Abstract
Surface water samples of Lake Taihu and its tributaries were collected from September 2012 to February 2013 to investigate the effects of dissolved organic carbon (DOC) dynamics on bacterial abundance. DOC concentrations, stable carbon isotope values (δ13CDOC), dissolved carbohydrates, and specific ultraviolet absorbance (SUVA254) were analyzed along with several environmental factors: water temperature, electrical conductivity (EC), chlorophyll a, and bacterial abundance. The highest DOC concentrations were observed in January 2013 at all sampling sites except at lake center. DOC concentrations decreased along both transects from the tributaries to the lake center in January 2013. In addition, the evidence from the positive correlation between DOC and EC in Lake Taihu suggests the input of river water into the lake. The variation in DOC concentration can be explained by temperature-dependent bacterial utilization of organic compounds. The δ13CDOC values ranged from −26.9‰ to −23.4‰ in Lake Taihu and its tributaries, suggesting an influence of autochthonous sources and microbial utilization. There was no significant relationship between the ratio of total dissolved carbohydrates to dissolved organic carbon (TCHO/DOC) and bacterial abundance, indicating a complex bacterial metabolic effect on carbohydrates. The spectroscopic character of DOC shifted toward less humic material from autumn to winter and correlated positively with bacterial abundance, suggesting that humic substances might serve as a substrate for bacteria in Lake Taihu and its tributaries. These results demonstrate that DOC may originate from autochthonous and allochthonous sources and that the quantity and quality of DOC changes considerably during autumn and winter, with important effects on bacterial abundance.
Introduction
Since the introduction of the microbial loop concept (Azam et al. Citation1983), heterotrophic bacteria have been recognized as a significant component of aquatic food webs, channeling energy from dissolved organic carbon (DOC) to higher trophic levels. The dynamics of DOC and its association with phytoplankton and bacteria have been intensively studied in ocean, estuary, coastal, and shelf regions (Raymond & Bauer Citation2000; Trabelsi & Rassoulzadegan Citation2011; Lasternas & Agustí Citation2013). A recent study by Kawasaki et al. (Citation2013) reported high bacterial activity in Lake Kasumigaura and suggested that bacteria play more important roles in the carbon cycles of freshwater environments than in those of open oceans.
The two DOC sources in lake ecosystems are autochthonous DOC produced from in situ autotrophic and heterotrophic activities and allochthonous inputs draining from the catchment (Kritzberg et al. Citation2004; Guillemette & del Giorgio Citation2012). The natural stable carbon isotope ratio of DOC (δ13CDOC) can be used to identify the contribution of DOC sources to aquatic systems (Ziegler & Brisco Citation2004; Kaldy et al. Citation2005). For example, the δ13CDOC (−28.5‰) observed in a reservoir during an algal bloom was indistinguishable from that (−28.6‰) of allochthonous sources (Pierson-Wickmann et al. Citation2011). It has been reported that phytoplankton blooms contribute to the autochthonous DOC, which contains more carbohydrates and is highly bioreactive in the carbon cycle (Søndergaard & Middelboe Citation1995; Meon & Kirchman Citation2001). Chrost et al. (Citation1989) observed that the highest concentration of total dissolved carbohydrates (TCHO) occurred shortly after the peak of phytoplankton biomass. Furthermore, a significant negative correlation between bacterial abundance (BA) and the ratio of total dissolved carbohydrates to dissolved organic carbon (TCHO/DOC) was observed in the Mandovi estuary (Khodse et al. Citation2010). Allochthonous DOC is composed extensively of humic substances, which can be measured by specific ultraviolet absorbance (SUVA254); therefore, it has been considered recalcitrant (Moran & Hodson Citation1990). However, recent studies have reported that these humic materials can be used as bacterial energy sources (Bergström & Jansson Citation2000; Burkowska & Donderski Citation2007). For example, a positive correlation between bacterial production and SUVA254 was observed in the lower Mississippi and Pearl rivers (Duan et al. Citation2007).
Lake Taihu is a large, shallow eutrophic lake with a complex river and channel network. There are 172 rivers or channels that connect to the lake and most of the polluted in-flowing rivers are in the west or northwest (Xu & Qin Citation2005). The DOC of Lake Taihu and its tributaries has drawn much attention. Zhang et al. (Citation2011) suggested that the contribution of allochthonous CDOM (chromophoric dissolved organic matter) decreased in the dry season due to low discharge. It was also shown that DOC quality and quantity are affected by the phytoplankton bloom collapse in Lake Taihu in autumn and winter (Ye, Shi, Wu, & Kong Citation2011; Ye, Shi, Wu, Zhang, et al. Citation2011). Nevertheless, the relationships among DOC dynamics and sources, the chemical and spectroscopic properties of DOC, and BA and water characteristics of Lake Taihu and its tributaries in autumn and winter remains unknown. The objectives of this work were to: (1) track the changes in water environmental variables, including water temperature (WT), chlorophyll a (Chl a), electrical conductivity (EC), and BA; (2) examine the natural stable carbon isotope ratios, the chemical and spectroscopic properties of DOC with respect to δ13CDOC, the dissolved carbohydrate concentrations, and SUVA254; and (3) investigate the relationship between DOC and BA. The resulting data provide insight into the sources, composition, and bioavailability of DOC in lake and tributary ecosystems.
