ABSTRACT
Overexploitation of a single target species often results in fish community changes. Commercial and recreational overexploitation of walleye (Sander vitreus) in Shoal Lake, Ontario, in the early 1980s resulted in a ban on walleye fishing. In this study, we summarize potential changes in the catch-per-unit-effort of large-bodied fishes (abundance and biomass in gill nets) and small-bodied fishes (abundance in shoreline seines) for most years in the period 1979–2001. Through our analysis of gill net data, we found that the collapse of walleye was followed by increases in the abundance and biomass of yellow perch (Perca flavescens), cisco (Coregous artedi), and white sucker (Catostomus commersoni). Lake whitefish (Coregonus clupeaformis) also increased following the collapse, but this pattern was confounded by reduction in the lake whitefish commercial harvest. We found no evidence that the collapse of walleye benefited potential competitors (e.g. northern pike, Esox lucius). The collapse also resulted in semi-annual alternations between minnow (Pimaphales spp.) and a combination of yellow perch and shiners (Notropis spp.). Overall, our results are consistent with the general literature showing that the overexploitation of a top predator affects the broader community and with the walleye literature showing that these community shifts tend to reflect a top-down, trophic cascade that stems more from predator–prey relationships than from competitive relationships. Our case study provides important insight into the structure and function of aquatic food webs and the trophic ecology of top predators.
Introduction
Overexploitation of a single target species often leads to changes in the broader fish community. These changes are well documented in marine systems (Baum & Worm Citation2009; Terborgh & Estes Citation2013) and usually inferred from experiments in freshwater systems (e.g. Colby et al. Citation1987; Lyons & Magnuson Citation1987; Carpenter Citation2003; Persson et al. Citation2007). Although evidence for fisheries-induced changes in freshwater systems can be confounded by other factors, examples from around the world include a reduction in the abundance of soft-rayed fishes that was coincident with the recovery of walleye (Sander vitreus) in Lake Erie (Knight & Vondracek Citation1993), an increase in the abundance of small-bodied fishes following the sustained removal of large-bodied fishes from the Oueme River, West Africa (Allan et al. Citation2005), and an increase in small cyprinids following the overexploitation of catfishes and carps in Cambodia's Tonle Sap River (Allan et al. Citation2005). Documented examples of fisheries-induced changes in freshwater communities are important for testing the hypothesis that the removal of one or more large predators can result in top-down effects that are directly or indirectly linked to multiple trophic levels and that fish harvesting induces responses that are consistent with experimental evidence for trophic cascades (Carpenter et al. Citation1985; Drenner & Hambright Citation2002).
The collapse of the walleye fishery in Shoal Lake, Ontario, Canada is an opportunity to examine a fisheries-induced trophic cascade involving a popular freshwater game fish. Shoal Lake is a large (25,856 ha), mesotrophic lake in northwestern Ontario. Commercial and recreational overexploitation of walleye brought this species to the verge of collapse in the early 1980s and resulted in a moratorium on sport and commercial fishing for walleye in 1983 (Bolton Citation2012). In other systems, it has been suggested that managing solely for walleye changes fish community composition (Knight & Vondracek Citation1993). Changes may include a top-down effect by evidence of increased prey abundance, such as yellow perch (Perca flavescens), or the dominance of a new top predator, such as northern pike (Esox lucius; Jacobson & Anderson Citation2007). Survey data from Shoal Lake before and after the decline of walleye suggest concomitant changes in community structure; however, these patterns are difficult to discern using bivariate approaches (Supplemental Figures 1–3).
In this study, we used redundancy analysis (RDA) to summarize community data that were too complex to analyze using bivariate techniques (Gauch Citation1982). RDA is a multivariate approach that is particularly appropriate for fisheries data because it helps to infer changes in relative abundance (or biomass) over time (Van den Brink & Ter Braak Citation1999) without suffering from the ‘arch’ or ‘horseshoe’ effects that are common with other multivariate techniques (Legendre & Legendre Citation2012). Here, we used RDA to describe the response of both large- and small-bodied fish communities in Shoal Lake to the commercial and recreational overexploitation of walleye. We defined large-bodied fishes as those that were captured in gill nets and small-bodied fishes as those that were captured in shoreline seines. The latter included forage fishes as well as juveniles of some large-bodies species (e.g. yellow perch). The goal of our study was to assess and document changes in the Shoal Lake fish community following the collapse of walleye.
