Abstract
Movement behaviors attributed to spawning, dispersal, or altered habitat availability are essential to the ecology of many lotic fishes and, although considerable research has described movements of sport fish, little is known about the movement patterns of nongame species. Streams and rivers, wherein plains topminnow Fundulus sciadicus are prevalent, occur in a nonequilibrium state in which habitat patches are sporadically created and lost due to hydrologic variability. This results in regular extirpation and need for recolonization of many plains fishes, including plains topminnow. Species persistence, therefore, is dependent on tolerances to fluctuating habitat conditions and life-history traits that allow dispersal over large areas. To better understand the regional distribution of plains topminnow, we monitored large-scale dispersal patterns and habitat use of two introduced populations in Nebraska. In 2011 and 2012, plains topminnow were marked using visible implanted elastomer (VIE) marks and released at the center of two 3000-m study reaches. Populations were sampled monthly from April to November in 2011 and April through September in 2012 to describe movement patterns and habitat use. Plains topminnow were highly mobile, consistently associated with select habitat features, and the estimated individual home range exceeded standard sampling reach distances by nearly four times. The movement of plains topminnow occurred at greater rates and to distances further than known for similar species. These large-scale movements likely help maintain connectivity among populations within stream drainages and facilitate recolonization of regularly extirpated habitat patches. In this context, species movement may be critical to the maintenance and potential recovery of populations of this and other rare lotic fishes.
Introduction
Movement behaviors attributed to spawning, dispersal, and responses to fluctuating habitat availability are common among lotic fishes (Peterson & Bayley Citation1993; Dingle Citation1996; Schmetterling & Adams Citation2004). Although there has been considerable research evaluating movement patterns of sport fish species (Rodríguez Citation2002; Schmetterling & Adams Citation2004; VanArnum et al. Citation2004), details concerning the movement potential of nongame species remain mostly unknown (Smithson & Johnston Citation1999; Hudy & Shiflet Citation2009; Hodges & Magoulick Citation2011). Understanding movement and spatially explicit patterns of habitat use is central to developing concepts of large-scale lotic ecology (Fausch et al. Citation2002; Scheurer et al. Citation2003).
Stream fish populations have been considered homogenous with individuals moving freely throughout a system (Gowan et al. Citation1994; Fausch & Young Citation1995) or as relatively discrete populations with restricted movement (Gerking Citation1959; Heggeness et al. Citation1991; Larson et al. Citation2002). The recognition of complex dispersal patterns in stream fishes and frequent long-distance movement (e.g., Fausch & Young Citation1995; Gowan & Fausch Citation1996; Hudy & Shiflet Citation2009) is contrary to the notion that stream fishes are completely sedentary (Fausch et al. Citation2002). Movement behaviors likely are necessary responses to changing resource abundance, distribution, and quality that cease when suitable habitat patches are encountered (Dingle Citation1996; Fausch et al. Citation2002). However, the scale at which these movement behaviors transpire has varied greatly among taxonomic groups and is commonly contingent upon distances to important habitats or mobility of the species (Skalski & Gilliam Citation2000; Kahler et al. Citation2001; Rodríguez Citation2002; Hudy & Shiflet Citation2009). Therefore, dispersal events are important to the regulation of population structure, connection of spatially divided populations, colonization of newly accessible habitat patches, and recolonization of areas where populations have been extirpated (Schlosser & Angermeier Citation1995; Taylor Citation1997; Lonzarich et al. Citation1998; Hughes Citation1999; Johnston Citation2000).
The Great Plains contain a variety of diverse and dynamic aquatic systems with high variation in physical and chemical properties (Poff & Ward Citation1989; Rinne & Stefferud Citation1999; Dodds et al. Citation2004; Franssen et al. Citation2006). Lotic systems of the region oscillate between precipitous states; consequently, habitat patches are sporadically created and lost (Fausch et al. Citation2002; Scheurer et al. Citation2003; Dodds et al. Citation2004; Franssen et al. Citation2006). Plains fishes are typically tolerant of wide fluctuations in habitat conditions at a local scale (Smale & Rabeni Citation1995) and have life-history traits that result in dispersal (Matthews & Marsh-Matthews Citation2003; Scheurer et al. Citation2003; Dodds et al. Citation2004; Gido et al. Citation2010).
