fork Wetland, 2007–2009. Bold sets indicate significant differences for each basin at α = 0.01** and α = 0.05*.
Plant community analyses – 2009
Characteristics of the 2009 plant community were not significantly related to the spatial variation in soil bulk density, percent organic matter, or soil pH. Nor were 2009 vegetation characteristics distributed according to distance from basin inflow. The 2007–2009 hydrologic regime was significantly related to 2009 plant community characteristics in a number of ways (). Nonnative cover decreased with depth (F2,209 = 8.2; p < 0.001) and increased with both time exposed (F2,209 = 9.1; p < 0.001) and coefficient of depth variance (F2,209 = 15.8; p < 0.001), while planted cover was the greatest at intermediate depth, exposure and coefficient of depth variance (A and B). Similarly, Simpson's diversity was the greatest at sampling locations with intermediate depth (F2,209 = 10.3; p < 0.001), exposure (F2,209 = 6.3; p = 0.02), and coefficient of depth variance (F2,209 = 12.4; p < 0.001; C). The cover-weighted , a measure of floristic quality, increased significantly with depth (F2,209 = 9.3; p < 0.001) and decreased with increasing exposure (F2,209 = 14.2; p < 0.001) and increasing coefficient of depth variance (F2,209 = 25.8; p < 0.001). Inverse distance-weighted interpolation () for Simpson's 1 − D and cover-weighted
in the 2009 plant community revealed poor spatial correlation in Basin 1 (r2 = 0.34), Basin 2 (r2 = 0.10), and Basin 3 (r2 = 0.10).
Figure 3. Evaluation of (A) nonnative plant percent cover per 5 m2, (B) planted species percent cover per 5 m2, (C) Simpson's diversity index (1 − D) per 5 m2, and (D) cover-weighted coefficient of conservatism () per 5 m2 of the 2009 Dutch Fork Wetland sampling locations in Basins 1–3 according to hydrologic classification, mean ± SE. Letters indicate significant differences in each graph and correspond with ANOVA results provided.
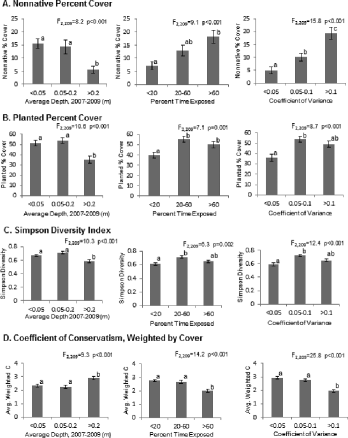
Discussion
The Dutch Fork Wetland plant community was highly variable in the first three years following construction. While all three basins trended toward a more diverse and more hydrophilic community, nonnative species clearly influenced the overall floristic quality in the third year, particularly in Basins 1 and 3. Such variability is common in early successional mitigation wetlands; generally, species influx is much higher in newly created and restored wetlands than in natural systems but this influx declines over the first six years (Spieles Citation2005). The Dutch Fork data of this study provide a means of assessing the relative importance of soil, seed bank, hydrologic regime, and proximity to inflow for plant community assortment in the third year. None of the third year plant community characteristics differed spatially according to soil organic matter, pH, or bulk density. Matthews et al. Citation(2009) found soil organic content and pH to be moderate but significant predictors of plant community composition and floristic quality in a study of 28 restored wetlands in Illinois. Similarly, Kirkman et al. Citation(2000) found an association of soil organic content with ground flora community characteristics in Georgian swamps and marshes. It may be that the ranges of soil parameters in this study were not large enough to influence the plant community or it may be that soil effects were dominated by hydrologic effects as Weiher et al. Citation(1996), Kirkman et al. Citation(2000), and Matthews et al. Citation(2009) found or by nutrient availability and limitation (Bedford et al. Citation1999). The seed bank and proximity to inflow (a measure of hydrochory effects) were not found to be important factors in the third-year Dutch Fork plant community with a seed bank–macrophyte community similarity of less than 4%. In a meta-analysis of 42 wetland studies, Hopfensperger Citation(2007) found an average 47% similarity of seed bank to macrophyte community in a wide range of natural wetland environments; clearly, the seed bank is not yet such an important factor in the young created wetland of the present study. It is reasonable to expect that soil and seed bank will influence the macrophyte community to a greater extent as the Dutch Fork Wetland ages.