Methods
Netting data
We used data from two long-term netting surveys that were conducted by the Ontario Ministry of Natural Resources Lake of the Woods Fisheries Assessment Unit to generate three ‘species × year’ data matrices: the abundance (number/net) and biomass (kg/net) of large-bodied fishes, and the abundance (number/net) of small-bodied fishes. For large-bodied abundance and biomass, we used data from gill net surveys for the years 1979–1988, 1993, 1998, 2000, and 2001. Four of these surveys (1979–1982) took place prior to the closure of the fishery in 1983; the remaining 10 surveys took place on or after 1983. For the years 1979–1988 and 1993, data were from fall (mid-August–October) index surveys that consisted of 4–38 overnight (20–24 hours) sets of multifilament, multi-mesh gill nets. Nets were 122 m long and 1.8 m deep with 8, 15.2-m panels randomly graduated in stretched mesh size from 3.8 to 12.7 cm in 1.3-cm increments. For the years 1998, 2000, and 2001, data were from fall walleye index netting (FWIN) surveys that consisted of 20–60 overnight (7–25 hours) sets of monofilament, multi-mesh gill nets in September to October (Morgan Citation2002). FWIN nets were 60 m long and 1.8 m deep with 8, 7.5-m panels that graduated sequentially in stretched mesh size from 2.5 to 15.2 cm (Morgan Citation2002). Finally, we generated a matrix of small-bodied fish abundance from shoreline seine net surveys for the years 1980–1997, and 1999. Seine nets were 30.5 m long and 2.4 m deep, and deployed at up to 10 sites/month from June to August (mean 22, range 8–44 seines/year).
Statistical analyses
We focused our multivariate analyses on those species that, on average, made up >0.1% of total annual abundance. This common-species approach reduced both the number of variables (species) relative to observations (sample years) and the amount of redundant information in each matrix (Grossman et al. Citation1991; Marchant Citation1999, Citation2002). We then focused our analysis on the eight common species in the 18-species dataset (Supplemental Table A1). For the small-bodied fishes, we first grouped certain species by genus (Notropis, shiners; Pimephales, minnows), Subfamily (Etheostomatinae, darters; Leuciscinae, dace), or Family (Centrarchidae, sunfishes; Gasterosteidae, sticklebacks). Grouping was necessary because the identification of small-bodied fishes to species was not always possible or may have been inaccurate for closely related species. We then focused our analysis on the 10 common taxa from the 16-taxa dataset (Supplemental Table A2).
We used RDA to test for changes in the abundance and biomass of large-bodied fishes and the abundance of small-bodied fishes. RDA is a constrained ordination method that allows us to use our explanatory variable (year) to model our response variables (species abundance). To minimize the influence of very abundant species, we applied a Hellinger transformation to the species data (i.e. abundance or biomass). We did not transform years so that results could be interpreted in terms of community changes over time. The RDA applies a series of multiple linear regressions, followed by a principal component analysis (PCA) on fitted values of species abundance to generate the length of our first axis, RDA1, and then a PCA on the residuals of the fitted values to generate the length of our second axis, PC1 (Legendre and Legendre Citation2012). We conducted all analyses using R version 3.0.2 (R Core Team Citation2015) and version 2.0-10 of the R package vegan (Oksanen et al. Citation2013) at a level of significance of α = 0.05.
Results
According to RDA, the abundance of large-bodied fishes in gill net samples varied significantly among years ( and sum of all canonical variables = 0.298; F = 10.00, p = 0.001 for all canonical axes). The first and second axes explain 45.5% and 28.9% of the variance, respectively (). Walleye dominated the sample during the pre-collapse period 1979–1982 (). This was followed by a relative decrease of walleye from 1983 to 1985. Northern pike showed a relative increase in 1987, but the community shifted to yellow perch, cisco (Coregonus artedi), and white sucker (Catostomus commersoni) in samples from 1988 and 1993, and included lake whitefish (Coregonus clupeaformis) in the final three samples (1998, 2000, and 2001).
