ABSTRACT
Phenology of fish spawning in lotic ecosystems depends on interactions between species life history strategies and patterns of environmental variation related to hydrology and seasonality. To further study these relationships, we examined patterns of larval fish abundance in relation to discharge and other environmental variables in the Brazos and Trinity rivers, Texas. From March 2013 to March 2014, environmental data and larval fishes were collected twice each month using drift nets. Multivariate analyses indicated that, at both study sites, water temperature was the primary mechanism driving temporal and taxonomic variation in larval fish assemblage structure at the family level. However, in the Trinity River, assemblage structure was significantly associated with discharge, albeit to a lesser degree than with water temperature. Cyprinid protolarvae dominated catches in both rivers and did not seem to be constrained by any aspect of the flow regime, as evidenced by their high abundance throughout the reproductive season (April–September). Analysis of larval fish abundance at the family level compromised our ability to infer spawning dynamics of species classified as fluvial specialists. Further research enabling identification of larvae to species-level is needed to elucidate how flow regime components influence reproduction and recruitment of fluvial specialists.
Introduction
The most influential environmental factor affecting fish community structure in lotic ecosystems is flow regime (Poff et al. Citation1997; Grossman et al. Citation1998, Citation2010). Research on the functional traits of fishes in rivers has demonstrated relationships between hydrological regime and predominant life history strategy (Mims & Olden Citation2012). For example, species with a periodic life history strategy (large bodied, delayed maturation, high fecundity, episodic recruitment) dominate in seasonal environments, such as large rivers in the tropics and those at high latitudes that receive spring snow melt (Winemiller & Rose Citation1992; Olden & Kennard Citation2010; Winemiller Citation2010). In rivers with unpredictable discharge, such as those of the Great Plains of the United States, opportunistic species (small-bodied, early maturation, continuous reproduction, low survivorship) tend to dominate (Olden & Kennard Citation2010; Winemiller Citation2010; Mims & Olden Citation2012). Relationships between fish life history strategies and gradients of hydrologic variability reveal inter-continental convergence (Olden & Kennard Citation2010), suggesting that general hydrological mechanisms may structure fish species assemblages in rivers.
To further assess these relationships, we examined hydrology and temporal patterns of larval fish abundance in lowland rivers of the northwestern coastal plain of the Gulf of Mexico. Hydrology influences multiple mechanisms that can influence local assemblages of larval fish. The low flow hypothesis (Humphries et al. Citation1999) proposes that many fish species invest greater reproductive effort during periods of low flow so that larvae can inhabit marginal pools and other slack-water habitats with relatively high temperatures and abundant larval food resources (Humphries et al. Citation1999) that promote growth and development (Moore Citation1944; Platania & Altenbach Citation1998; Humphries et al. Citation1999). Rapid development enhances larval fish survival, because larger size is associated with better swimming ability, which is critical for avoiding predation and capturing prey (Fuiman Citation1989; Bone et al. Citation1995; Mann & Bass Citation1997). During this susceptible period, mortality from starvation is believed to be high because poor swimming ability prevents larval fishes from efficiently capturing appropriately sized prey items (Humphries et al. Citation1999). Additionally, during low flow conditions, larval fish may have an advantage in capturing prey because resource densities are higher (Humphries et al. Citation1999). Conversely, low flow conditions can increase larval mortality if habitat reduction results in greater food resource competition and risks of predation, or environmental quality declines within isolated habitats due to low concentrations of dissolved oxygen and high water temperatures that challenge physiological tolerances (Harvey Citation1991; Copp Citation1992; Humphries et al. Citation1999).
