Abstract
Palmetto bass (female striped bass Morone saxatilis × male white bass Morone chrysops) were stocked into Long Branch Lake, Missouri, USA in an effort to restructure the gizzard shad Dorosoma cepedianum population via predation. The goal of this restructuring was to provide more suitable-size prey for white crappie Pomoxis annularis, thereby improving growth of this important sport fish. Secondarily, palmetto bass may prey on the abundant white crappie population which may reduce density-dependent effects on growth. Trends in abundance and mean total length (TL) at age of white crappie and gizzard shad were examined over an eight-year period which spanned years before, during, and after stocking of palmetto bass. Also, trends in water quality and zooplankton variables, which may influence gizzard shad demographics, were examined during this period. Following stockings of palmetto bass, mean TL at age of adults and abundance of age-0 gizzard shad increased. Water quality variables and zooplankton biomass that may have influenced gizzard shad showed no temporal trends except for turbidity which increased over time. Despite the changes in the gizzard shad population, mean TL at age of white crappie did not improve and their abundance did not decline. Age-0 gizzard shad still quickly outgrew their vulnerability to most white crappie. It may be necessary to incorporate other management strategies in addition to stocking palmetto bass to improve growth of white crappie in this reservoir.
Introduction
Adequate abundance of suitable-size prey fish is necessary to support optimal growth and condition of piscivorous sport fishes. Prey fish shortages may occur for a variety of reasons including inadequate water fertility (Ney Citation1996; Stockner et al. Citation2000), mismatches in temporal or spatial overlap between predators and prey (Rose & Leggett Citation1989; Cyterski & Ney Citation2005), excessive habitat refugia for prey (Dibble et al. Citation1996; Sammons & Maceina Citation2006), and prey defense mechanisms (Moody et al. Citation1983; Savino & Stein Citation1989; Pettersson et al. Citation2000). In some cases, prey can be abundant but are not available to predators because of their large size (Hambright et al. Citation1991; Nilsson & Brönmark Citation2000). This is a common occurrence in Midwestern and southern US reservoirs where the major prey species, the gizzard shad Dorosoma cepedianum, frequently attains large biomass with few individuals vulnerable to piscivores (Noble Citation1981; Hambright et al. Citation1991).
In an effort to provide more suitable-size prey for piscivores, management strategies have been directed toward reducing adult gizzard shad abundance, with the anticipation that production of small-bodied young would increase. Reservoirs with a high biomass of adults commonly have poor recruitment of young gizzard shad (Smith Citation1959; Ostrand et al. Citation2002). By reducing adult abundance, production of young has increased in some waters (Kim & DeVries Citation2000; Schramm et al. Citation2000; Ostrand et al. Citation2002). Reducing gizzard shad abundance by using fish toxicants has been commonly used for this purpose with some success (reviewed by DeVries & Stein Citation1990; Kim & DeVries Citation2000). However, these successes are often short-lived and frequent treatments are required to maintain desirable conditions (Zeller & Wyatt Citation1967; Kim & DeVries Citation2000). Large piscivores capable of preying on large gizzard shad have also been introduced to reduce gizzard shad abundance. In particular, striped bass Morone saxatilis and their hybrids (striped bass × white bass Morone chrysops) have been used to restructure gizzard shad populations (review by Dettmers et al. Citation1996; Schramm et al. Citation2000; Ostrand et al. Citation2002). Some of these stockings have been successful in reducing adult abundance and increasing abundance of young gizzard shad (e.g., Harper & Namminga Citation1986; Schramm et al. Citation2000; Ostrand et al. Citation2002).
Restructuring gizzard shad populations through stockings of Morone spp. has led to improvements in growth and size structure of piscivorous sport fishes. Jahn et al. (Citation1987) and Schramm et al. (Citation2000) found improvements in the growth and size structure of white crappie Pomoxis annularis after stockings of these piscivores. Ostrand et al. (Citation2002) noted improvements in the abundance and size of black crappies Pomoxis nigromaculatus and largemouth bass Micropterus salmoides following the restructuring of the gizzard shad population via hybrid Morone stockings. Thus, introductions of Morone spp. have the potential to improve sport fish populations through changes in gizzard shad population size structure.