Methods
Site description
Lake Taihu is one of the largest freshwater lakes in China. It is located in the southeast region of the Yangtze River delta (30° 55′ 40″–31° 32′ 58″ N, 119° 52′ 32″–120° 36′ 10″ E), with an area of 2340 km2. The maximum depth of the lake is 3.3 m and the mean water residence time is approximately 300 d (Tang et al. Citation2010). The northwestern section is severely polluted. Most of the tributaries, polluted by anthropogenic inputs such as urban sewage and aquaculture, are in the west or northwest (Yao et al. Citation2011). The water quality in the southern region is comparatively good relative to the polluted northwestern regions (Zhu Citation2008). In addition, frequent phytoplankton blooms occur in the northwestern region and large amounts of DOC are released upon the lysis of phytoplankton cells (Ye, Shi, Wu, Zhang, et al. Citation2011). Finally, the northern part of the lake is the drinking water source for Wuxi City. Therefore, the pollution in the northwestern area is a main concern.
Sampling
Surface water samples were taken monthly from September 2012 to February 2013 in Lake Taihu along two transects. One transect contained five sampling sites, starting in the Yincungang River (site 1), continuing to sites 2, 3, 4, and ending in the center of the lake (site 5). The other transect started in the Chendonggang River (site 6) and continued to sites 7, 4, and 5. Site 2 is located in the mouth of Zhushan Bay, which is one of the most eutrophic bays in the northern part of the lake (Liu et al. Citation2012). Site 7 is located in the western part of Lake Taihu ().
Analytical procedures
Measurements from three replicate subsamples of each sample were recorded. WT and EC were measured with a YSITM Professional Plus water quality meter. The surface water samples were then filtered, and the filters were analyzed for Chl a. The filtrates were analyzed for DOC, dissolved carbohydrates, SUVA254, and δ13CDOC. Chl a collected on a Whatman GF/C glass filter was determined spectrophotometrically after hot methanol extraction (Pápista et al. Citation2002). For BA, 9.5 mL samples were fixed with 0.5 mL 37% formaldehyde and stored at 4 °C until analysis. A well-mixed subsample was diluted with particle-free distilled water (1:40) and stained with 4′,6′-diamidino-2-phenylindole (DAPI, Sigma, Inc.) at a final concentration of 2 μg mL−1 for 10 min (Tang et al. Citation2010). After staining, the samples were filtered onto black polycarbonate filters (0.2-μm pore size) and embedded in nonfluorescent immersion oil. The bacterial cells were enumerated using an epifluorescence microscope (Zeiss AxiovertTM 135 M) equipped with a HBO 100 W mercury lamp and a filter set (excitation: G 365, emission: BP 445/50, Zeiss filter set 49). A minimum of 20 fields of view and 400 bacterial cells were counted per sample. BA was expressed in cells mL−1 of water.
DOC, δ13CDOC, SUVA254, and the dissolved carbohydrate samples were collected by filtration using a Whatman GF/F glass filter that was precombusted at 450 °C for 5 h. DOC was determined by the high-temperature catalytic oxidation method (Zhang et al. Citation2006) using a total organic carbon analyzer (Shimadzu TOC-V CPN, Japan). The relative aromaticity of DOC was provided by the SUVA254 values, which were derived by dividing the ultraviolet absorbance measured at 254 nm by the DOC concentration and reported in L mg−1 cm−1 (Weishaar et al. Citation2003). For δ13CDOC analysis, the filtrates were acidified with 1 N HCl to remove all traces of inorganic carbon and then frozen and freeze-dried. The freeze-dried samples were combusted in a Flash EA1112 elemental analyzer coupled to a dual-inlet continuous flow isotope ratio mass spectrometer (Thermo Finnigan MAT DeltaPlus). The δ13C values were calculated as the relative deviation between the measured 13C/12C ratio (Rsample) and the 13C/12C of the international standard Vienna Pee Dee Belemnite (VPDB) (Rstandard) as follows: δ13C (‰) = [(Rsample/Rstandard) − 1] × 1000. The precision of the δ13C values reported is estimated at ±0.1‰.
The dissolved carbohydrates, including TCHO, polysaccharides (PCHO), and monosaccharides (MCHO), were determined by a spectrophotometric method, which involves oxidation of the free reduced sugars with 2,4,6-tripyridyl-s-tri-azine (TPTZ, Sigma) and subsequent spectrophotometric analysis (Myklestad et al. Citation1997). The absorbance was measured at 595 nm. The MCHO concentration of each sample was measured directly without hydrolysis. The concentration of PCHO was calculated as the difference between the TCHO and MCHO concentrations ([PCHO] = [TCHO] – [MCHO]). The concentrations of TCHO, PCHO, and MCHO are expressed as mg L−1 (Hung et al. Citation2005).
Statistical analysis
The WT, EC, Chl a, BA, DOC, δ13CDOC, PCHO, MCHO, and SUV254 results are presented as the means ± standard deviations. Relationships between the parameters were tested by Pearson correlation analysis in SPSS 16.0.
Results
The WT in Lake Taihu and its tributaries decreased gradually from September 2012 to January 2013 and then increased in February 2013 ((A)). EC ranged from 0.208 to 0.785 mS cm−1 and varied among all sampling sites ((B)).
Figure 2. Variations of (A) water temperature, (B) electrical conductivity, (C) chlorophyll a, and (D) bacterial abundance in Lake Taihu and its tributaries from September 2012 to February 2013. Each symbol represents a different sampling site (1–7). The EC and BA were not measured in September 2012.