At this early successional stage, variability in the hydrologic regime is clearly an important factor in plant community development. This is widely noted elsewhere: Matthews et al. Citation(2009) found water depth to be the greatest local factor on plant community characteristics in restored wetlands; Fennessy et al. Citation(1994) found water depth to be the primary driver of plant community composition in created freshwater wetlands; Weiher et al. Citation(1996) found flooding to be a main determinant of the plant community in wetland mesocosms. The vegetation response to the hydrologic regime at the Dutch Fork Wetland adds nuance to our understanding of the relative importance of depth, duration, and frequency of flooding on the plant community. In particular, the results suggest that intermediate depth variance and exposure facilitate plant community diversity, while floristic quality is more likely in relatively deep and stable environments. These results are corroborated by Casanova and Brock Citation(2000) who used mesocosms with various hydrologic regimes as factors of early successional wetland plant community assembly. They found that depth, duration, and frequency of flooding all play a role in plant community formation while duration and frequency particularly promoted community segregation. Stable depths, according to Casanova and Brock Citation(2000), select for a single plant community, while fluctuating inundation and dry periods select for a greater diversity of species, including a greater incidence of nonnative species. The Dutch Fork Wetland results advance these concepts by showing that areas of greater diversity and areas of greater floristic quality can both be present but spatially noncoincident within the same wetland complex.
The implications of this study are twofold. First, the 2008 Rule's call for replacement of both a specific community and a diverse community (CFR Citation2008) may be difficult to achieve for any given wetland locus. Of course, the Rule does not state that both must be achieved in one place, or that either must necessarily be achieved at all. Still, in mitigation practice, it is clear that the specific community (i.e., a community dominated by desirable species and lacking undesirable species) is much more common as a compliance target than a diverse community (Matthews & Endress Citation2008). Results of this study suggest that a specific community is best achieved on the local scale with a deep, stable, and hydrologic regime but that such a design could come at the expense of community diversity (Brooks et al. Citation2005). Second, while the Dutch Fork results demonstrate that diversity and floristic quality may coexist in a hydrologically heterogeneous design, they also amplify the need for compliance criteria and monitoring schemes that account for and reward both community quality and diversity. Beyond the mandated monitoring period, it is likely that both quality and diversity will be necessary to achieve long-term positive functional outcomes of mitigation.
Acknowledgements
The access and assistance provided by Dawes Arboretum is gratefully acknowledged. Thanks to Simonne Benoit, Miranda Carter, Melissa Kennedy, Xije Lv, Jackson Means, Jordan Mora, Kyle Plummer, and the Denison University Departments of Biology and Geosciences for field and lab assistance.
Additional information
Funding
References
- Andreas BK, Lichvar RW. 1995. Floristic index for establishing assessment standards: a case study of northern Ohio. Wetlands Research Program Technical Report WRP-DE-8. Vicksburg (MS): U.S. Army Corps of Engineers, Waterways Experiment Station.
- Andreas BK, Mack JJ, McCormac JS. 2004. Floristic Quality Assessment Index (FQAI) for vascular plants and mosses for the state of Ohio. Columbus (OH): Ohio Environmental Protection Agency, Division of Surface Water, Wetland Ecology Group; p. 219.
- Balcombe CK, Anderson JT, Fortney RH, Rentch JS, Grafton WN, Kordek WS. 2005. A comparison of plant communities in mitigation and reference wetlands in the Mid-Appalachians. Wetlands. 25(1):130–142.
- Bedford BL, Walbridge MR, Aldous A. 1999. Patterns in nutrient availability and plant diversity of temperate North American wetlands. Ecology. 80(7):2151–2169.
- Bigwood DW, Inouye DW. 1988. Spatial pattern analysis of seed banks: an improved method and optimized sampling. Ecology. 69(2):497–507.
- Brooks RP, Wardrop DH, Cole CA, Campbell DA. 2005. Are we purveyors of wetland homogeneity? A model of degradation and restoration to improve wetland mitigation performance. Ecol Eng. 24:331–340.
- Brown SC, Bedford BL. 1997. Restoration of wetland vegetation with transplanted wetland soil: an experimental study. Wetlands. 17(3):424–437.
- Carter MR, editor. 1993. Soil sampling and methods of analysis. Boca Raton (FL): Lewis Publishers; p. 823.
- Casanova MT, Brock MA. 2000. How do depth, duration and frequency of flooding influence the establishment of wetland plant communities? Plant Ecol. 147:237–250.
- [CFR] Code of Federal Regulations. 2008. Compensatory mitigation for losses of aquatic resources: final rule. Department of Defense, Department of the Army, Corps of Engineers, and Environmental Protection Agency. 33 C.F.R. § 332; 40 C.F.R. § 230
- Cronk J, Fennessy MS. 2001. Wetland plants: biology and ecology. Boca Raton (FL): CRC Press; p. 462.