In Long Branch Lake, Missouri, age-0 gizzard shad, the dominant prey species, outgrow their vulnerability to white crappie predation every year and, thus, white crappies consistently grow slowly in this reservoir (Michaletz et al. Citation2012; Michaletz Citation2013). Palmetto bass (female striped bass Morone saxatilis × male white bass M. chrysops) were stocked into Long Branch Lake in an attempt to restructure the gizzard shad population. Fast-growing young gizzard shad indicates that their densities are relatively low because their growth is often density dependent (Garvey & Stein Citation1998; Dettmers & Wahl Citation1999; Michaletz Citation1999). By reducing adult gizzard shad abundance, adults may respond with greater reproductive success. More abundant and smaller age-0 gizzard shad could provide prey for white crappie and thereby improve growth rates of this sport fish. Also, if palmetto bass can keep adult gizzard shad abundance at moderate levels, growth of adult gizzard shad could increase, leading to a wider size range of adults that could result in a more prolonged spawning season and a wider size range of young (Miranda & Muncy Citation1988). Secondarily, white crappies are very abundant in Long Branch Lake and growth of these fish may improve if intraspecific competition can be reduced. Although Morone hybrids prefer clupeids as prey (Jahn et al. Citation1987; Ebert et al. Citation1988), they will feed on crappies as well (Germann Citation1983; Neal et al. Citation1999) and may improve crappie size structure through reductions in crappie abundance (Jahn et al. Citation1987; Neal et al. Citation1999).
The objective for this study was to determine if stockings of palmetto bass could lead to improved growth of white crappie via restructuring of the gizzard shad population. Palmetto bass were sampled annually to determine population demographics and diets and trends in mean total length (TL) at age and abundance of gizzard shad and white crappie were examined over an eight-year period. Diets of white crappie were also quantified to ascertain if these piscivores consumed more gizzard shad over time. Although similar studies have been conducted (e.g., Schramm et al. Citation2000; Ostrand et al. Citation2002), few, if any, studies have statistically assessed changes in mean TL at age and abundance of gizzard shad and sport fishes (Dettmers et al. Citation1996). Additionally, other variables that may have influenced the gizzard shad population independently of palmetto bass were not examined in previous studies. For example, gizzard shad growth rate may increase over time owing to temporal increases in food abundance (i.e., zooplankton, phytoplankton, detritus; Yako et al. Citation1996; Schaus et al. Citation2002; Kim et al. Citation2007). Herein, trends in water quality and zooplankton variables that could influence gizzard shad populations were also analyzed. This study provides information useful to future attempts to improve sport fish populations via restructuring of gizzard shad populations.
Methods
Study area
This study was conducted in Long Branch Lake (surface area = 980 ha) located in north-central Missouri, USA. Long Branch Lake is a shallow (mean depth = 4.4 m), frequently turbid, eutrophic reservoir that contains a stunted white crappie population, a largemouth bass population with sparse recruitment, and a gizzard shad population with fast-growing age-0 individuals (Michaletz Citation2012, Citation2013; Michaletz et al. Citation2012; Missouri Department of Conservation, unpublished data). Most crappies (both black crappie and white crappie) never reach sizes desirable to anglers. Because of undesirable crappie populations and the low abundance of largemouth bass, sport fish angling effort was very low in Long Branch Lake.
Stockings
Small fingerling (mean TL = 47–60 mm) palmetto bass were stocked annually from 2005 to 2011 at a rate of about 128 fish/ha during late May – early July. A major exception occurred in 2007 when small fingerlings were stocked at 255 fish/ha and advanced fingerlings (mean TL = 130 mm) were stocked at 11 fish/ha in September due to a surplus of hatchery-reared fish. With the exception of these advanced fingerlings, which were stocked off a boat ramp, most fingerlings were distributed in offshore areas by boat. Stocking was avoided in the lower one-third of the reservoir to reduce the likelihood of fingerlings exiting the reservoir through the water-release structure, as palmetto bass are known to move out of reservoirs during periods of water releases (Jahn et al. Citation1987).