Plains topminnow, Fundulus sciadicus, populations have declined in both distribution and local abundance throughout their native range (Rahel & Thel Citation2004; Pasbrig et al. Citation2012). Declining water quality and quantity, habitat degradation, population fragmentation, and the introduction of nonnative fishes have resulted in declines of other Great Plains stream fishes (Haslouer et al. Citation2005), and likely influence the persistence of this species (Rahel & Thel Citation2004; Pasbrig et al. Citation2012). Plains topminnow is a habitat specialist that occurs in widely distributed, isolated, and dynamic habitat patches characterized by shallow, stagnant water that are especially vulnerable to desiccation by means of prevalent agricultural activities that divert water from the channel or lower the water table (Rahel & Thel Citation2004; Fischer & Paukert Citation2008; Pasbrig et al. Citation2012). The isolated nature of plains topminnow populations suggests that stream connectivity may be important to their long-term persistence (Cross & Collins Citation1995; Pflieger Citation1997; Rahel & Thel Citation2004; Pasbrig et al. Citation2012).
Movement behaviors permit the occupancy of irregularly distributed and sporadically available habitat patches important to lotic fish occurrence. In recent years, the understanding of movement patterns and habitat use of sport fish species has greatly increased; however, little is currently known about the movement behaviors of many nongame fishes. To further understand the regional population dynamics of plains topminnow and the influence of dispersal on large-scale conservation activities, the movement patterns and spatially explicit habitat use of two introduced populations were monitored in Nebraska over a two-year period.
Methods
Study area
Two low-order prairie streams in which plains topminnow were thought to have been locally extirpated were selected for reintroduction to evaluate movement patterns and habitat use as part of an effort to recover the species in Nebraska. Historic occurrences were identified using the Nebraska Game and Parks Fish Inventory Database and were recently confirmed in a range-wide survey to describe the species status (Pasbrig et al. Citation2012). Both streams are located in the 39,103 km2 Loup River Basin in central Nebraska. Human settlement in the area is sparse with land use dominated by pasture and to a lesser degree groundwater-irrigated crop cultivation. Plains topminnow were historically common throughout the Loup River Basin; however, following substantial declines the species currently occurs sporadically in relatively close proximity to the selected reintroduction locations (Pasbrig et al. Citation2012).
Sweet Creek is a low-gradient, meandering stream with a broad, well-developed floodplain that flows through a sand-dominated alluvial valley in the 257-km2 Sweet Creek subwatershed (). Sweet Creek is located near the confluence of the South Loup River near Ravenna, NE. In the 3000-m stream reach selected for study, the available macrohabitat included isolated perennial pools, limited backwater, and scour pools, and many reaches with minimal surface flow (e.g., glides) embedded within larger intermittent segments that expand and contract seasonally. The average gradient throughout Sweet Creek is <0.01% (elevation of 602–605 m), and at base flow the wetted width (mean ± standard error, SE) was 5.84 ± 0.20 m during 2011 and 2012. Sand was the dominant substrate found in association with abundant slow-moving glides, but finer substrates were present in the isolated backwater areas. The most abundant resident fish species included sand shiner, Notropis stramineus, bigmouth shiner, Notropis dorsalis, and brassy minnow, Hybognathus hankinsoni. Largemouth bass, Micropterus salmoides, green sunfish, Lepomis cyanellus, bluegill, Lepomis macrochirus, fathead minnow, Pimephales promelas, red shiner, Cyprinella lutrensis, common carp, Cyprinus carpio, black bullhead, Ameiurus melas, and plains killifish, Fundulus kansae, were also present.
Figure 1. Prairie streams in the Loup River Basin, NE, which were sampled during 2011 and 2012 to describe movement behaviors and habitat use of introduced plains topminnow. The 3000-m sampling reaches (X) were sampled monthly to describe movement behaviors after reintroduction.