- Crow GE, Hellquist CB. 2000. Aquatic and wetland plants of northeastern North America, Volumes One and Two. Madison (WI): The University of Wisconsin Press.
- Fassett NC 1957. A manual of aquatic plants. Madison (WI): The University of Wisconsin Press.
- Fennessy MS, Cronk JK, Mitsch WJ. 1994. Macrophyte productivity and community development in created freshwater wetlands under experimental hydrological conditions. Ecol Eng. 3(4):469–484.
- Gardner RC, Zedler J, Redmond A, Turner RE, Johnston CA, Alvarez VR, Simenstand CA, Prestegaard KL, Mitsch WJ. 2009. Compensating for wetland losses under the Clean Water Act (redux): evaluating the federal compensatory mitigation regulation. Stetson Law Rev. 38(2):213–249.
- Hopfensperger KN. 2007. A review of similarity between seed bank and standing vegetation across ecosystems. Oikos. 116:1438–1448.
- Hough P, Robertson M. 2009. Mitigation under Section 404 of the Clean Water Act: where it comes from, what it means. Wetlands Ecol Manag. 17:15–33.
- Kirkman LK, Goebel PC, West L, Drew MB, Palik BJ. 2000. Depressional wetland vegetation types: a question of plant community development. Wetlands. 20(2):373–385.
- Matthews JW, Endress AG. 2008. Performance criteria, compliance success, and vegetation development in compensatory mitigation wetlands. Environ Manag. 41:130–141.
- Matthews JW, Peralta AL, Flanagan DN, Baldwin PM, Soni A, Kent AD, Endress AG. 2009. Relative influence of landscape vs. local factors on plant community assembly in restored wetlands. Ecol Appl. 19(8):2108–2123.
- Matthews JW, Spyreas G. 2010. Convergence and divergence in plant community trajectories as a framework for monitoring wetland restoration progress. J Appl Ecol. 47:1128–1136.
- Murphy J, Goldman-Carter J, Sibbing J. 2009. New mitigation rule promises more of the same: why the new Corps and EPA mitigation rule will fail to protect our aquatic resources adequately. Stetson Law Rev. 38(2):311–336.
- Newcomb L 1977. Newcomb's wildflower guide. Boston (MA): Little, Brown and Company; p. 490.
- Reed PB 1988. National list of plant species that occur in wetlands: 1988 national summary. United States Department of the Interior, Fish and Wildlife Service, Washington, DC. Biol Rep. 88(24):1–244.
- Seabloom EW, van der Valk AG. 2003. Plant diversity, composition, and invasion of restored and natural prairie pothole wetlands: implications for restoration. Wetlands. 23(1):1–12.
- Simenstad C, Reed D, Ford M. 2006. When is restoration not? Incorporating landscape-scale processes to restore self-sustaining ecosystems in coastal wetland restoration. Ecol Eng. 26:27–39.
- Simpson EH. 1949. Measurements of diversity. Nature. 163: 688.
- Sørenson T. 1948. A method of establishing groups of equal amplitude in plant sociology based on similarity of species content and its application to analyses of the vegetation on Danish commons. Biol Skr (K. Danske Vidensk. Selsk. NS). 5:1–34.
- Spieles DJ. 2005. Vegetation development in created, restored, and enhanced mitigation wetland banks of the United States. Wetlands. 25(1):51–63.
- Symonds GWD. 1963. The shrub identification book. New York, (NY): Harper Collins.
- Ter Heerdt GNJ, Verweij GL, Bekker RM, Bakker JP. 1996. An improved method for seed-bank analysis: seedling emergence after removing the soil by sieving. Funct Ecol. 10(1):144–151.
- Touzard B, Amiaud B, Langlois E, Lemauviel S, Clement B. 2002. The relationships between soil seed bank, aboveground vegetation and disturbances in a eutrophic alluvial wetland of Western France. Flora. 197(3):175–185.
- [USACE] United States Army Corps of Engineers. 1973. Permits for activities in navigable waters or ocean waters. Fed Regist. 38:12217–12230.
- [USEPA] United States Environmental Protection Agency. 1980. Guidelines for specification of disposal sites for dredged or fill material. Fed Regist. 45:85336–85357.
- Weiher E, Wisheu IC, Keddy PA, Moore DRJ. 1996. Establishment, persistence, and management implications of experimental wetland plant communities. Wetlands. 16(2):208–218.
- Wentworth TR, Johnson GP, Kologiski RL. 1988. Designation of wetlands by weighted averages of vegetation data: a preliminary evaluation. Water Resour Bull. 24(2):389–396.
- Zedler JB. 2000. Progress in wetland restoration ecology. Trends Ecol Evol. 15(10):402–407.