Fish collections
Palmetto bass were sampled in mid-October, 2006–2011, with experimental monofilament gill nets set on the bottom in water depths of 3–8 m on flats and points (Colvin Citation2002). Gill nets were set either parallel or perpendicular to shore depending on topography (i.e., steep slopes were avoided). In 2006–2008, nets were 1.8 m deep × 38 m long and consisted of 7.6-m panels of 19-, 25-, 38-, 51-, and 64-mm bar mesh. In 2009–2011, three larger mesh (74-, 89-, and 102-mm bar mesh) panels were added to potentially capture larger fish. Gill nets were set at 6 fixed sites in 2006, 21 sites in 2007, and 40 sites in 2008–2011. One gill net was set per site during the day and retrieved about 24 h later. Up to 10 randomly selected sites (from the potential list of fixed sites) were sampled each day. Each site was fished for only one day. All fish were removed from the nets, identified to species, counted, and processed separately by mesh size. Catch-per-unit-effort (CPUE) was expressed as the number of fish caught per gill-net night. Palmetto bass were measured for TL (nearest mm), and scale samples were removed from most fish. Sagittal otoliths were removed from large, dead palmetto bass in 2011 to corroborate scale ages. During 2007–2011, diet samples were removed from a subset of the palmetto bass by inserting a plexiglass tube into the stomach and flushing contents out using water from a squirt bottle. In some cases, a forceps was also used to assist in removing large prey items. Instead of removing stomach contents with tubes, whole fish were retained for diet analysis for age-0 palmetto bass.
Annual mean densities (N/1000 m3) of age-0 and older gizzard shad and crappies were estimated from monthly trawl collections during July–October or early November. Paired 1-m2 frame trawls equipped with 6-mm mesh were towed at night in a stair-stepped fashion from the surface to 1–2 m off bottom at six fixed sites in upper and lower areas (12 sites total) of Long Branch Lake (Michaletz et al. Citation2012). Trawls were towed at 1.6 m/sec and flowmeters attached to the trawl frames were used to determine the volume of water filtered by each tow. Age-0 crappies and gizzard shad were retained for later identification, counting, and measuring. All age-1 and older fish were identified, counted, and released. Age-1 and older crappies collected by trawls were not consistently separated by species but white crappie comprised more than 90% of the two species combined when species were identified. Thus, these density estimates were considered to reflect abundance of white crappie even though a small portion of black crappie was included in these estimates.
Because ages were not estimated for age-1 and older fish captured by trawling, gizzard shad collected by nighttime electrofishing in May were used to estimate mean TL at age. Gizzard shad were collected during six electrofishing transects, usually 20 minutes in duration (Michaletz Citation2012). All gizzard shad were counted and measured for each transect and sagittal otoliths were removed for aging from a subsample (10 fish per 10-mm TL class) of these fish. However, first year growth of gizzard shad was estimated from the mean TL of age-0 gizzard shad captured during late October or early November trawling instead of mean TL of age-1 fish captured by electrofishing in the following spring. Mean TL of age-0 gizzard shad in the fall of 2003 was acquired from Michaletz (Citation2010). This method provided a more accurate estimate of first year growth than that estimated from spring electrofishing owing to size-selective and extensive overwinter mortality (Michaletz Citation2010), which sometimes resulted in very low spring sample sizes for age-1 gizzard shad.
White crappies collected by autumn trap netting were used to estimate mean TL at age. Missouri-style trap nets (Colvin & Vasey Citation1986) were fished for one night at eight sites and white crappies collected in these nets were counted and measured. Sagittal otoliths were removed from 35 fish for each small (<150 mm TL), medium (150–229 mm TL), and large (≥230 mm TL) size group of fish (Michaletz Citation2013).
Age and growth
Ages of palmetto bass were estimated from scales and whole otoliths and ages of gizzard shad and white crappie were estimated from sectioned otoliths (Michaletz Citation2012, Citation2013). Annuli were counted on projected images of these bony structures. Mean TL at age was determined by applying age–length keys developed from aged fish to the entire sample for each species (Isermann & Knight Citation2005).
Diets
Prey items found in stomach samples of palmetto bass were identified as zooplankton, macroinvertebrates, or fish (to species level, if possible). Prey items were pooled by each food category and weighed (nearest 0.001 g) in aggregate except that prey fish were weighed individually when possible. Percentage of each food category by weight was determined for each predator and mean percent weight of each food category was computed by averaging across all predators for each predator length group and year. Palmetto bass were divided into two length groups, <220 mm and ≥220 mm TL, which usually separated age-0 from older ages.
Diets of small (<150 mm TL), medium (150–229 mm TL), and large (≥230 mm TL) white crappie were determined from monthly trap net collections during May–October or early November, 2004–2011 (Michaletz et al. Citation2012). When available, 35 fish of each size group were retained for diet analysis. Mean percent weight of each food category was determined in the same manner as for palmetto bass for each size group and month. Mean percent wet weight of gizzard shad in the diets for each month was then averaged across all months for each year to produce a single annual estimate for each white crappie size group.