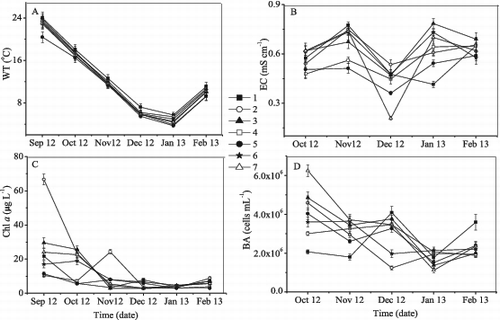
Chl a concentrations were less than 10 μg L−1 from December 2012 to February 2013 at all the seven sites. At sites 2, 3, and 7, Chl a concentrations decreased from September 2012 to December 2012 and then increased. At site 1, Chl a concentrations decreased rapidly from September 2012 to November 2012 and then fluctuated from December 2012 to February 2013. At site 6, Chl a concentration decreased to its minimum value in January 2013 but then rose in February 2013 ((C)).
At sites 3, 5, and 7, BA decreased to its minimum value in January 2013 and then increased in February 2013. At sites 2 and 6, BA decreased, reaching its minimum value in December 2012; it then increased in January 2013 and plateaued in February 2013. At site 4, BA increased from October 2012 to December 2012 and then decreased to its lowest value in February 2013. At site 1, BA varied, with maximum and minimum values observed in December 2012 and January 2013, respectively ((D)).
At site 5, the DOC concentration increased from September 2012 to November 2012 and then decreased to a minimum value of 3.87 mg L−1 in February 2013. At all other sites, the maximum DOC concentrations were observed in January 2013. At site 4, the DOC concentration increased from September 2012, reaching its maximum value in January 2013 and then decreasing in February 2013. At sites 1 and 2, DOC concentrations decreased from September 2012 to October 2012 and then exhibited the same pattern as site 4. In September 2012 and January 2013, DOC concentrations decreased along the transect from site 1 to site 5. This spatial distribution was also observed along the transect from site 6 to site 5 in January 2013 ((A)).
Figure 3. Variations of (A) DOC, (B) δ13CDOC, (C) PCHO, (D) MCHO, and (E) SUVA254 in Lake Taihu and its tributaries from September 2012 to January 2013. See text for definitions of acronyms. Each symbol represents a different sampling site (1–7). The sample for δ13CDOC analysis in Chendonggang River in September 2012 was lost.
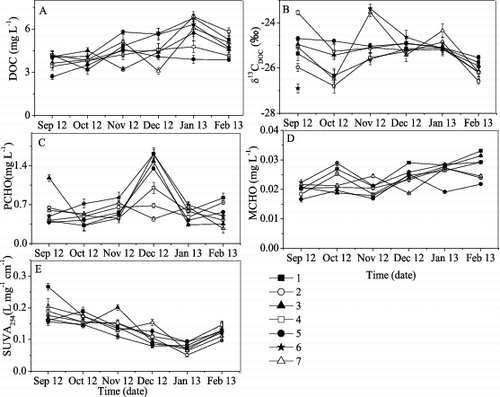
The δ13CDOC values varied widely, ranging from −26.8‰ to −23.5‰ in Lake Taihu and from −26.9‰ to −23.4 ‰ in the tributaries ((B)). The general distribution pattern of TCHO was similar to that of PCHO. The concentration of PCHO ranged from 0.27 ± 0.08 to 1.62 ± 0.11 mg L−1 in Lake Taihu and its tributaries between September 2012 and February 2013. The maximum PCHO concentrations were observed in December 2012 at all sites except sites 4 and 7 ((C)). The MCHO concentration was much lower than the PCHO concentration ((D)).
SUVA254 ranged from 0.052 ± 0.005 to 0.27 ± 0.01 L mg−1 cm−1 in Lake Taihu and its tributaries. It decreased from September 2012 to January 2013, subsequently increasing in February 2013 ((E)).
The WT was significantly and positively correlated with Chl a and BA and was negatively correlated with DOC and MCHO. EC was significantly and negatively correlated with PCHO. The BA correlated significantly and positively with Chl a and SUVA254, but significantly and negatively with DOC. PCHO was significantly and positively correlated with TCHO ().
Table 1. Pearson correlation coefficients between DOC and water parameters in Lake Taihu and its tributaries (n = 35 for EC and BA, 41 for δ13CDOC, and 42 for others).
Discussion
As our study was limited to the northwestern region of Lake Taihu, we cannot address the changes in the quality and quantity of DOC over the entire lake. DOC concentration has exhibited significant spatiotemporal heterogeneity. A wide range of DOC concentrations (0.65–4.68 mg L−1) was reported in winter (Yao et al. Citation2011), with higher concentrations (5.34–9.34 mg L−1) in summer (Liu et al. Citation2009). The DOC concentrations recorded in our study are consistent with previous studies (Yao et al. Citation2011; Zhang et al. Citation2011).
Phytoplankton are the main source of DOC-supporting bacterial communities, and approximately 50% of daily primary production can be released by phytoplankton as DOC (Karl et al. Citation1998) as a result of cell lysis or direct exudation (Nagata Citation2000). High-molecular-weight DOC (Ye, Shi, Wu, Zhang, et al. Citation2011) was found to be released via phytoplankton cell lysis (Ye, Shi, Wu, & Kong Citation2011) in autumn and winter in Lake Taihu. However, we found no significant correlation between Chl a and DOC concentration in the present study, consistent with previous results (Ye, Shi, Wu, & Kong Citation2011). This finding indicates that phytoplankton-derived carbon is not the determining factor affecting DOC dynamics.