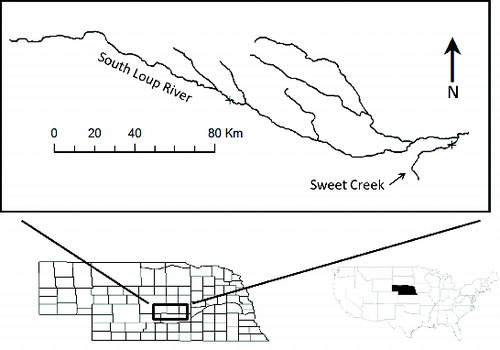
The South Loup River is a relatively low-gradient stream with highly entrenched banks and many meanders that flows through a sand-dominated alluvial valley in the 4099-km2 South Loup River subwatershed near Oconto, NE (). In the 3000-m stream segment selected for study, the most abundant macrohabitats consisted of perennial runs and low-velocity marginal areas with abundant overhanging vegetation. Isolated backwater habitats and scour pools were rare along the sampling reach but were consistently available. The South Loup River has an average gradient of approximately 0.01% (elevation of 725–729 m), and at base flow the wetted width was 25.9 ± 3.99 m during the two-year sampling period. Sand was the dominate substrate found in association with runs, with finer substrates available in areas with little flow. The most abundant resident fish were red shiner and sand shiner. However, fathead minnow, bigmouth shiner, brassy minnow, flathead chub, Platygobio gracilis, channel catfish, Ictalurus punctatus, green sunfish, bluegill, largemouth bass, river carpsucker, Carpiodes carpio, shorthead redhorse, Moxostoma macrolepidotum, white sucker, Catostomus commersonii, and common carp were also present.
Plains topminnow introductions
Plains topminnow were released at the center of the 3000-m sampling reach which limited upstream and downstream detection of dispersal to a maximum of 1500 m. Introductory population sizes were determined based upon the total area of habitat considered suitable for plains topminnow colonization, using velocity tolerances and introduction rates of western mosquitofish, Gambusia affinis, and Gila topminnow, Poeciliopsis occidentalis (Duryea et al. Citation1996; Ward et al. Citation2003). Water velocity was measured during base-flow conditions at five points along equally spaced transects throughout each sampling reach, and was interpolated to describe the total area available to plains topminnow. Areas with velocities less than 0.365 m/s were considered suitable for plains topminnow. A total of 1000 individuals per suitable hectare were released in March of 2011 and 2012 at each site. At Sweet Creek, a total of 662 adult (age 1+) individuals (total length, TL ± SE: 47.3 ± 0.53) were released in both 2011 and 2012. At the South Loup River, 770 adult (age 1+) individuals (TL ± SE: 47.3 ± 0.53) were introduced each year. All plains topminnow were obtained from an extensive aquaculture pond constructed to house a species-specific refuge population near Wilcox, NE (Schumann et al. Citation2012).
Plains topminnow were marked with red visible implanted elastomer (VIE) in 2011 and blue VIE marks in 2012. All VIE marks were injected under the integument on either side of the fish, either immediately below or slightly anterior to the dorsal fin. Experimental evaluation of long-term VIE mark retention and marking mortality of plains topminnow found zero-marking mortality and 100% retention (Schumann et al. Citation2013).
Plains topminnow sampling
Starting one month after introduction, sampling was conducted in an upstream direction using single-pass backpack electrofishing for the entire 3000-m study reach. Study reaches were sampled monthly from April to November in 2011 and between April and September in 2012. All captured plains topminnow were checked for a VIE mark and measured to the nearest 1 mm TL. Global positioning system coordinates were recorded for all plains topminnow captured to determine the distance moved. The macrohabitat type where individuals were captured was described and the substrate composition was visually estimated using a modified Wentworth classification for dominant type as silt/muck (<0.06 mm), sand (0.06–2 mm), fine gravel (2–16 mm), coarse gravel (16–64 mm), cobble (64–250 mm), and boulder (>250 mm) categories. The captured individuals were released approximately 50 m downstream of the capture location to avoid incidental recapture.
Data analysis
Marked fish were classified as sedentary or mobile in either the upstream or downstream direction. Individuals that did not move beyond the central reach (i.e., <150 m) were classified as sedentary and assigned a value of zero distance moved. Distance traveled by mobile individuals was measured relative to the center of the reintroduction reach with a maximum of 1500 m moved. The percent of recaptured individuals that were mobile was expressed as the ratio of recaptures that were mobile to those that were sedentary (Roberts & Angermeier Citation2007). Home range size was estimated as the median linear distance moved by recaptured individuals at each site (Valley et al. Citation2010). We evaluated movement patterns of plains topminnow by comparing the differences among the three movement groups (sedentary, upstream, and downstream) at each site and during each year using a Mann–Whitney U-test. To compare temporal movement patterns, we fit a linear regression model to movement distance for each recapture period. Habitat use was described as the proportion of captured individuals in association with each substrate and macrohabitat available at each site.