Environmental variables
Water quality and zooplankton data were collected in each of the two areas (upper and lower) during 2004–2011 in Long Branch Lake. Chlorophyll a, turbidity, Secchi depth, and zooplankton biomass were measured at two fixed sites per area weekly from late April to late June or early July and then once every two weeks through October or early November. Water temperature and dissolved oxygen profiles, total phosphorus, and total nitrogen were measured at one fixed site per area on the same dates. Water quality and zooplankton biomass data were collected, processed, and analyzed following procedures in Michaletz (Citation2012, Citation2014). Estimates of all variables were averaged across all sites and sampling dates for each year to provide a single annual estimate.
Data analysis
Kendall tau-b correlations (Snedecor & Cochran Citation1967) were used to detect temporal trends in annual estimates of abundance and mean TL at age of gizzard shad and white crappie, the proportion of gizzard shad in the diets of white crappie, water quality, and zooplankton biomass. Surface water temperature was used as the temperature variable. Trends in dissolved oxygen were not assessed because sufficient oxygen (>3 mg/L) was always available. Kendall tau-b is a nonparametric test which estimates the correlation coefficient for ranks between the variable of interest and time (Maceina & Pereira Citation2007). Positive coefficients indicate an increasing trend in the variable of interest, whereas negative coefficients indicate a decreasing trend. This test is appropriate for analyzing trend data with negligible autocorrelation and has been useful in analyzing trends in fisheries data (Maceina & Pereira Citation2007). Durbin–Watson tests (Manly Citation2001) were used to determine if trend data exhibited autocorrelation. Due to the relatively low sample size (n ≤ 8 years) for trend analyses, these tests were considered significant at p ≤ 0.10.
Results
Palmetto bass population characteristics and diets
Palmetto bass CPUE was highly variable among gill nets, with 43% of the sets capturing no palmetto bass and some capturing 20 fish or more. Gill net CPUE ranged from a low of 1.3 fish/net in 2007 to a high of 5.6 fish/net in 2009 (). Age-0 palmetto bass were not collected every year but members from every year class were sampled over the study period (). The 2009 stocking and, to a lesser degree, the 2007 stocking produced strong year classes (). With successive annual stockings, the length range of palmetto bass widened, and by 2009 some fish exceeded 600 mm TL ().
Figure 1. Mean (± SE) catch per unit effort (N/gill-net night) for palmetto bass in Long Branch Lake, 2006–2011. Numbers above error bars indicate the number of gill net sets.
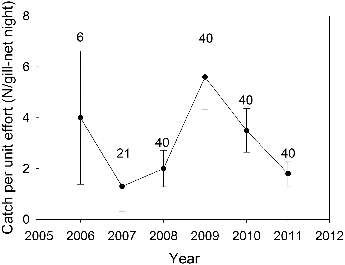
Diets of palmetto bass consisted mostly of gizzard shad and other fishes (). Small palmetto bass (<220 mm TL) consumed more invertebrates than larger fish (≥220 mm TL). Gizzard shad was the most common fish consumed by palmetto bass but other fishes, including palmetto bass, sunfish Lepomis spp., and white crappies, were consumed and sometimes important in the diets.
Table 1. Mean percent weight of prey items ingested by palmetto bass in Long Branch Lake, 2007–2011. Palmetto bass were split into two total length (TL) categories: <220 mm TL and ≥220 mm TL. Abbreviations for prey items are gizzard shad (Giz), palmetto bass (Pal), sunfish (Sun), white crappie (Whc), other fish (Oth), unidentified fish (Uni), all fish combined (Fish), and invertebrates (Inv). N = sample size.
Temporal changes in gizzard shad and white crappie
Temporal changes in abundance and growth were evident for gizzard shad but not for white crappie. The abundance of age-0 and mean TL for age-3 and older gizzard shad increased over time (, ). The trends test was not significant (p = 0.105) for density of age-1 and older gizzard shad but their densities were consistently low after 2007 (). There were no significant trends in mean TL for age-0 (estimated from fall trawl catches) or age-2 gizzard shad.
Table 2. Results of trend analysis of density (N/1000 m3) and mean total length at age (TL, mm) of gizzard shad and white crappie in Long Branch Lake, 2004–2011, using Kendall tau-b correlations (τ). Mean TL at age were estimated from fall samples for white crappie and from spring samples for gizzard shad, except age-0 gizzard shad captured in the fall were used in lieu of the following spring age-1 fish. Values for τ range from −1 to +1 with positive values indicating an increasing trend and negative values indicating a decreasing trend. n = years. An asterisk indicates significant positive autocorrelation (Durbin-Watson test, p ≤ 0.10).