Zweifel et al. (Citation1995) suggested that riverine DOC has a major impact on coastal DOC dynamics. In the Taihu basin, the volumes of inflow water in Chendonggang and Yincungang rivers ranked first and third, respectively, among the main 13 inflow rivers (Qin et al. Citation2007; Shi et al. Citation2012). In 2009, the annual flux of inflow water was 5 × 108 and 14 × 108 m3 in Yincungang and Chendonggang rivers, respectively (Yan et al. Citation2011), accounting for 11% and 32% of the total lake volume, respectively. Therefore, it would be reasonable to assume that the dynamics of DOC are influenced by riverine input. Where water bodies of different chemical composition meet, EC can be used as an index of solute mixing (Sugiyama et al. Citation2004). Monteiro et al. (Citation2014) predicted DOC concentrations in an Amazonian black-water catchment using EC measures. Moreover, a significant positive correlation between DOC and EC was observed in both mesotrophic Lake Ikeda (Sugiyama et al. Citation2004) and Yasu River (Mostofa et al. Citation2007) in Japan. Large amounts of untreated wastewater from factories and residential areas are discharged into Chendonggang and Yincungang rivers (Yang et al. Citation2012), a significant difference (n = 5, p = 0.04, two-tailed t-test) in EC was observed between lake center and Chendonggang River. Moreover, a positive relationship was apparent in Lake Taihu (n = 25, r = 0.5, p = 0.01), indicating that a fraction of DOC is input from the inflow rivers (Yoshioka et al. Citation2002; Sugiyama et al. Citation2004).
Several studies suggest that lake currents impact phosphorus levels and phytoplankton biomass distributions in Lake Taihu (Ma & Cai Citation1997; Qin et al. Citation2007; Wu et al. Citation2010). There is a counterclockwise current in the inner region of Zhushan Bay (Li Citation2006; Hu et al. Citation2010) that promotes DOC transportation and explains the nonsignificant difference in DOC among sampling sites.
Few data are available regarding the variation in δ13CDOC in Lake Taihu and its tributaries. The δ13CDOC values observed in the present study are within the same range as those observed in Lake Kasumigaura (Nara et al. Citation2007). In Lake Biwa, a higher δ13CDOC value (−22.7‰) was observed over the productive seasons and a lower value (−27.2‰) over the less productive seasons (Maki et al. Citation2010). In Lake Taihu and its tributaries, most δ13CDOC values were more enriched in 13C relative to the terrestrial signature (−27‰, Zeng Citation2008), which indicates an influence of an autochthonous source in autumn and winter (Nara et al. Citation2007; Maki et al. Citation2010). In addition, the preferential utilization of lighter C (12C) isotopes by microbes during DOC decomposition may have also contributed to the observed higher δ13C values (Schiff et al. Citation1997; Kang & Mitchell Citation2013).
Carbohydrates are among the most important biopolymers in freshwater systems (Sigleo Citation1996). This study provides the first available data-set of TCHO, PCHO, and MCHO concentrations in Lake Taihu and its inflow rivers. The MCHO concentrations were low in Lake Taihu, consistent with those in Lake Biwa (Hayakawa Citation2004). The high proportion of PCHO to TCHO (92%–96%) in the tributaries was in contrast to that found in riverine environments (Hung et al. Citation2005; Guéguen et al. Citation2006), suggesting that not all carbohydrates originate from the decomposition products of soil litter (Hedges et al. Citation1994). The contribution of TCHO to DOC is defined as the carbohydrate yield (Khodse et al. Citation2010; Wu & Yang Citation2013) and varied from 6% to 34% in the present study, close to the range (15%–30%) recorded for freshly derived organic matter from phytoplankton (Pakulski & Benner Citation1994). Thus, the high proportion of PCHO to TCHO may be due to the in situ production of high-molecular-weight PCHO compounds by phytoplankton or bacteria (Hung et al. Citation2003; Guimarães et al. Citation2013). However, TCHO, PCHO, and MCHO were not correlated with Chl a, consistent with the studies in the Yellow Sea and the northern East China Sea in early winter (Wu & Yang Citation2013). Riverine input has been reported to significantly influence carbohydrate distribution (Yang et al. Citation2010). A maximum monthly runoff rate was observed in December in Chendonggang and Yincungang rivers from September 2001 to January 2002 (Qin et al. Citation2007). In the present study, the maximum concentrations of TCHO and PCHO were also observed in December 2012 at almost all sampling sites (i.e., all except sites 4 and 7), implying that riverine input is likely to contribute to the higher carbohydrate concentrations.
SUVA254 has been used for evaluating aromaticity and hence the presence of humic-like compounds in DOC (Weishaar et al. Citation2003; Inamdar et al. Citation2012). In Lake Kasumigaura in Japan, SUVA254 of the river was greater than that of the lake water (Imai et al. Citation2001; Kim et al. Citation2002), whereas in the mesotrophic Lake Unmum, the ratios in river waters were lower than those in lake waters (Kim et al. Citation2006). In this study, no significance differences in SUVA254 were observed among sampling sites, suggesting a homogenous composition of humic-like compounds in Lake Taihu and its tributaries in autumn and winter.
Previous studies have reported that BA is controlled by DOC concentration (Naganuma & Seki Citation1993; Neogi et al. Citation2011) and that bacteria can rapidly utilize DOC of autochthonous origin (Chen & Wangersky Citation1996). However, the interaction between DOC and BA is complex, as the interaction can be affected by other factors, such as photodegradation of DOC (Lindell et al. Citation1995; Catalán et al. Citation2013) and bacteria loss due to sedimentation and grazing (Work & Havens Citation2003; Tang et al. Citation2010). For example, a significant positive correlation between DOC and BA was found in a productive pond water ecosystem (Fortu & Antai Citation2013) and a lack of correlation between DOC and BA was observed in Barguzin Bay of Lake Baikal (Ueno et al. Citation2005). In the present study, BA correlated positively with Chl a and negatively with DOC. These results suggest that BA might have increased under high algae conditions and corresponding high DOC, with exogenous DOC contributing less to BA than autochthonous DOC (Liu et al. Citation2010). In other words, quality, not quantity, of DOC determines BA.