In direct contrast to the low flow hypothesis, the flood pulse concept maintains that fish reproduction is greater during periods of increased discharge. Flood pulses may provide spawning cues and floodplain inundation creates temporary but productive aquatic habitats for spawning and larval growth and survival (Junk et al. Citation1989). As flood pulses recede, they leave behind shallow, low-velocity habitats that often are ‘hot spots’ for aquatic primary production (Bowen et al. Citation2003; Hoagstrom & Turner Citation2013). Spawning during flood pulses allows larvae and their predators to disperse at low densities within a greatly expanded volume of aquatic habitat, which should reduce both predation mortality and competition for food resources (Hoagstrom & Turner Citation2013). Relative benefits of a flow pulse for successful reproduction depend on pulse duration and timing (Finger & Stewart Citation1987). In the Great Plains of the United States, the relationship between flow pulses and spawning synchronicity has been documented for several minnow species (family Cyprinidae; Durham & Wilde Citation2008, Citation2014).
Prior research on the lower Brazos River in Texas determined that spatial and temporal drift patterns of larval fish were nonrandom. Comparison of larval fish catches between day and night revealed the dominate reproductive guilds present in the lower Brazos River (substrate spawners, pelagic broadcast spawners and adhesive broadcast spawners) exhibited higher drift densities at night and in near shore areas (Williams Citation2011). Greater density of drifting larval fish at night is a general phenomenon in rivers worldwide (Reeves & Galat Citation2010). Additionally, based on two years of study, larval fish densities were higher during a wet year when daily mean flows were at the 94th percentile of historical daily mean average (during the first year, flow was at the 41st percentile). Williams’ (Citation2011) findings, therefore, suggest that fish reproduction in the lower Brazos River conforms to the flood pulse concept. The objective for this study were to examine patterns of larval fish abundance in relation to environmental variables, particularly discharge, to infer mechanisms structuring larval fish assemblages in the Brazos and Trinity rivers within the coastal plains of the northwestern Gulf of Mexico.
Methods
Study locations
Surveys were conducted at one site on the lower Brazos River near State Highway 105 west of Navasota, Brazos County, Texas (30° 21' 45.1" N, 96° 09' 16.8" W) and one site on the middle Trinity River near State Highway 7, west of Crockett, Leon County, Texas (31° 19' 08.6" N, 95° 40' 07.8" W; ). Study sites were chosen based on presence of point sandbars that facilitated the setting of drift nets.
Figure 1. Map showing Brazos and Trinity River survey sites. Upstream dots are locations of USGS gages; downstream dots are survey sites.
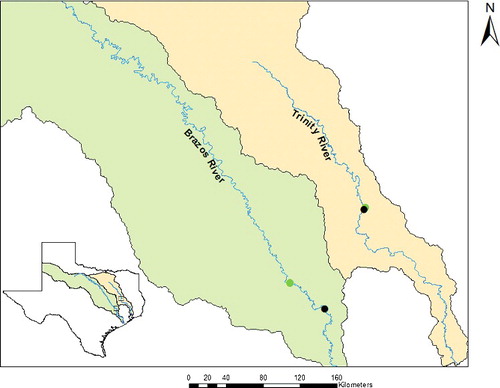
The Brazos River is the third largest river in Texas. Originating in New Mexico, the Brazos flows southeast approximately 2736 km and discharges into the Gulf of Mexico at Freeport, Texas. The Brazos River mainstem and tributaries have been altered by the construction of 16 reservoirs for flood control and water supply (Brazos River Bay and Basin Expert Science Team [Brazos BBEST Citation2012]). The lower Brazos River extends 330 km from Waco, Texas, to the Gulf of Mexico. This reach is unimpounded, but flows are regulated by upstream and tributary dams. Historical discharge records indicate that highest flows generally occur during winter and spring (Zeug & Winemiller Citation2008); however, rainstorms can generate high flow pulses and overbanking flows during any month. The Brazos River has high dissolved nutrient concentrations, moderate pH and high turbidity associated with suspended fine sediments during and after periods of high discharge (Roach & Winemiller Citation2011).
The Trinity River is another large river in Texas characterized by high turbidity. The Trinity River originates west of Fort Worth, Texas, and extends 563 km to the Gulf of Mexico (Phillips et al. Citation2004); its basin is approximately 47,000 km2 (Trinity and San Jacinto and Galveston Bay River Basin and Bay Expert Science Team [Trinity BBEST Citation2009]). The Trinity has 31 reservoirs and numerous water diversions for agricultural and municipal uses that have altered the flow regime (Trinity BBEST Citation2009). Much like the Brazos, the Trinity has a hydrologic regime driven by relatively unpredictable precipitation events.