Figure 4. Mean July–October or early November density (N/1000 m3) of age-0 (filled circle) and age-1 and older (open circle) and mean total length (TL) at age for gizzard shad and white crappie in Long Branch Lake, 2004–2011. Error bars are SE. Mean TL at age was estimated from white crappie collected in the fall and gizzard shad collected in the spring except that fall age-0 gizzard shad were used to estimate first year growth in lieu of age-1 fish collected in the spring. Sample sizes for density estimates are 48 trawl tows for 2005–2009 and 2011 and 60 trawl tows for 2004 and 2010. Sample sizes averaged 280 fish (range, 80–409) for age-0 gizzard shad, 155 fish (range, 3–695) per age class for age 1 and older gizzard shad, 89 fish (range, 37–256) for age-0 white crappie, and 63 fish (range, 5–344)) per age class for age 1 and older white crappie (based on length–age keys applied to the entire sample).
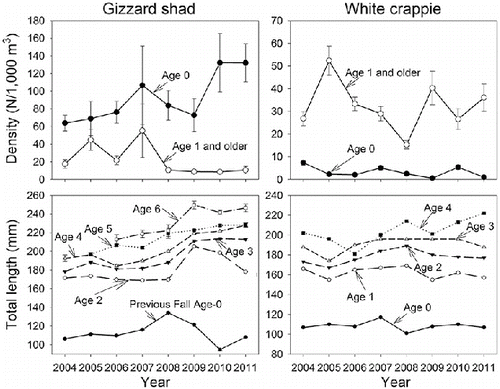
In contrast to gizzard shad, there were no significant trends in abundance of age-0 or older crappies (, ). There was a positive trend in mean TL for age-4 white crappie but no other trends in mean TL were significant for other ages. The mean percent wet weight of gizzard shad in the diets of small (τ = 0.00, p = 1.00), medium (τ = −0.14, p = 0.62), and large (τ = 0.07, p = 0.80) white crappie also did not exhibit significant temporal trends ().
Environmental variables
No temporal trends were evident for most water quality and zooplankton variables (). Only the analysis for turbidity was significant and it indicated that turbidity increased over the study period. All other variables showed no significant trends.
Table 3. Median, minimum (Min), and maximum (Max) values and results of trend analysis of water quality and zooplankton in Long Branch Lake, 2004–2011, using Kendall tau-b correlations (τ). Values for τ range from −1 to +1 with positive values indicating an increasing trend and negative values indicating a decreasing trend. An asterisk indicates significant positive autocorrelation (Durbin–Watson test, n = 8 years, p ≤ 0.10).
Discussion
A population of palmetto bass was developed in Long Branch Lake and appeared to influence gizzard shad demographics but had little effect on white crappie. Although the trends test for density of age-1 and older gizzard shad was not significant, the concurrent increases in mean TL of older gizzard shad support the notion that their abundance declined. Densities of age-1 and older gizzard shad were consistently low after 2007 compared to previous years. Temporal increases in mean TL for age-3 and older gizzard shad were clearly evident (). There was evidence for positive autocorrelation for these tests (), but that does not negate the validity of these trends (Maceina & Pereira Citation2007). Declines in abundance of age-1 and older gizzard shad and concurrent increases in their growth rates are similar to findings of other studies (Morris & Follis Citation1979; Harper & Namminga Citation1986; Schramm et al. Citation2000; Ostrand et al. Citation2002). Reductions in age-1 and older gizzard shad were concomitant with increases in age-0 abundance. Gizzard shad are known to exhibit density-dependent growth (DiCenzo et al. Citation1996; Michaletz Citation1999) and reductions in adult gizzard shad abundance have led to higher reproductive success (Morris & Follis Citation1979; Harper & Namminga Citation1986; Kim & DeVries Citation2000; Schramm et al. Citation2000), which is congruent with these results.