Variation in organic carbon is typically accompanied by pronounced changes in BA (Smith et al. Citation1995). On the other hand, bacteria play significant roles as both remineralizers of organic carbon and trophic intermediaries in aquatic ecosystems. Thus, elucidating the relationships between DOC dynamics and BA is a significant ecological issue. Temperature is among the most important ecological parameters affecting phytoplankton composition, bacterial growth, and biological activity (White et al. Citation1991; Kobari et al. Citation2010; Neogi et al. Citation2011). Bacterial abundances in our study were within the range reported by Zhu et al. (Citation2009) in their study of Lake Taihu. In the present study, a significant positive relationship between bacterial count and WT was observed in Lake Taihu and its tributaries. At high temperatures, bacteria exhibit higher metabolic rates and can degrade organic substrates more rapidly (Pomeroy & Wiebe Citation2001). Accordingly, a negative correlation between DOC concentration and WT was found in the present study and in the eastern Japan Sea (Kim & Kim Citation2013), indicating that DOC concentrations are controlled by the temperature-dependent degradation reactions of bacteria.
Carbohydrates are useful substrates for heterotrophic bacteria (Hanisch et al. Citation1996; Piontek et al. Citation2011). The distribution of TCHO to DOC is useful for assessing the degradation state of organic substances (Hung et al. Citation2005; Khodse et al. Citation2010). Hung et al. (Citation2005) observed a negative linear relationship between WT and TCHO/DOC ratio, suggesting that microbial utilization of carbohydrates was strongly influenced by WT. In addition, bacterial cell numbers were significantly and negatively correlated with TCHO/DOC in the Mandovi estuary (Khodse et al. Citation2010). However, such relationships were not observed in the present study, indicating that the effects of bacterial metabolism on the dynamics of carbohydrates during autumn and winter are not straightforward (Wu & Yang Citation2013).
Previous studies have indicated that the available DOC contributes a significant fraction to humic substances in Lake Balaton and River Zala (Tóth et al. Citation2007) and that humic substances are an important substrate for planktonic bacteria (V.-Balogh & Vörös Citation1997). A significant positive correlation between SUVA254 and BA was observed in a high mountain lake (Hörtnagl et al. Citation2010); this finding was confirmed by the result of the present study, indicating that the humic fraction of DOC can be utilized by bacteria in Lake Taihu and its tributaries in autumn and winter. However, it remains unclear whether the bacteria-based pathway transfers carbon efficiently, as bacterial growth efficiency was not measured in this study.
Conclusions
Little is known regarding the relationship between DOC and BA in Lake Taihu and its tributaries. In the present study, we determined the chemical and spectroscopic characters and stable carbon isotope ratios of DOC and investigated the correlations between environmental factors and DOC. Our results showed that the release of carbon from phytoplankton during the decline phase is not the sole factor affecting DOC dynamics. DOC distribution was affected by both riverine input and lake currents. Both autochthonous and allochthonous sources contribute to the DOC pool in Lake Taihu and its tributaries in autumn and winter. Furthermore, temperature-dependent degradation by bacteria shapes the dynamics of DOC and humic substances might serve as a substrate for bacteria in Lake Taihu and its tributaries. Further research is needed to fully understand the relationship between DOC variation and bacterial production and respiration, which can be evaluated by measuring the labile and semi-labile fraction of DOC and the bacterial growth efficiency.
Acknowledgements
We are grateful to Ji Jiang, Tang Xiangming, Shen Xing, Qian Rongshu, and Huang Jianming for their assistance with sample collection and analysis.
Additional information
Funding
References
- Azam F, Fenchel T, Field JG, Gray JS, Meyer-Reil LA, Thingstad F. 1983. The ecological role of water-column microbes in the sea. Marine Ecol Prog Ser. 10:257–263.
- Bergström A-K, Jansson M. 2000. Bacterioplankton production in humic Lake Örträsket in relation to input of bacterial cells and input of allochthonous organic carbon. Microb Ecol. 39:101–115.
- Burkowska A, Donderski W. 2007. Impact of humic substances on bacterioplankton in eutrophic lake. Polish J Ecol. 55:155–160.
- Catalán N, Obrador B, Felip M, Pretus JL. 2013. Higher reactivity of allochthonous vs. autochthonous DOC sources in a shallow lake. Aquatic Sci. 75:581–593.
- Chen W, Wangersky PJ. 1996. Rates of microbial degradation of dissolved organic carbon from phytoplankton cultures. J Plankton Res. 18:1521–1533.
- Chrost RJ, Münster U, Rai H, Albrecht D, Witzel KP, Overbeck J. 1989. Photosynthetic production and exoenzymatic degradation of organic matter in the eutrophic zone of a eutrophic lake. J Plankton Res. 11:223–242.
- Duan S, Bianchi TS, Shiller AM, Dria K, Hatcher PG, Carman KR. 2007. Variability in the bulk composition and abundance of dissolved organic matter in the lower Mississippi and Pearl rivers. J Geophys Res. 112:G02024.
- Fortu RT, Antai EE. 2013. The dynamics of bacterial population during growth and decomposition of phytoplankton in a tropical productive pond water ecosystem. Afr J Microbiol Res. 7:5625–5631.