Results
Sweet Creek
Of the 1324 released plains topminnow, 40 marked individuals were recaptured which represented 7.3% of the 547 individuals captured during the monthly sampling ( and ). No plains topminnow that were marked in 2011 were encountered in 2012 sampling efforts; however, the number of recaptured individuals was greater in 2012 (n = 24) than 2011 (n = 16) (). Across years, three individuals were recaptured within the central release reach (). These individuals had zero movement whereas a significantly greater number of individuals (n = 37) were mobile (Mann–Whitney U-test: U = 143.5; p = 0.02). The average movement rate of plains topminnow was calculated as 93.3% (standard deviation, SD = 11.18) which increased through time (F1,12 = 5.09, r2 = 0.30, p = 0.04) as marked individuals moved away from the release point and with decreased discharge (). Plains topminnow were captured throughout the 3000-m study reach at Sweet Creek; however, a majority (Mann–Whitney U-test: U = 141.5; p = 0.03) of individuals were recaptured downstream of the release point (). The greatest movement distance observed was 1300 m with individuals moving this distance in both the upstream and downstream directions (). The median movement distance or the estimated home-range size was 655 m and was greater than four times the standard sampling reach for a stream of this size (i.e., 150 m).
Figure 2. Distribution of distances moved by released plains topminnow at Sweet Creek (A) and the South Loup River (B) in Nebraska, throughout a 3000-m sampling reach during April to November in 2011 (black) and April to September in 2012 (white).
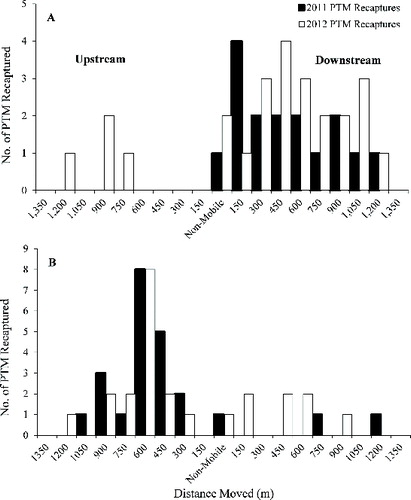
Figure 3. Mean monthly discharge (solid line) at Sweet Creek (A) and the South Loup River (B), in Nebraska, and total number of both marked (dark) and unmarked (white) plains topminnow captured during 2011 and 2012.
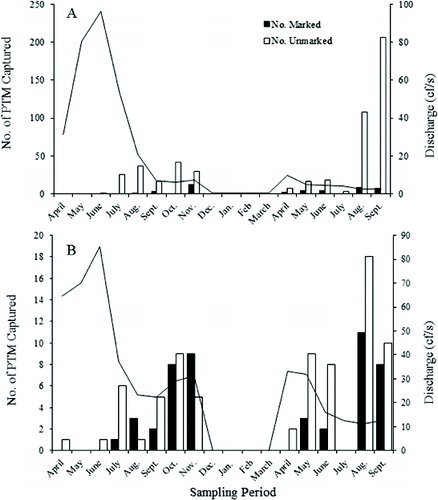
No plains topminnow individuals were captured at the reintroduction site (i.e., 40 times mean wetted width) during preliminary assessments of the fish community. However, after the release of marked individuals unmarked, adult (>25 mm TL) plains topminnow were encountered throughout the 3000-m sampling reach (). Unmarked juvenile and adult plains topminnow captured during the monthly sampling efforts were potentially wild individuals that were present prior to introductions or developed offspring from the hatchery stocked individuals. Regardless of origin, both marked and unmarked plains topminnow moved similarly throughout Sweet Creek, and were consistently captured in association with the same habitat features. Recaptured and unmarked plains topminnow were concentrated along the inner margin of a stream bend downstream of the release site (n = 469) and relatively few individuals (n = 78) were captured in the artificially channelized upstream reach (). A majority of marked and unmarked plains topminnow individuals were consistently captured along the unstable, stagnant stream margins (n = 404), while fewer individuals were encountered in glides (n = 87) and backwater habitats (n = 56). Nearly all plains topminnow were captured in association with sand substrates (92%), with few captured over fine substrates.
South Loup River
Of the 1540 released plains topminnow, 47 marked individuals were recaptured which represented 62.7% of the 74 individuals captured during the monthly sampling ( and ). The number of plains topminnow recaptured in 2011 (n = 23) was similar to the number captured in 2012 (n = 24) and no individuals released in 2011 were encountered in 2012 (). Across years, only two individuals exhibited no movement and were recaptured at the reintroduction site (), while a significantly greater number of individuals (n = 45) were considered mobile (Mann–Whitney U-test: U = 154.0; p = 0.004). At the South Loup River, plains topminnow movement rate was 97.8% (SD = 4.18), and the rate of movement and total capture were stable through time (F1,12 = 2.02, r2 = 0.14, p = 0.18) despite the decreasing discharge (). No significant difference between the number of fish that moved upstream (n = 36) and downstream (n = 9) was found, despite a greater number of individuals captured upstream (Mann–Whitney U-test: U = 72.5; p = 0.22; ). The greatest observed distance moved was 1255 m in the upstream direction, while the furthest downstream movement was 1200 m (). The estimated home range of recaptured plains topminnow was 635 m (), which were more than twice the previously designated sampling reach (i.e., 300 m).