Field surveys
From March 2013 to March 2014, larval fish surveys were generally conducted in each river every other week (two surveys per month for each river) from March through October during the peak fish reproductive season, alternating weeks between the Brazos and Trinity rivers. The winter period that is outside of the known reproductive season for most fish species (November–February) was surveyed once per month at each site. Drift nets (rectangular opening 0.45 m wide by 0.30 m tall and length of 1 m with 500 μm mesh) were placed on gently sloping sandbars at wadable depths within current (Williams Citation2011). Three net arrays (each with three nets) were set in a straight line perpendicular to the shoreline. Water depth (cm) and current velocity (m/s) were measured at the center of each drift net opening at the beginning and end of each sampling interval using a meter stick and Marsh-McBirney current velocity meter (Flo-Mate Model 2000, Frederick, MD, USA), respectively. Drift net samples were standardized as catch-per-unit effort (CPUE) according to total volume of water filtered. This volume was calculated as the product of mean current velocity in each net (m/s), net area (m2; width × height of the submerged portion of net) and duration of sampling (s). Based on prior research on diel drift patterns of larval fish (Reeves & Galat Citation2010; Williams Citation2011), drift net surveys were conducted at night and near shore to exploit higher larval fish drift densities. Drift net samples were collected every 2 h, with the first sample collected at 2000 h and the last sample collected at 0200 h (yielding a total of 36 individual drift net samples on each date). From November to February, sampling effort was reduced, with the last collections initiated at 2200 h (yielding a total of 18 individual drift net samples). After each 2-h interval, samples from each drift net were washed into individual Whirl-Pak® bags containing 95% ethanol and then transported to the lab at Texas A&M for processing. Water temperature (°C) was measured on each date at each survey site using a multi-parameter water quality sonde (Model 65, YSI Inc., http://www.ysi.com) and water transparency (cm) was measured using a Secchi disk.
Streamflow data collection
Daily discharge data were obtained from the nearest upstream US Geological Survey (USGS) streamflow gage. Discharge data for the Brazos River came from USGS 08108700 ‘Brazos Rv at SH21 near Bryan, TX, USA’, and USGS 08065350 ‘Trinity Rv near Crockett, TX, USA’ was used for the Trinity River. USGS 08108700 is located approximately 92 (river) km upstream of our Brazos River site. USGS 08065350 is located approximately 4 (river) km upstream of our Trinity River site. Maximum daily discharge values were used for the Brazos River. Maximum daily discharge values were not provided for the Trinity River; therefore, mean daily discharge values were used.
Laboratory methods
Larval fish were separated from other contents of the Whirl-Paks® and then counted and stored in labeled vials in 70% ethanol. Larval fish were sorted by developmental stage prior to taxonomic identification. Identifications proceeded from the juvenile stage and progressed through successively earlier stages. Developmental stages were categorized as protolarvae, mesolarvae, metalarvae or juveniles based on characteristics reported by Snyder (Citation1998). Our analyses focused on the earliest stage, protolarvae, because this stage generally is only a few days old and best reflects spawning events. Protolarvae are characterized by a complete absence of fin spines and rays as well as pelvic fin buds (Snyder Citation1998). Larvae still maintaining their yolk sacs were counted with protolarvae (). To date, there are no published keys that enable species-level identification of protolarvae of Cyprinidae from the southern United States. Since cyprinids were by far the most abundant taxon in our samples, larval identification was carried to family level based primarily on Auer (Citation1982), Fuiman et al. (Citation1983), and Snyder and Seal (Citation2008). Very large drift net samples from one date at the Trinity River required us to estimate total larval abundance based on subsamples. After 500 individuals had been identified at the family level, these counts were extrapolated to estimate total abundances. In all other cases, individual larvae were identified and counted.