- Guéguen C, Guo LD, Wang DL, Tanaka N, Hung CC. 2006. Chemical characteristics and origin of dissolved organic matter in the Yukon River. Biogeochemistry. 77:139–159.
- Guillemette F, del Giorgio PA. 2012. Simultaneous consumption and production of fluorescent dissolved organic matter by lake bacterioplankton. Environ Microbiol. 14:1432–1443.
- Guimarães PS, Zigiotto L, Garcia M, Dellamano-Oliveira MJ, Vieira AAH, Giroldo D. 2013. Phytoplankton relationship with bacterioplankton, dissolved carbohydrates and water characteristics in a subtropical coastal lagoon. J Limnology. 72:543–554.
- Hanisch K, Schweitzer B, Simon M. 1996. Use of dissolved carbohydrates by planktonic bacteria in a mesotrophic lake. Microb Ecol. 31:41–55.
- Hayakawa K. 2004. Seasonal variations and dynamics of dissolved carbohydrates in Lake Biwa. Org Geochem. 35:169–179.
- Hedges JI, Cowie GL, Richey JE, Quay PD, Benner R, Strom M, Forsberg BR. 1994. Origins and processing of organic matter in the Amazon River as indicated by carbohydrates and amino acids. Limnology Oceanography. 39:743–761.
- Hörtnagl P, Pérez MT, Zeder M, Sommaruga R. 2010. The bacterial community composition of the surface microlayer in a high mountain lake. FEMS Microbiol Ecol. 73:458–467.
- Hu K, Yong P, Xie F, Jiang Y, Yang S. 2010. Effects of Zhihugang and Wujinggang closing sluices on water environment of Lake Zhushan, Lake Taihu. J Lake Sci. 22:923–929.
- Hung C-C, Guo L, Santschi PH, Alvarado-Quiroz N, Haye JM. 2003. Distributions of carbohydrate species in the Gulf of Mexico. Marine Chem. 81:119–135.
- Hung C-C, Warnken KW, Santschi PH. 2005. A seasonal survey of carbohydrates and uronic acids in the Trinity River, Texas. Org Geochem. 36:463–474.
- Imai A, Fukushima T, Matsuschige K, Kim YH. 2001. Fractionation and characterization of dissolved organic matter in a shallow eutrophic lakes, its inflowing rivers, and other organic matter sources. Water Res. 35:4019–4028.
- Inamdar SP, Finger N, Singh S, Mitchell M, Levia D, Bais H, Scott D, McHale P. 2012. Dissolved organic matter (DOM) concentrations and quality in a forested mid-Atlantic watershed, USA. Biogeochemistry. 108:55–76.
- Kaldy JE, Cifuentes LA, Brock D. 2005. Using stable isotope analyses to assess carbon dynamics in a shallow subtropical estuary. 28:86–95.
- Kang P-G, Mitchell MJ. 2013. Bioavailability and size-fraction of dissolved organic carbon, nitrogen, and sulfur at the Arbutus Lake watershed, Adirondack Mountains, NY. Biogeochemistry. 115:213–234.
- Karl DM, Hebel DV, Bjorkman K, Letelier RM. 1998. The role of dissolved organic matter release in the productivity of the oligotrophic North Pacific Ocean. Limnology Oceanography. 43:1270–1286.
- Kawasaki N, Komatsu K, Kohzu A, Tomioka N, Shinohara R, Satou T, Watanabe FN, Tada Y, Hamasaki K, Kushairi MRM, Imai A. 2013. Bacterial contribution to dissolved organic matter in eutrophic lake Kasumigaura, Japan. Appl Environ Microbiol. 79:7160–7168.
- Khodse VB, Bhosle NB, Matondkar SGP. 2010. Distribution of dissolved carbohydrates and uronic acids in a tropical estuary, India. J Earth Syst Sci. 119:519–530.
- Kim T-H, Kim G. 2013. Factors controlling the C:N:P stoichiometry of dissolved organic matter in the N-limited, cyanobacteria-dominated East/Japan Sea. J Marine Syst. 115–116:1–9.
- Kim Y-H, Lee S-H, Imai A, Matsushige K. 2002. Characterization of dissolved organic matter in a shallow eutrophic lake and inflowing waters. Environ Eng Res. 7:93–101.
- Kim Y-H, Lee S-H, Kim J-H, Park J-W, Choi K. 2006. Characterization of recalcitrant dissolved organic matter in lake and inflow river waters. Environ Eng Res. 11:181–193.
- Kobari T, Fujii T, Kobari Y, Habano A. 2010. Seasonal variations in abundance, growth and mortality of heterotrophic bacteria in Kagoshima Bay. J Oceanography. 66:845–853.
- Kritzberg ES, Cole JJ, Pace ML, Granéli W, Bade DL. 2004. Autochthonous versus allochthonous carbon sources of bacteria: results from whole-lake 13C addition experiments. Limnology Oceanography. 49:588–596.
- Lasternas S, Agustí S. 2013. Bacterial survival governed by organic carbon release from senescent oceanic phytoplankton. Biogeosciences Discuss. 10:16973–16999.
- Li YP. 2006. Study on the influence factors of transparency by experiment and numerical model in Lake Taihu [dissertation]. Nanjing (China): Hohai University. Chinese.
- Lindell MJ, Granéli W, Tranvik LJ. 1995. Enhance bacterial growth in response to photochemical transformation of dissolved organic matter. Limnology Oceanography. 40:195–199.
- Liu M, Zhang Y, Qin B. 2009. Characterization of absorption and three-dimensional excitation-emission matrix spectra of chromophoric dissolved organic matter at the river inflow and the open area in Lake Taihu. J Lake Sci. 21:234–241.