No plains topminnow were captured at the reintroduction site during the preliminary assessments of the fish community. However, after the release of marked individuals in 2011 and during the 3000-m sampling conducted in summer 2011, adult (>25 mm TL) unmarked individuals were captured (). Unmarked plains topminnow had the potential to be wild individuals that were present prior to introductions or offspring of stocked individuals. Irrespective of source, both marked and unmarked plains topminnow were found in similar habitats in the South Loup River and were consistently captured in association with similar habitat features (). A majority of both unmarked and marked plains topminnow (n = 74) were captured upstream of the introduction location and those individuals were frequently captured in association with a large bend (80.5%). Location and extent of habitat patches were relatively consistent throughout the study and individuals were captured most often in a single, large backwater area (n = 44) but were also prevalent along the stream margin (n = 30). Most individuals were captured in association with fine (silt/muck) substrates (75%), with fewer individuals captured in association with sand substrates (25%).
Discussion
The demonstration of explicit dispersal patterns and frequent large-scale movements of plains topminnow challenges the assumption that the resident stream fishes exhibit restricted movement (Gerking Citation1959; Hill & Grossman Citation1987; Heggeness et al. Citation1991; Gowan et al. Citation1994; Fausch & Young Citation1995). This evidence of large-scale movement behaviors is in agreement with several contemporary studies of lotic fish movement in which similar patterns were found (Gowan & Fausch Citation1996). Although individual plains topminnow recaptured at both sites exhibited both sedentary and mobile behaviors, the movement rate and the distance traveled greatly exceeded values reported by previous studies of small lotic fishes (Schlosser Citation1998; Smithson & Johnston Citation1999; Larson et al. Citation2002; Rodríguez Citation2002). Additionally, our estimates of movement are likely conservative as most marked fish were not recaptured and many may have moved beyond the established 3000-m sampling reach. Plains topminnow were encountered in association with specific habitat types available at each site regardless of proximity to the release location. The occurrence of marked and unmarked individuals at specific habitat patches was similar; however, the presence of individuals from each cohort was sporadic through time suggesting regular movement behaviors occuring within these systems. These observations supplement a developing landscape paradigm for stream assemblages in which large-scale movement patterns are common in response to resource demands and habitat availability (Fausch & Young Citation1995; Gowan & Fausch Citation1996; Fausch et al. Citation2002). We acknowledge the possibility that our introduction of hatchery-reared plains topminnow influenced the rates and distances we observed; however, the research has shown that hatchery-reared fish can acclimate and behave similarly to wild individuals and use the entire study area (Jordan et al. Citation2006).
Direct comparisons to previous movement studies are difficult due to differing monitoring methods, timing, duration of studies, and limited knowledge of movement patterns of nongame fishes. However, movement was quantified for two species in Fundulidae: blackspotted topminnow, Fundulus olivaceus (Smithson & Johnston Citation1999) and banded killifish, Fundulus diaphanous (Valley et al. Citation2010). Adult blackspotted topminnow regularly moved among study pools in a dynamic lotic system at rates that exceeded other taxa evaluated (i.e., creek chub, Semotilus atromaculatus, green sunfish, and longear sunfish, Lepomis megalotis), similar to our results for plains topminnow. The observation of large-scale movement behaviors for both species emphasizes the importance of movement to the life history of this family of fishes (Smithson & Johnston Citation1999). Banded killifish also exhibited similar movement patterns to plains topminnow and generally moved greater distances than blackchin shiner, Notropis heterodon, and blacknose shiner, Notropis heterolepis, in a lentic system (Valley et al. Citation2010). Despite traveling greater distances (maximum distance: 1813 m) than plains topminnow, banded killifish exhibited a more limited linear home range likely as a result of uniform habitat distribution in the lentic system relative to the longitudinal habitat mosaic common to lotic environments (Valley et al. Citation2010). Such consistency accentuates the importance of movement behaviors for these short-lived, opportunistic taxa (Dingle Citation1996).