Statistical analysis
Canonical correspondence analysis (CCA) was used to ordinate protolarvae samples based on taxonomic structure and environmental variables, and to evaluate associations between assemblage structural and environmental gradients. Environmental variables included in this analysis were total discharge, coefficient of variation (CV) of discharge (%), maximum increase of discharge (%), maximum decrease of discharge (%), water temperature (°C) and water transparency (cm). Total discharge, CV of discharge, maximum increase of discharge and maximum decrease of discharge were calculated over a six-day period preceding the sampling date. A lag period of six days was chosen as the interval most likely to produce protolarvae. Moore (Citation1944) and Bottrell et al. (Citation1964) estimated that cyprinid eggs hatch in 1–2 days, and development of the gas bladder occurs in about four days (Dudley & Platania Citation2007). Formation of the gas bladder enhances the larva's ability to move vertically and horizontally and, thereby, may influence entry into drift nets (Platania & Altenbach Citation1998). Total discharge was the total water volume passing the upstream gage station during the six-day period. Each daily discharge cubic feet per second (cfs) value was multiplied by 86,400 (number of seconds in one day), and these values were then summed across the six-day interval to yield total discharge. Discharge CV was calculated by dividing the standard deviation of the daily discharge during the six-day interval by the mean of the daily discharge during the same period and multiplying by 100. This variable gives an indication of the variation in discharge around the mean (i.e. rate of change), but does not indicate whether the overall trend was increasing or decreasing. Maximum increase of discharge was the largest positive percent change in maximum daily discharge relative to the previous day's discharge during the six-day interval. Similarly, the maximum decrease of discharge was the largest negative percent change in maximum daily discharge relative to the previous day's discharge during the six-day interval. Water temperature and transparency were measured and recorded on each survey date. A Monte Carlo permutation test (999 permutations) was used to determine statistical significance of environmental variables and constrained ordination axes (p ≤ 0.05). For both rivers, sampling dates from March through September 2013 plus one sampling date in March 2014 were included in the CCA. Dates from October to February were excluded because of the rarity of protolarvae in drift net samples. Only the first two canonical axes from the CCA (dominant axes) were interpreted. Protolarvae abundance in drift net samples was standardized as CPUE (# protolarvae per 100 m3). Prior to analysis, protolarvae CPUE values were log(x + 1) transformed, and rare families (present in <10% of survey dates) were removed to reduce disproportionate effects of rare species (Legendre & Legendre Citation1998). Multivariate ordinations and Monte Carlo permutation tests were performed using the Vegan package in R (Oksanen et al. Citation2015).
Results
Brazos River larval fish assemblage
Within Brazos River drift net samples, 9767 protolarvae were identified from seven families, with Cyprinidae the dominant taxon (7814 specimens; ∼80% of the total sample). The next most abundant family was Centrarchidae (1011; ∼10%) followed by Clupeidae (800; ∼8%), and Catostomidae (110; ∼1%). Atherinopsidae, Moronidae and Percidae were the other families represented in Brazos drift net samples, with each accounting for <1% of the total catch.
Percid, catostomid, clupeid and moronid protolarvae were captured during early spring (beginning 8 March 2013). Larvae from these families, as well as one centrarchid larva, were also present in the 25 March 2014 sample. Protolarvae from these families were uncommon or absent from samples after May. Four atherinopsid protolarvae were present in the 20 April 2013 sample. Centrarchid protolarvae were first captured in late April 2013, but most centrarchid protolarvae were captured from July to September. Cyprinid protolarvae first appeared in the 29 March 2013 sample (absent in the 25 March 2014 sample). On all but two survey dates (8 March 2013 and 25 March 2014), Cyprinidae was the most abundant taxon (). CPUE of cyprinid protolarvae exhibited a bimodal distribution with a spring peak in late April and early May, and a summer peak in late July and early August. The first peak of cyprinid protolarvae occurred during a period of relatively stable flows early in the reproductive season (20 April 2013 and 4 May 2013). Large magnitude flow pulses in May and June were not associated with higher cyprinid CPUE, and 16 May and 10 June had some of the lowest CPUE values. A peak in cyprinid protolarvae abundance occurred on 24 July 2013, shortly after a pronounced flow pulse. Protolarvae CPUE was high during the two surveys following 24 July 2013, a period that had relatively stable flows ().