- Liu X, Lu X, Chen Y. 2012. [Temporal and spatial dynamics of Cryptophytes biomass in the north of Lake Taihu]. J Lake Sci. 24:142–148. Chinese.
- Liu X, Yao T, Kang S, Jiao N, Zeng Y, Liu Y. 2010. Bacterial community of the largest Oligosaline Lake, Namco on the Tibetan Plateau. Geomicrobiol J. 27:669–682.
- Ma S, Cai Q. 1997. Numerical study on the distribution of TP in Taihu Lake and the impact from lake current. J Lake Sci. 9:325–330.
- Maki K, Kim C, Yoshimizu C, Tayasu I, Miyajima T, Nagata T. 2010. Autochthonous origin of semi-labile dissolved organic carbon in a large monomictic lake (Lake Biwa): carbon stable isotopic evidence. Limnology. 11:143–153.
- Meon B, Kirchman DL. 2001. Dynamics and molecular composition of dissolved organic material during experimental phytoplankton blooms. Marine Chem. 75:185–199.
- Monteiro MTF, Oliveira SM, Luizão FJ, Cândido LA, Ishida FY, Tomasella J. 2014. Dissolved organic carbon concentration and its relationship to electrical conductivity in the waters of a stream in a forested Amazonian blackwater catchment. Plant Ecol Divers. 7:205–213.
- Moran MA, Hodson RE. 1990. Bacterial production on humic and nonhumic components of dissolved organic carbon. Limnology Oceanography. 35:1744–1756.
- Mostofa KMG, Yoshioka T, Konohira E, Tanoue E. 2007. Dynamics and characteristics of fluorescent dissolved organic matter in the groundwater, river and lake water. Water Air Soil Pollut. 184:157–176.
- Myklestad SM, Skånøy E, Hestmann S. 1997. A sensitive and rapid method for analysis of dissolved mono- and polysaccharides in seawater. Marine Chem. 56:279–286.
- Naganuma T, Seki H. 1993. Abundance and productivity of bacterioplankton in a eutrophication gradient of Shimoda Bay. J Oceanography. 49:657–665.
- Nagata T. 2000. Production mechanisms of dissolved organic matter. In: Kirchman D, editor. Microbial ecology of the oceans. New York (NY): Wiley; p. 121–151.
- Nara F, Imai A, Yoneda M, Matsushige K, Komatsu K, Nagai T, Shibata Y, Watanabe T. 2007. Seasonal variation in sources of dissolved organic carbon in a lacustrine environment revealed by paired isotopic measurements (Δ14C and δ13C). Radiocarbon. 49:767–773.
- Neogi SB, Koch BP, Schmitt-Kopplin P, Pohl C, Kattner G, Yamasaki S, Lara RJ. 2011. Biogeochemical controls on the bacterial populations in the eastern Atlantic Ocean. Biogeosciences. 8:3747–3759.
- Pakulski JD, Benner R. 1994. Abundance and distribution of carbohydrates in the ocean. Limnology Oceanography. 39:930–940.
- Pápista É, Ács É, Böddi B. 2002. Chlorophyll – a determination with ethanol ― a critical test. Hydrobiologia. 485:191–198.
- Pierson-Wickmann A-C, Gruau G, Jardé E, Gaury N, Brient L, Lengronne M, Crocq A, Helle D, Lambert T. 2011. Development of a combined isotopic and mass-balance approach to determine dissolved organic carbon sources in eutrophic reservoirs. Chemosphere. 83:356–366.
- Piontek J, Händel N, De Bodt C, Harlay J, Chou L, Engel A. 2011. The utilization of polysaccharides by hetetrotrophic bacterioplankton in the Bay of Biscay (North Atlantic Ocean). J Plankton Res. 33:1719–1735.
- Pomeroy LR, Wiebe WJ. 2001. Temperature and substrate as interactive limiting factors for marine heterotrophic bacteria. Aquatic Microb Ecol. 23:187–204.
- Qin B, Xu P, Wu Q, Luo L, Zhang Y. 2007. Environmental issues of Lake Taihu, China. Hydrobiologia. 581:3–14.
- Raymond PA, Bauer JE. 2000. Bacterial consumption of DOC during transport through a temperate estuary. Aquatic Microb Ecol. 22:1–12.
- Schiff SL, Aravena R, Trumbore SE, Hinton MJ, Elgood R, Dillon PJ. 1997. Export of DOC from forested catchments on the Precambrian Shield of Central Ontario: clues from 13C and 14C. Biogeochemistry. 36:43–65.
- Shi YD, Mo LJ, Cheng YH. 2012. [Countermeasures for water allocation program of Taihu Lake]. J Econ Water Resour. 30:49–51. Chinese.
- Sigleo AC. 1996. Biochemical composition in suspended particles and colloids: carbohydrates in the Potomac and Patuxent Rivers. Org Geochem. 24:83–93.
- Smith DC, Steward GF, Long AR, Azam F. 1995. Bacterial mediation of carbon fluxes during a diatom bloom in a mesocosm. Deep Sea Res II. 42:75–97.
- Søndergaard M, Middelboe M. 1995. A cross-system analysis of labile dissolved organic carbon. Marine Ecol Prog Ser. 118:283–294.
- Sugiyama Y, Anegawa A, Kugmgagal T, Harita Y, Hori T, Sugiyama M. 2004. Distribution of dissolved organic carbon in lakes of different trophic types. Limnology. 5:165–176.
- Tang X, Gao G, Chao J, Wang X, Zhu G, Qin B. 2010. Dynamics of organic-aggregate-associated bacterial communities and related environmental factors in Lake Taihu, a large eutrophic shallow lake in China. Limnology Oceanography. 55:469–480.