Figure 1. The distribution of sample years in two-dimensional species-space as determined by RDA of abundance (number/net) of common, large-bodied fishes in gill net surveys of Shoal Lake, Ontario, 1979–1988, 1993, 1998, 2000, and 2001. A close association between species and year suggests there was a relatively high increase in the abundance of that species in that year. CIS = cisco (Coregonus artedi), LWF = lake whitefish (Coregonus clupeaformis), NPI = northern pike (Esox lucius), RBA = rock bass (Amblopites rupestris), SMB = smallmouth bass (Micropterus dolomieu), WAL = walleye (Sander vitreus), WSU = white sucker (Catostomus commersoni), YPE = yellow perch (Perca flavescens).
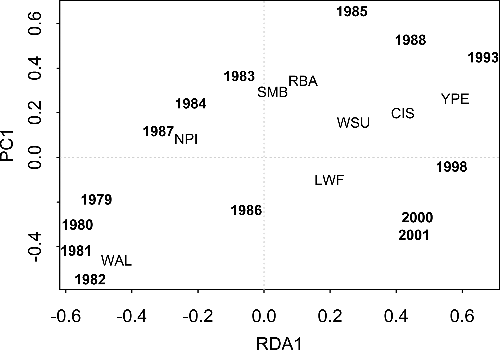
Table 1. Results of the redundancy analysis of the abundance and biomass of 8 fish species captured in gill nets (14 years in the period 1979–2001) and the abundance of 10 species captured in shoreline seine nets (19 years in the period 1980–1999) in Shoal Lake, Ontario.
The biomass of large-bodied fishes in gill net samples also varied significantly among years ( and sum of all canonical variables = 0.130; F = 4.86, p = 0.01 for all canonical axes). The first and second axes explain 28.8% and 47.4% of the variance, respectively (). Patterns of large-bodied biomass and abundance were similar (). One exception was northern pike, which showed a relative increase in biomass in 1983; this increase was not evident in the abundance RDA until 1987. These results suggest that the sample contained few large pike in 1983, and then many small pike in 1987. Another exception was the 1988 sample, which showed a relative increase in white sucker biomass while yellow perch and cisco increased in abundance.
Figure 2. The distribution of sample years in two-dimensional species-space as determined by RDA of biomass (kg/net) of common, large-bodied fishes in gill net surveys of Shoal Lake, Ontario, 1979–1988, 1993, 1998, 2000, and 2001. A close association between species and year suggests there was a relatively high increase in the biomass of that species in that year. Species identification is the same as in
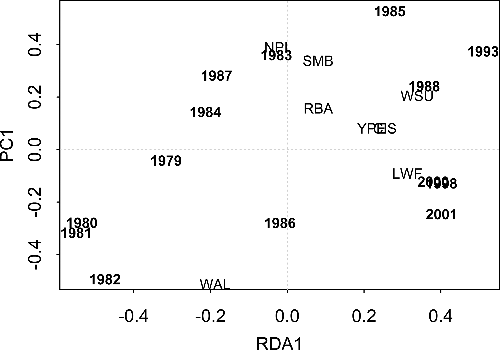
According to RDA, the abundance of small-bodied fishes in shoreline seine nets varied significantly among years (, sum of all canonical variables = 0.078; F = 3.88, p = 0.02 for all canonical axes). The first and second axes explain 18.56% and 54.17% of the variance, respectively (). Samples from the period 1980–1982 suggested that minnows (Pimephales spp.) were relatively common (). The 1983 sample showed a relative decrease in minnows and increase in yellow perch, while 1984 showed an increase in stickleback (Gasterosteidae) and, to a lesser extent, Centrarchids. From 1985 onward, the RDA identified alternations among three taxa – minnows, yellow perch, and shiners (Notropis spp.). Yellow perch showed a relative increase in 1985 and 1986, then minnows in 1987 and 1988. With the exception of 1999, all other years in this period alternated between yellow perch and shiners.