Our investigation revealed multidimensional movement patterns in response to the available habitat complexes at two distinct lotic systems in which experimental populations of marked plains topminnow were introduced. At Sweet Creek, where the extent and location of habitat patches varied considerably, plains topminnow moved more frequently. In contrast, at the South Loup River site, habitats were relatively stable through time and the locations of capture were more consistent. Despite the differences between sites, the movement was regularly responsive to the availability of specific morphological stream features that are the results of lower water velocities. These results support past suggestions of the contributions that habitat heterogeneity has in structuring lotic fish movement patterns and longitudinal distribution (Labbe & Fausch Citation2000; Johnston Citation2000; Scheurer et al. Citation2003; Schmetterling & Adams Citation2004). Thus, plains topminnow movement behaviors are unlikely random, but rather facultative responses to environmental conditions that cease when suitable habitat patches are encountered (Dingle Citation1996; Johnston Citation2000; Kahler et al. Citation2001; Fausch et al. Citation2002; Schmetterling & Adams Citation2004).
Our recapture rates of plains topminnow were low throughout the study duration, potentially affected by sampling inefficiently, mortality of marked individuals, and the probable absence of individuals that migrated from the study area (Fausch et al. Citation2002). The marking process employed in the current study is known to result in limited mortality and has high retention and recognition (Schumann et al. Citation2013). Because we were able to sample a large segment of each system repeatedly, it is unlikely that sampling failed to detect a large number of individuals within the sampling reach. Instead, many individuals potentially moved beyond our sampling reaches. The failure to recapture individuals marked in 2011 in the subsequent year's sampling may be due to the high annual mortality previously reported for the species (Rahel & Thel Citation2004).
The observation of large-scale movement behaviors of plains topminnow identifies the importance of spatial dynamics in fish distribution. Traditional sampling methodologies may be inadequate for defining species’ presence or absence at typical spatial scales (i.e., 100–300 m) that may not reflect the life history of all taxa (Fausch & Young Citation1995; Fausch et al. Citation2002). Recent surveys of plains topminnow throughout its native range described a substantial number of local extirpations (Pasbrig et al. Citation2012). However, attempts to identify range-wide distributions of this species may have underrepresented the spatial extent and number of extant populations, given our results of regular, large-scale movements. Sampling of historically occupied localities resulted in numerous discrete populations in close proximity that likely interact. These conclusions for plains topminnow demonstrate the importance of large-scale spatial patterns of lotic species distribution and conservation efforts.
Species movement behaviors appear to be important for the maintenance and potential recovery of populations of threatened and endangered fish species. Plains topminnow demonstrated consistent patterns of movement and clear associations with select habitat features throughout the riverscape. Large-scale movements maintain connectivity among populations within stream drainage areas (Li et al. Citation2009), reduce the potential for local extinctions, allow recolonization of habitats following extirpation, and have implications for population genetics (Jackson et al. Citation2001; Larson et al. Citation2002; Rahel & Thel Citation2004).
This study of movement behaviors and occupancy of critical habitat patches at multiple scales highlights the importance of embracing the continuous and heterogeneous nature of linear aquatic habitats to understand fish spatial dynamics (Labbe & Fausch Citation2000; Fausch et al. Citation2002; Scheurer et al. Citation2003; Dodds et al. Citation2004). Plains topminnow are highly mobile components of stream ecosystems and their occurrence is regulated by hydrologic variability and habitat features that occur disparately throughout these streams. The heterogeneous nature of plains topminnow occurrence exemplifies the difficulties associated with measurements and predictions of species distributions and further reveals the need to understand the spatial and temporal organization of important habitat patches.
Acknowledgements
Critical comments on our work and suggestions for improvement were made by Katie Bertrand. Special thanks are offered to Gerald Coates, Hal Walker, and Clayton Funk, the staff at the Rock Creek State Fish Hatchery, NE, for providing technical advice and project support with rearing and marking of fish. Assistance with marking, population monitoring, and technical support were provided by Chris Uphoff, Jacob Wirtz, Mike Cavallaro, Seth Lundgren, John Howard, Zachariah Woiak, Rebecca Pawlak, Josh Krietman, Zach Lind, Erik Prenosil, and Jeremy Grauf.
Additional information
Funding
References
- Cross FB, Collins JT. 1995. Fishes in Kansas. 2nd ed. Lawrence (KS): University Press of Kansas.