- Tóth N, Vörös L, Mózes A, V.-Balogh K. 2007. Biological availability and humic properties of dissolved organic carbon in Lake Balaton (Hungary). Hydrobiologia. 592:281–290.
- Trabelsi A, Rassoulzadegan F. 2011. Effects of bacterial community dynamics on DOC seasonal changes in the north-western Mediterranean Sea. J Plankton Res. 33:1249–1262.
- Ueno H, Katano T, Nakano S, Mitamura O, Anbutsu K, Satoh Y, Drucker V, Sugiyama M. 2005. Abundance and community structure of picoplankton and protists in the microbial food web of Barguzin Bay, Lake Baikal. Aquatic Ecol. 39:263–270.
- V.-Balogh K, Vörös L. 1997. High bacterial production in hypertrophic shallow reservoirs rich in humic substances. Hydrobiologia. 342/343:63–70.
- Weishaar JL, Aiken GR, Bergamaschi BA, Fram MS, Fujii R, Mopper K. 2003. Evaluation of specific ultraviolet absorbance as an indicator of the chemical composition and reactivity of dissolved organic carbon. Environ Sci Technol. 37:4702–4708.
- White PA, Kalff J, Rasumssen JB, Gasol JM. 1991. The effect of temperature and algal biomass on bacterial production and specific growth rate in Freshwater and Marine Habitats. Microb Ecol. 21:99–118.
- Work KA, Havens KE. 2003. Zooplankton grazing on bacteria and cyanobacteria in a eutrophic lake. J Plankton Res. 25:1301–1307.
- Wu G-W, Yang G-P. 2013. Distributions of dissolved carbohydrates in the Yellow Sea and the Northern East China Sea in Early Winter. J Coastal Res. 29:449–459.
- Wu X, Kong F, Chen Y, Qian X, Zhang L, Yu Y, Zhang M, Xing P. 2010. Horizontal distribution and transport processes of bloom-forming Microcystis in a large shallow lake (Taihu, China). Limnologica. 40:8–15.
- Xu P, Qin B. 2005. [Water quantity and pollutant fluxes of the surrounding rivers of Lake Taihu during the hydrological year of 2001−2002]. J Lake Sci. 17:213–218. Chinese.
- Yan S, Yu H, Zhang L, Xu J, Wang Z. 2011. [Water quality and pollutant fluxes of inflow and outflow rivers of Lake Taihu, 2009]. J Lake Sci. 23:855–862. Chinese.
- Yang G, Zhang Y, Lu X, Ding H. 2010. Distributions and seasonal variations of dissolved carbohydrates in the Jiaozhou Bay, China. Estuarine Coastal Shelf Sci. 88:12–20.
- Yang X, Luo X, Zheng Z, Fang S. 2012. [Explore the spatial and temporal patterns of water pollution in the Yincungang canal of the Lake Taihu basin, China]. Environ Sci. 33:3051–3055. Chinese.
- Yao X, Zhang Y, Zhu G, Qin B, Feng L, Cai L, Gao G. 2011. Resolving the variability of CDOM fluorescence to differentiate the sources and fate of DOM in Lake Taihu and its tributaries. Chemosphere. 82:145–155.
- Ye L, Shi X, Wu X, Kong F. 2011. Phytoplankton cell lysis after water bloom in a eutrophic freshwater Lake Taihu (China). Int Rev Hydrobiol. 96:709–719.
- Ye L, Shi X, Wu X, Zhang M, Yu Y, Li D, Kong F. 2011. Dynamics of dissolved organic carbon after a cyanobacterial bloom in hypereutrophic Lake Taihu (China). Limnologica. 41:382–388.
- Yoshioka T, Ueda S, Khodzher T, Bashenkhaeva N, Korovyakova I, Sorokovikova L, Gorhunova L. 2002. Distribution of dissolved organic carbon in Lake Baikal and its watershed. Limnology. 3:159–168.
- Zeng QF. 2008. Stable isotope compositions of suspended particulate organic matter and its ecological significance from Taihu Lake [dissertation]. Nanjing (China): Nanjing Institute of Geography and Limnology, Chinese Academy of Science. Chinese.
- Zhang Y, Yin Y, Liu X, Shi Z, Feng L, Liu M, Zhu G, Gong Z, Qin B. 2011. Spatial – seasonal dynamics of chromophoric organic matter in Lake Taihu, a large eutrophic, shallow lake in China. Org Geochem. 42:510–519.
- Zhang ZP, Sun XJ, Lou ZH, Chi QQ, Zhu GW. 2006. [Analysis of colloidal organic carbon content and its influencing factors in the waters of Taihu Lake in spring]. Chin Environ Sci. 26:166–170. Chinese.
- Zhu GW. 2008. [Eutrophic status and causing factors for a large, shallow and subtropical Lake Taihu]. J Lake Sci. 20:21–26. Chinese.
- Zhu LP, Gao G, Tang XM, Cao JY, Shen Y. 2009. [Variation of bacteria in water and attached on Microcystis colonies and their activity during the algal blooms]. J Lake Sci. 21:395–400. Chinese.
- Ziegler SE, Brisco SL. 2004. Relationships between the isotopic composition of dissolved organic carbon and its bioavailability in contrasting Ozark streams. Hydrobiologia. 513:153–169.
- Zweifel UL, Wikner J, Hagström Ǻ. 1995. Dynamics of dissolved organic carbon in a coastal ecosystem. Limnology Oceanography. 40:299–305.