Figure 3. The distribution of sample years in two-dimensional species-space as determined by RDA of abundance (number/net) of common, small-bodied fishes in seine net surveys of Shoal Lake, Ontario, 1980–1997, and 1999. The forward slash separate overlapping years. A close association between species and year suggests there was a relatively high increase in the abundance of that species in that year. CEN = centrarchids, CHU = lake chub (Couesius plumbeus), DAR = non-logperch darters (Etheostomatinae), LPE = logperch (Percina caprodes), MIN = minnows (Pimephales spp.), SBA = sticklebacks (Gasterosteidae), SCU = mottled sculpin (Cottus bairdii), SHI = shiners (Notropis spp.), SMB = smallmouth bass (Micropterus dolomieu), YPE = yellow perch (Perca flavescens).
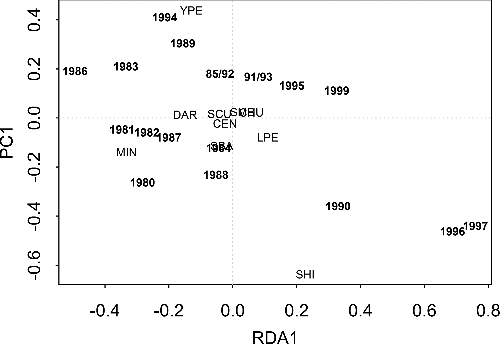
Discussion
The collapse of walleye in Shoal Lake in the early 1980s forced the closure of the fishery and resulted in decades-long changes in community structure. Our RDA of large-bodied fishes suggested that the Shoal Lake community showed relative increases in yellow perch, cisco, white sucker, and lake whitefish after 1987 (five years after collapse). The increase in the abundance of large yellow perch was preceded by an increase in the abundance of small yellow perch in the 1985 and 1986 seine samples. Given that yellow perch are a principal diet item for walleye (Nielsen Citation1980), the dominance of small yellow perch in 1985 and 1986 (and large perch starting in 1988) was likely due to lower predation following the collapse of walleye in 1983 (Forney Citation1974; Bertolo & Magnan Citation2005; Ivan et al. Citation2011).
The relative increase of cisco and white sucker in the large-bodied sample may have also resulted from reduced walleye predation. Kaufman et al. (Citation2006) suggest that cisco biomass comprises up to 70% of the diet of large walleye (>37.5 cm), even in the presence of yellow perch. White sucker in the large-bodied sample increased (albeit to a lesser extent) in the same years as yellow perch and cisco. In Wilson Lake, Minnesota, the removal of 85% of white sucker biomass resulted in a one-third increase in walleye biomass (Johnson Citation1977). This result implies an inverse relationship between white suckers and walleye biomass, which may stem from competition for food (Johnson Citation1977) or an alternative steady state (Persson et al. Citation2007).
Less clear is the mechanism for the relative increase in lake whitefish at the end of the study period. Age-1+ walleye prefer small, soft-rayed fishes as prey (Knight & Vondracek Citation1993), so it is possible that lake whitefish experienced a release from predation. However, this interpretation is confounded by the implementation of commercial lake whitefish quotas in the early 1980s (Bolton Citation2012). These quotas curtailed harvest and may have contributed to increased whitefish abundance. Similarly, the apparent increase in lake whitefish in 1998, 2000, and 2001 might reflect an increase in the vulnerability of this species to the FWIN protocol, which was adopted in 1998.
We did not find evidence that northern pike replaced walleye as the top predator in Shoal Lake, despite evidence in other systems (e.g. Nate et al. Citation2003). Northern pike abundance and biomass remained relatively consistent during the period of interest. Two exceptions were relative increases of biomass in 1983 (i.e. relatively large pike in that year) and abundance in 1987 (i.e. relatively small pike in that year), which may be indicative of a recruitment pulse in response to an increase in the availability of forage. However, it does not appear that northern pike benefited from the walleye collapse in the long term. Consistent with walleye removal experiments (Colby et al. Citation1987), the absence of a northern pike response to walleye collapse may suggest minimal competition between the two species in Shoal Lake.
The RDA of the abundance of small-bodied fishes also showed shifts in the community following the collapse of walleye. Pimephales spp. showed a relative increase prior to the walleye collapse, which eventually shifted to small yellow perch. Minnow abundance in Sparkling Lake, Wisconsin, was not significantly impacted by walleye predation (Lyons & Magnuson Citation1987), but was reduced by higher abundances of yellow perch (Lyons Citation1987). Although it has been suggested that young-of-the-year (YOY) yellow perch buffer other forage species such as minnows from walleye predation (Forney Citation1974; Lyons & Magnuson Citation1987), YOY yellow perch at high abundance may reduce minnow abundance through the consumption of eggs and larvae (Lyons Citation1987). The consumption of minnows by YOY perch may explain why minnows in Shoal Lake showed a decrease in relative abundance after 1982 (i.e. post-collapse).