Study results support the hypothesis that predation by palmetto bass reduced gizzard shad abundance leading to these changes in gizzard shad demographics. Other variables that may have influenced gizzard shad, such as water quality and zooplankton biomass, exhibited no trends over the study period with the exception of an increase in turbidity. Turbidity generally increased over the study period because of more precipitation in the latter years which led to greater influxes of suspended solids into the reservoir. Conceivably, growth of detritivorous gizzard shad could have increased in response to these greater inputs of detrital material (Pilati et al. Citation2009). However, growth of gizzard shad feeding on detrital material depends upon the energy content of this food source (Schaus et al. Citation2002; Kim et al. Citation2007). Growth of gizzard shad is only enhanced when detrital material is composed of greater amounts of organic material (Kim et al. Citation2007), which was a small portion of the total suspended solids in years with high turbidity (unpublished data, Limnology Laboratory, University of Missouri). Mean TL of adult gizzard shad was increasing and their abundance decreasing prior to years when turbidity was higher. Thus, increases in turbidity alone cannot explain the changes in growth rates nor the changes in densities of both age-0 and older gizzard shad.
Some studies reported an improvement in growth rates of crappies as a result of Morone hybrid stockings (Jahn et al. Citation1987; Neal et al. Citation1999). Unfortunately, this was not the case in Long Branch Lake. Despite higher densities of age-0 gizzard shad during the last years of the study, their growth rates were still relatively fast, and these prey quickly reached sizes invulnerable to predation by most white crappie (Michaletz et al. Citation2012). For example, age-0 gizzard shad were no longer vulnerable to predation by age-3 white crappie by early September 2009, the year when age-0 gizzard shad were smallest in fall (P.H. Michaletz, unpublished data). Consequently, the proportion of gizzard shad in diets of white crappie did not increase over time and growth rates of white crappie did not improve (except for fall mean TL at age 4). Higher turbidity in the latter years of the study may have also influenced foraging success of white crappie. High turbidity has been shown to influence foraging of piscivores (Reid et al. Citation1999; De Robertis et al. Citation2003; Shoup & Wahl Citation2009) and may have further reduced the ability of white crappie to prey on gizzard shad. White crappies are very abundant in this reservoir as well. Although palmetto bass did consume some white crappie, this predation was not sufficient to cause significant reductions in white crappie abundance. Probably in response to chronic slow growth, white crappie in Long Branch Lake matured at smaller sizes and younger ages than in other northern Missouri reservoirs, thus diverting energy into gonad production at the expense of somatic growth (Michaletz Citation2013). Early maturation further hampers attempts to improve growth. Modifying land-use practices in the watershed to reduce nutrient and sediment inputs into the reservoir, in conjunction with piscivore stockings, may be necessary to effectively manage gizzard shad populations in this highly eutrophic reservoir (Vanni et al. Citation2005), which may potentially lead to improvements in growth of white crappie.
Acknowledgements
I thank Dean Nicks, Earl Buckner, Rachel Wright, and Steffanie Abel for conducting much of the field and laboratory work. Numerous other individuals assisted with various aspects of this study. I thank the Missouri Department of Conservation's hatchery staff who reared and stocked the palmetto bass. I also thank Mike Colvin who was co-investigator on this study prior to his retirement.
Additional information
Funding
References
- Colvin MA. 2002. A comparison of gill netting and electrofishing as sampling techniques for white bass in Missouri's large reservoirs. North Am J Fish Manag. 22:690–702.
- Colvin MA, Vasey FW. 1986. A method of qualitatively assessing white crappie populations in Missouri reservoirs. In: Hall GE, Van Den Avyle MJ, editors. Reservoir fisheries management: strategies for the 80's. Bethesda (MD): Reservoir Committee, Southern Division, American Fisheries Society. p. 79–85.
- Cyterski MJ, Ney JJ. 2005. Availability of clupeid prey to primary piscivores in Smith Mountain Lake, Virginia. Trans Am Fish Soc. 134:1410–1421.
- De Robertis A, Ryer CH, Veloza A, Brodeur RD. 2003. Differential effects of turbidity on prey consumption of piscivorous and planktivorous fish. Can J Fish Aquatic Sci. 60:1517–1526.
- Dettmers JM, DeVries DR, Stein RA. 1996. Quantifying responses to hybrid striped bass predation across multiple trophic levels: implications for reservoir biomanipulation. Trans Am Fish Soc. 125:491–504.
- Dettmers JM, Wahl DH. 1999. Evidence for zooplankton compensation and reduced fish growth in response to increased juvenile fish density. Hydrobiologia 400:115–121.
- DeVries DR, Stein RA. 1990. Manipulating shad to enhance sport fisheries in North America: an assessment. North Am J Fish Manag. 10:209–223.