- Dingle H. 1996. Migration: The biology of life on the move. New York (NY): Oxford University Press.
- Dodds WK, Gido K, Whiles MR, Fritz KM, Matthews WJ. 2004. Life on the edge: the ecology of Great Plains prairie streams. BioScience. 54:205–216.
- Duryea R, Donnelly J, Guthrie D, O'Malley C, Romanowski M, Schmidt R. 1996. Gambusia affinis effectiveness in New Jersey mosquito control. In: Proceedings of the 83rd Meeting of the New Jersey Mosquito Control Association, Inc. New Brunswick (NJ); p. 95–102.
- Fausch KD, Torgersen CE, Baxter CV, Li HW. 2002. Landscapes to riverscapes: bridging the gap between research and conservation of stream fishes. BioScience. 52:483–498.
- Fausch KD, Young MK. 1995. Evolutionarily significant units and movement of resident stream fishes: a cautionary tale. Am Fish Soc Symp. 17:360–370.
- Fischer JR, Paukert CP. 2008. Historical and current environmental influences on an endemic Great Plains fish. Am Midl Nat. 159:364–377.
- Franssen NR, Gido KB, Guy CS, Tripe JA, Shrank SJ, Strakosh TR, Bertrand KN, Franssen CM, Pitts KL, Paukert CP. 2006. Effects of floods on fish assemblages in an intermittent prairie stream. Freshw Biol. 51:2072–2086.
- Gerking SD. 1959. The restricted movement of fish populations. Biol Rev. 34:221–242.
- Gido KB, Bertrand KN, Murdock JN, Dodds WK, Whiles MR. 2010. Disturbance-mediated effects of fishes on stream ecosystem processes: concepts and results from highly variable prairie streams. Am Fish Soc Symp. 73:593–617.
- Gowan C, Fausch KD. 1996. Mobile brook trout in two high-elevation Colorado streams: reevaluating the concept of restricted movement. Can J Fish Aquat Sci. 53:1370–1381.
- Gowan C, Young MK, Fausch KD, Riley SC. 1994. Restricted movement in stream salmonids: a paradigm lost? Can J Fish Aquat Sci. 51:2626–2637.
- Haslouer SG, Eberle ME, Edds DR, Gido KB, Mammoliti CS, Triplett JR, Collins JT, Distler DA, Huggins DG, Stark WJ. 2005. Current status of native fish species in Kansas. Trans Kans Acad Sci. 108:32–46.
- Heggeness J, Northcote TG, Peter A. 1991. Spatial stability of cutthroat trout (Oncorhynchus clarki) in a small, coastal stream. Can J Fish Aquat Sci. 48:757–762.
- Hill J, Grossman GD. 1987. Home range estimates for three North American stream fishes. Copeia. 1987:376–380.
- Hodges SW, Magoulick DD. 2011. Refuge habitats for fishes during seasonal drying in an intermittent stream: movement, survival and abundance of three minnow species. Aquat Sci. 73:513–522.
- Hudy M, Shiflet J. 2009. Movement and recolonization of Potomac sculpin in a Virginia stream. N Am J Fish Manag. 29:196–204.
- Hughes NF. 1999. Populations processes responsible for larger-fish-upstream distribution patterns of Arctic grayling (Thymallus arcticus) in interior Alaskan runoff rivers. Can J Fish Aquat Sci. 56:2292–2299.
- Jackson DA, Peres-Neto PR, Olden JD. 2001. What controls who is where in freshwater fish communities – the roles of biotic, abiotic, and spatial factors. Can J Fish Aquat Sci. 58:157–170.
- Johnston CE. 2000. Movement patterns of imperiled blue shiners (Pisces: Cyprinidae) among habitat patches. Ecol Freshw Fish. 9:170–176.
- Jordan GR, Klumb RA, Wanner GA, Stancill WJ. 2006. Poststocking movements and habitat use of hatchery reared juvenile pallid sturgeon in the Missouri River below Fort Randall Dam, South Dakota and Nebraska. Trans Am Fish Soc. 135:1499–1511.
- Kahler TH, Roni HP, Quinn TP. 2001. Summer movement and growth of juvenile anadromous salmonids in small western Washington streams. Can J Fish Aquat Sci. 58:1947–1956.
- Labbe TR, Fausch KD. 2000. Dynamics of intermittent stream habitat regulate persistence of a threatened fish at multiple scales. Ecol Appl. 10:1774–1791.