We can only speculate as to why YOY yellow perch and shiners were out of phase after walleye collapsed. The increase in both species probably resulted from reduced predation by age-1+ walleye (Knight & Vondracek Citation1993). However, Knight et al. (Citation1984) described yellow perch as opportunistic feeders that can alternate between zooplankton and small fish, including shiners. Given also that yellow perch become piscivorous at age-1 (Pelham et al. Citation2001), a strong yellow perch year class soon after the Shoal Lake walleye collapse may have temporarily established a classic boom and bust predator–prey dynamic (Krebs et al. Citation2001).
Although our results are consistent with the history of exploitation on Shoal Lake and the literature, we highlight three caveats to our results. First, our analysis of large-bodied fishes combined data from two netting protocols: FWIN (1998, 2000, 2001) and index netting (all other years). The years associated with FWIN sampling stand out as having relatively high lake whitefish abundance and biomass (although yellow perch, cisco, and white sucker remained high). However, this pattern persisted when we re-analyzed using FWIN data that were converted to index data via species-specific regression equations from nearby Rainy Lake, Ontario (McLeod et al. Citation2004). Although it is possible that these conversions were inadequate or inappropriate for our system, an alternative explanation is that the changes in the Shoal Lake fish community overwhelmed any difference in gear selectivity between gill netting protocols. Second, gill net data were limited to only four years pre-collapse (1979–1982) and discontinuous (three gaps, two of which spanned five years). Limited data before the collapse prevented us from inferring community dynamics during this period, and from detecting longer term trends that may have persisted after (albeit independent of) the collapse. Similarly, gaps in the gill net data after the collapse probably obscured finer scale dynamics of yellow perch, shiners, and other species. Finally, data were unavailable to test the hypothesis that the Shoal Lake community was also responding to environmental gradients (e.g. temperature change, water clarity) at the local or regional scale. Although samples sizes were relatively low in some years (e.g. 4 and 8 gill net samples in 1988 and 1993, respectively), removing these years did not affect our findings (Venturelli, unpublished results).
Our results contribute to the limited studies on community effects following walleye removal in a large, temperate lake that is not subject to stocking, invasive species, and other disturbances. Overall, our findings are consistent with other studies involving fish community changes (e.g. Colby et al. Citation1987; He & Kitchell Citation1990; Knight & Vondracek Citation1993). The overexploitation of walleye in Shoal Lake was a ‘natural’ experiment that, similar to walleye removal experiments in smaller systems (Colby et al. Citation1987), had a cascading effect on the overall fish community. Specifically, the removal of a top predator (walleye) resulted in relative increases in prey species (e.g. yellow perch, cisco, etc.), led to changes in the relative abundance of other large-bodied fishes (e.g. lake whitefish, white sucker), and may have caused yellow perch and shiners to oscillate out of phase. Our observations support the hypothesis that predatory fishes play a significant role in the assemblage of forage fish (i.e. fewer yellow perch and cisco in the presence of high walleye abundance), and that the removal of a large, predatory fish often leads to a broader changes to the fish community (Carpenter et al. Citation1985; Colby et al. Citation1987; Lyons & Magnuson Citation1987; He & Kitchell Citation1990; Allan et al. Citation2005; Baum & Worm Citation2009). These changes are also an important reminder to managers that fisheries collapses can affect communities in ways that are unpredictable and unfavorable (Link Citation2002; Daskalov et al. Citation2007; Altieri et al. Citation2012) and that capture fisheries can be slow to recover from exploitation (NeuBauer et al. Citation2013). More broadly, this case study provides important insight into the structure and function in large-lake food webs (Shurin et al. Citation2006), and the trophic ecology of top predators (Sergio et al. Citation2014).