- Dibble ED, Killgore KJ, Harrel SL. 1996. Assessment of fish-plant interactions. In: Miranda LE, DeVries DR, editors. Multidimensional approaches to reservoir fisheries management. Bethesda (MD): American Fisheries Society Symposium 16; p. 357–372.
- DiCenzo VJ, Maceina MJ, Stimpert MR. 1996. Relations between reservoir trophic state and gizzard shad population characteristics in Alabama reservoirs. North Am J Fish Manag. 16:888–895.
- Ebert DJ, Shirley KE, Farwick JJ. 1988. Evaluation of Morone hybrids in a small, shallow, warmwater impoundment. Proc Annu Conf Southeast Assoc Fish Wildl Agencies. 41:55–62.
- Garvey JE, Stein RA. 1998. Competition between larval fishes in reservoirs: the role of relative timing of appearance. Trans Am Fish Soc. 127:1021–1039.
- Germann JF. 1983. Food habits of Morone hybrid bass in Clarks Hill Reservoir, Georgia. Proc Annu Conf Southeast Assoc Fish Wildl Agencies. 36:53–61.
- Hambright KD, Drenner RW, McComas SR, Hairston Jr. NG. 1991. Gape-limited piscivores, planktivore size refuges, and the trophic cascade hypothesis. Archiv für Hydrobiologie. 121:389–404.
- Harper JL, Namminga HE. 1986. Fish population trends in Texoma Reservoir following establishment of striped bass. In: Hall GE, Van Den Avyle MJ, editors. Reservoir fisheries management: strategies for the 80's. Bethesda (MD): Reservoir Committee, Southern Division, American Fisheries Society; p. 156–165.
- Isermann DA, Knight CT. 2005. A computer program for age-length keys incorporating age assignment to individual fish. North Am J Fish Manag. 25:1153–1160.
- Jahn LA, Douglas DR, Terhaar MJ, Kruse GW. 1987. Effects of stocking hybrid striped bass in Spring Lake, Illinois. North Am J Fish Manag. 7:522–530.
- Kim GW, DeVries DR. 2000. Effects of a selectively reduced gizzard shad population on trophic interactions and age-0 fishes in Walker County Lake, Alabama. North Am J Fish Manag. 20:860–872.
- Kim GW, Wintzer AP, Menker TK, Stein RA, Dettmers JM, Wright RA, DeVries DR. 2007. Effects of detritus quality on growth and survival of gizzard shad (Dorosoma cepedianum): potential importance to benthic-pelagic coupling. Can J Fish Aquatic Sci. 64:1805–1815.
- Maceina MJ, Pereira DL. 2007. Recruitment. In: Guy CS, Brown ML, editors. Analysis and interpretation of freshwater fisheries data. Bethesda (MD): American Fisheries Society; p. 121–185.
- Manly BFJ. 2001. Statistics for environmental science and management. Boca Raton (FL): Chapman and Hall/CRC.
- Michaletz PH. 1999. Influence of reservoir productivity and juvenile density on first-year growth of gizzard shad. North Am J Fish Manag. 19:842–847.
- Michaletz PH. 2010. Overwinter survival of age-0 gizzard shad in Missouri reservoirs spanning a productivity gradient: roles of body size and winter severity. Trans Am Fish Soc. 139:241–256.
- Michaletz PH. 2012. Gizzard shad population dynamics in eutrophic Missouri reservoirs with emphasis on environmental influences on their growth. J Freshwater Ecol. 27:185–197.
- Michaletz PH. 2013. Contrasting population demographics and reproductive investment of white crappie among three eutrophic reservoirs. Fisheries Manage Ecol. 20:1–9.
- Michaletz PH. 2014. Temperature, plankton and conspecific density influence dynamics of age-0 gizzard shad: implications for a gape-limited piscivore. Ecol Freshwater Fish. 23:322–335.
- Michaletz PH, Bajer PG, Hayward RS. 2012. Seasonal patterns in white crappies’ consumption and growth: influences of varying water temperatures and prey availability. Trans Am Fish Soc. 141:681–696.
- Miranda LE, Muncy RJ. 1988. Spawning sequence of largemouth bass, bluegill, and gizzard shad. Proc Annu Conf Southeast Assoc Fish Wildl Agencies. 41:197–204.
- Moody RC, Helland JM, Stein RA. 1983. Escape tactics used by bluegills and fathead minnows to avoid predation by tiger muskellunge. Environ Biol Fishes. 8:61–65.