- Larson GL, Hoffman RL, Moore SE. 2002. Observations of the distributions of five fish species in small Appalachian stream. Trans Am Fish Soc. 131:791–796.
- Li C, Bessert ML, Macrander J, Ortí G. 2009. Low variation but strong population structure in mitochondrial control region of the plains topminnow, Fundulus sciadicus. J Fish Biol. 74:1037–1048.
- Lonzarich DG, Warren Jr. ML, Lonzarich MR. 1998. Effects of habitat isolation on the recovery of fish assemblages in experimentally defaunated stream pools in Arkansas. Can J Fish Aquat Sci. 55:2141–2149.
- Matthews WJ, Marsh-Matthews E. 2003. Effects of drought on fish across axes of space, time and ecological complexity. Freshw Biol. 8:1232–1253.
- Pasbrig CA, Koupal KD, Schainost S, Hoback WW. 2012. Changes in range-wide distribution of plains topminnow Fundulus sciadicus. Endanger Species Res. 16:235–247.
- Peterson JT, Bayley PB. 1993. Colonization rates of fishes in experimentally defaunated warmwater streams. Trans Am Fish Soc. 122:199–207.
- Pflieger WL. 1997. The fishes of Missouri. Jefferson City (MO): Missouri Department of Conservation.
- Poff NL, Ward JV. 1989. Implications of streamflow variability and predictability for lotic community structure: a regional analysis of streamflow patterns. Can J Fish Aquat Sci. 46:1805–1818.
- Rahel FJ, Thel LA. 2004. Plains Topminnow (Fundulus sciadicus): a technical conservation assessment. Fort Collins (CO): U.S. Department of Agriculture, Forest Service, Rocky Mountain Region.
- Rinne JN, Stefferud JA. 1999. Single versus multiple species management: native fishes in Arizona. For Ecol Manag. 114:357–365.
- Roberts JH, Angermeier PL. 2007. Movement responses of stream fishes to introduced corridors of complex cover. Trans Am Fish Soc. 136:971–978.
- Rodríguez MA. 2002. Restricted movement in stream fish: the paradigm is incomplete, not lost. Ecology. 83:1–13.
- Scheurer JA, Fausch KD, Bestgen KR. 2003. Multiscale processes regulate brassy minnow persistence in a Great Plains river. Trans Am Fish Soc. 132:840–855.
- Schlosser IJ. 1998. Fish recruitment, dispersal, and trophic interactions in a heterogeneous lotic environment. Oecologia. 113:260–268.
- Schlosser IJ, Angermeier PL. 1995. Spatial variation in demographic processes of lotic fishes: conceptual models, empirical evidence, and implication for conservation. Am Fish Soc Symp. 17:392–401.
- Schmetterling DA, Adams SB. 2004. Summer movements with the fish community of a small Montane stream. N Am J Fish Manag. 24:1163–1172.
- Schumann DA, Koupal KD, Hoback WW, Schoenebeck CW. 2013. Evaluation of sprayed fluorescent pigment as a method to mass-mark fish species. Open Fish Sci J. 6:41–47.
- Schumann DA, Pasbrig CA, Koupal KD, Hoback WW. 2012. Culture of plains topminnow in a pond constructed for species conservation. N Am J Aquac. 74:360–364.
- Skalski GT, Gilliam JT. 2000. Modeling diffusive spread in a heterogeneous population: a movement study with stream fish. Ecology. 81:1685–1700.
- Smale MA, Rabeni CF. 1995. Hypoxia and hyperthermia tolerance of headwater stream fishes. Trans Am Fish Soc. 124:698–710.
- Smithson WB, Johnston CE. 1999. Movement patterns of stream fishes in a Ouachita Highlands stream: an examination of the restricted movement paradigm. Trans Am Fish Soc. 128:847–853.
- Taylor CM. 1997. Fish species richness and incidence patterns in isolated and connected stream pools: effects of pool volume and spatial position. Oecologia. 110:560–566.
- Valley RD, Habrat MD, Dibble ED, Drake MT. 2010. Movement patterns and habitat use of three declining littoral fish species in a north-temperate mesotrophic lake. Hydrobiologia. 644:385–399.
- VanArnum CJG, Buynak GL, Ross JR. 2004. Movement of smallmouth bass in Elkhorn Creek, Kentucky. N Am J Fish Manag. 24:311–315.
- Ward DL, Schultz AA, Matson PG. 2003. Differences in swimming ability and behavior in response to high water velocities among native and nonnative fishes. Environ Biol Fish. 68:87–92.