Acknowledgments
We are grateful to the many employees and volunteers of the Ontario Ministry of Natural Resources that collected and archived the data that were used in this study. Sapna Sharma, Kory Vue, and Steve Walker helped with statistics. Pete Addison, Jay Hatch, Don Jackson, Jenni McDermid, Ray Newman, Mark Poesch, Jessica Ward, and two anonymous reviewers provided valuable comments during the development of this manuscript.
Disclosure statement
The authors have no financial interest or benefit arising from the direct applications of this research.
Additional information
Funding
References
- Allan JD, Abell R, Hogan ZEB, Revenga C, Taylor BW, Welcomme RL, Winemiller K. 2005. Overfishing of inland waters. BioScience. 55:1041–1051.
- Altieri AH, Bertness MD, Coverdale TC, Herrmann NC, Angelini C. 2012. A trophic cascade triggers collapse of a salt‐marsh ecosystem with intensive recreational fishing. Ecology. 93:1402–1410.
- Baum JK, Worm B. 2009. Cascading top‐down effects of changing oceanic predator abundances. J Anim Ecol. 78:699–714.
- Bertolo A, Magnan P. 2005. The relationship between piscivory and growth of white sucker (Catostomus commersoni) and yellow perch (Perca flavescens) in headwater lakes of the Canadian Shield. Can J Fish Aquat Sci. 62:2706–2715.
- Bolton R. 2012. “Being out on the lake”: Iskatewizaagegan Anishinaabeg perspectives on contemporary fishing practice and well-being [master thesis]. Winnipeg: University of Manitoba.
- Carpenter SR. 2003. Regime shifts in lake ecosystems: pattern and variation. Luhe: International Ecology Institute.
- Carpenter SR, Kitchell JF, Hodgson JR. 1985. Cascading trophic interactions and lake productivity. BioScience. 35:634–639.
- Colby PJ, Ryan PA, Schupp DH, Serns SL. 1987. Interactions in north-temperate lake fish communities. Can J Fish Aquat Sci. 44:s104–s128.
- Daskalov GM, Grishin AN, Rodionov S, Mihneva V. 2007. Trophic cascades triggered by overfishing reveal possible mechanisms of ecosystem regime shifts. Proc Natl Acad Sci USA. 104:10518–10523.
- Drenner RW, Hambright RKD. 2002. Piscivores, trophic cascades, and lake management. ScientificWorldJournal. 2:284–307.
- Forney JL. 1974. Interactions between yellow perch abundance, walleye predation, and survival of alternate prey in Oneida Lake, New York. Trans Am Fish Soc. 103:15–24.
- Gauch HG Jr. 1982. Multivariate analysis and community structure. New York (NY): Cambridge University Press.
- Grossman GD, Nickerson DM, Freeman MC. 1991. Principal component analysis of assemblage structure data: utility of tests based on eigenvalues. Ecology. 72:41–347.
- He X, Kitchell JF. 1990. Direct and indirect effects of predation on a fish community: a whole-lake experiment. Trans Am Fish Soc. 119:825–835.
- Ivan LN, Höök TO, Thomas MV, Fielder DG. 2011. Long-term and interannual dynamics of walleye and yellow perch in Saginaw Bay, Lake Huron. Trans Am Fish Soc. 140:1078–1092.
- Jacobson PC, Anderson CS. 2007. Optimal stocking densities of walleye fingerlings in Minnesota lakes. N Am J Fish Manage. 27:650–658.
- Johnson FH. 1977. Responses of walleye (Stizostedion vitreum vitreum) and yellow perch (Perca flavescens) populations to removal of white sucker (Catostomus commersoni) from a Minnesota Lake, 1966. J Fish Res Board Can. 34:1633–1642.
- Kaufman SD, Gunn JM, Morgan GE, Couture P. 2006. Muscle enzymes reveal walleye (Sander vitreus) are less active when larger prey (cisco, Coregonus artedi) are present. Can J Fish Aquat Sci. 63:970–979.
- Knight RL, Margraf FJ, Carline RF. 1984. Piscivory by walleyes and yellow perch in western Lake Erie. Trans Am Fish Soc. 113:677–693.
- Knight RL, Vondracek B. 1993. Changes in prey fish populations in western Lake Erie, 1969–88, as related to walleye, Stizostedion vitreum, predation. Can J Fish Aquat Sci. 50:1289–1298.