- Morris DJ, Follis BJ. 1979. Effects of striped bass predation upon shad in Lake E. V. Spence, Texas. Proc Annu Conf Southeast Assoc Fish Wildl Agencies. 32:697–702.
- Neal JW, Noble RL, Rice JA. 1999. Fish community response to hybrid striped bass introduction in small warmwater impoundments. North Am J Fish Manag. 19:1044–1053.
- Ney JJ. 1996. Oligotrophication and its discontents: effects of reduced nutrient loading on reservoir fisheries. In: Miranda LE, DeVries DR, editors. Multidimensional approaches to reservoir fisheries management. Bethesda (MD): American Fisheries Society Symposium 16; p. 285–295.
- Nilsson PA, Brönmark C. 2000. Prey vulnerability to a gape-size limited predator: behavioural and morphological impacts on northern pike piscivory. Oikos. 88:539–546.
- Noble RL. 1981. Management of forage fishes in impoundments of the southern United States. Trans Am Fish Soc. 110:738–750.
- Ostrand KG, Schramm Jr. HL, Kraai JE, Braeutigam B. 2002. Effects of intensive stocking of hybrid striped bass on the population structure of gizzard shad in a west Texas impoundment, a case study. Proc Annu Conf Southeast Assoc Fish Wildl Agencies. 55:324–333.
- Pettersson LB, Nilsson PA, Brönmark C. 2000. Predator recognition and defence strategies in crucian carp, Carassius carassius. Oikos 88:200–212.
- Pilati A, Vanni MJ, González MJ, Gaulke AK. 2009. Effects of agricultural subsidies of nutrients and detritus on fish and plankton of shallow-reservoir ecosystems. Ecological Appl. 19:942–960.
- Reid SM, Fox MG, Whillans TH. 1999. Influence of turbidity on piscivory in largemouth bass (Micropterus salmoides). Can J Fish Aquatic Sci. 56:1362–1369.
- Rose GA, Leggett WC. 1989. Interactive effects of geophysically-forced sea temperature and prey abundance on mesoscale coastal distributions of a marine predator, Atlantic cod (Gadus morhua). Can J Fish Aquatic Sci. 46:1904–1913.
- Sammons SM, Maceina MJ. 2006. Changes in diet and food consumption of largemouth bass following large-scale hydrilla reduction in Lake Seminole, Georgia. Hydrobiologia. 560:109–120.
- Savino JF, Stein RA. 1989. Behavioural interactions between fish predators and their prey: effects of plant density. Anim Behav. 37:311–321.
- Schaus MH, Vanni MJ, Wissing TE. 2002. Biomass-dependent diet shifts in omnivorous gizzard shad: implications for growth, food web, and ecosystem effects. Trans Am Fish Soc. 131:40–54.
- Schramm Jr. HL, Kraai JE, Munger CR. 2000. Intensive stocking of striped bass to restructure a gizzard shad population in a eutrophic Texas reservoir. Proc Annu Conf Southeast Assoc Fish Wildl Agencies. 53:180–192.
- Shoup DE, Wahl DH. 2009. The effects of turbidity on prey selection by piscivorous largemouth bass. Trans Am Fish Soc. 138:1018–1027.
- Smith Jr. WA. 1959. Shad management in reservoirs. Proc Annu Conf Southeast Assoc Game Fish Commissioners. 12:143–147.
- Snedecor GW, Cochran WG. 1967. Statistical methods. 6th ed. Ames (IA): Iowa State University Press.
- Stockner JG, Rydin E, Hyenstrand P. 2000. Cultural oligotrophication: causes and consequences for fisheries resources. Fisheries. 25:7–14.
- Vanni MJ, Arend KK, Bremigan MT, Bunnell DB, Garvey JE, González MJ, Renwick WH, Soranno PA, Stein RA. 2005. Linking landscape and food webs: effects of omnivorous fish and watersheds on reservoir ecosystems. Biosci. 55:155–167.
- Yako LA, Dettmers JM, Stein RA. 1996. Feeding preferences of omnivorous gizzard shad as influenced by fish size and zooplankton density. Trans Am Fish Soc. 125:753–759.
- Zeller HD, Wyatt HN. 1967. Selective shad removal in Southern reservoirs. In: Reservoir Committee, editors. Reservoir Fishery Resources Symposium. Bethesda (MD): American Fisheries Society, Southern Division, Reservoir Committee; p. 405–414.