- Krebs CJ, Boonstra R, Boutin S, Sinclair AR. 2001. What drives the 10-year Cycle of Snowshoe Hares? The ten-year cycle of snowshoe hares—one of the most striking features of the boreal forest—is a product of the interaction between predation and food supplies, as large-scale experiments in the Yukon have demonstrated. Bioscience. 51:25–35.
- Link JS. 2002. Ecological considerations in fisheries management: when does it matter? Fisheries. 27:10–17.
- Legendre P, Legendre L. 2012. Numerical ecology. 2nd English ed. Amsterdam: Elsevier.
- Lyons J. 1987. Distribution, abundance, and mortality of small littoral-zone fishes in Sparkling Lake, Wisconsin. Environ Biol Fish. 18:93–107.
- Lyons J, Magnuson JJ. 1987. Effects of walleye predation on the population dynamics of small littoral-zone fishes in a northern Wisconsin lake. Trans Am Fish Soc. 116:29–39.
- Marchant R. 1999. How important are rare species in aquatic community ecology and bioassessment? A comment on the conclusions of Cao et al. 1999. Limnol Oceanogr. 44:1840–1841.
- Marchant R. 2002. Do rare species have any place in multivariate analysis for bioassessment? J N Am Benthol Soc. 21:311–313.
- McLeod D, Taillon D, Rhodenzier A. 2004. A comparison of fall walleye index netting (FWIN) and long-term netting techniques in Rainy Lake, Ontario. Fort Frances: Ontario Ministry of Natural Resources. ( Fort Frances District report series no. 63).
- Morgan GE. 2002. Manual of instructions – Fall Walleye Index Netting (FWIN). Peterborough: Ontario Ministry of Natural Resources, Percid Community Synthesis, Diagnostics and Sampling Standards Working Group.
- Nate NA, Bozek MA, Hansen MJ, Ramm CW, Bremigan MT, Hewett SW. 2003. Predicting the occurrence and success of walleye populations from physical and biological features of northern Wisconsin lakes. N Am J Fish Manage. 23:1207–1214.
- Neubauer P, Jensen OP, Hutchings JA, Baum JK. 2013. Resilience and recovery of overexploited marine populations. Science. 340:347–349.
- Nielsen LA. 1980. Effect of walleye (Stizostedion vitreum vitreum) predation on juvenile mortality and recruitment of yellow perch (Perca flavescens) in Oneida Lake, New York. Can J Fish Aquat Sci. 37:11–19.
- Oksanen J, Blanchet FG, Kindt R, Legendre P, Minchin PR, O'Hara RB, Simpson GL, Solymos P, Stevens MHH, Wagner H. 2013. Vegan: community ecology package. R package version 2.0-10; [cited 2015 May 11]. Available from: http://CRAN.R-project.org/package=vegan
- Pelham ME, Pierce CL, Larscheid JG. 2001. Diet dynamics of the juvenile piscivorous fish community in Spirit Lake, Iowa, USA, 1997–1998. Ecol Freshw Fish. 10:198–211.
- Persson L, Amundsen PA, De Roos AM, Klemetsen A, Knudsen R, Primicerio R. 2007. Culling prey promotes predator recovery—alternative states in a whole-lake experiment. Science. 316:1743–1746.
- R Development Core Team. 2015. R: a language and environment for statistical computing. Vienna: R Foundation for Statistical Computing; [cited 2015 May 11]. Available from: http://www.R-project.org
- Sergio F, Schmitz OJ, Krebs CJ, Holt RD, Heithaus MR, Wirsing AJ, Ripple WJ, Ritchie E, Ainley D, Oro D, et al. 2014. Towards a cohesive, holistic view of top predation: a definition, synthesis and perspective. Oikos. 123:1234–1243.
- Shurin JB, Gruner DS, Hillebrand H. 2006. All wet or dried up? Real differences between aquatic and terrestrial food webs. Proc Biol Sci. 273:1–9.
- Terborgh J, Estes JA, editors. 2013. Trophic cascades: predators, prey, and the changing dynamics of nature. Washington (DC): Island Press.
- Van den Brink PJ, Ter Braak CJ. 1999. Principal response curves: analysis of time‐dependent multivariate responses of biological community to stress. Environ Toxicol Chem. 18:138–148.