Figure 3. Temporal distribution of protolarvae composition collected from the Brazos River between March 2013 and October 2013, plus an additional sampling date in March 2014. Total sample size for each month is as follows: March, n = 259; April, n = 2211; May, n = 2561; June, n = 142; July, n = 1623; August, n = 2383; September, n = 587; October, n = 1.
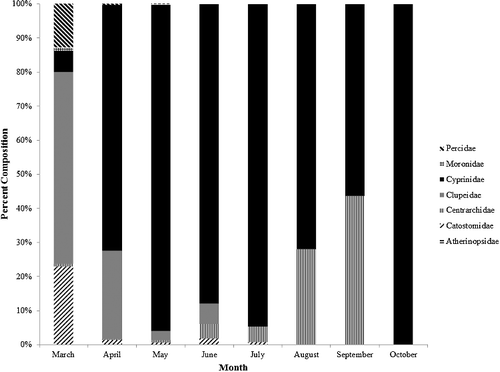
Trinity River larval fish assemblage
From Trinity River drift net samples, 10,119 protolarvae were identified from six families. Cyprinids dominated the sample (9844 specimens; ∼97% of the total sample), followed by Percidae (215; ∼2%). Catostomidae, Centrarchidae, Clupeidae and Ictaluridae each accounted for <1% of the total sample.
Percid and catostomid protolarvae were the only taxa sampled on the first survey date, (11 March 2013). Percid, catostomid and clupeid protolarvae were most abundant in early spring samples. Four ictalurid protolarvae were collected during May. Cyprinid protolarvae were not collected until 1 May 2013; however, only one date (6 April) was surveyed during the month of April in 2013. One cyprinid protolarvae and 90 percid protolarvae were captured on 20 March 2014. Cyprinid protolarvae CPUE in the Trinity peaked on 18 August 2013. Except for sampling dates in March and April, Cyprinidae was the most abundant taxon in each sample. Centrarchid protolarvae were not captured until July and had a minor peak (19 specimens) on 18 August 2013 (). From 21 July 2013 to 16 August 2013, the Trinity River was in either subsistence or base flow conditions, and a flow pulse was initiated on 17 August 2013. Three smaller peaks occurred on 8 May, 14 June and 31 July, but no pattern in cyprinid protolarvae CPUE was discernable in relation to hydrology during this period ().
Figure 5. Temporal distribution of protolarvae composition collected from the Trinity River between March 2013 and October 2013, plus an additional sampling date in March 2014. Total sample size for each month is as follows: March, n = 31; April, n = 88; May, n = 2128; June, n = 716; July, n = 1308; August, n = 5635; September, n = 111; October, n = 13.
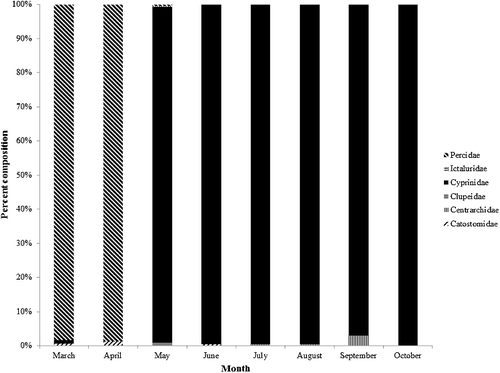
Ordination of Brazos River protolarvae CPUE and environmental data
CCA axis 1 (CCA1) described a water temperature gradient, with low temperatures associated with negative scores and higher temperatures represented by larger positive scores. CCA1 alone explained 76.2% of the variation in this plot. CCA2 explained an additional 16.9% of total variation, and described a gradient associated with water transparency. CCA1 was the only statistically significant axis (Monte Carlo permutation test, n = 999, F = 8.56, p = 0.002), and temperature was the only environmental variable with a statistically significant relationship (Monte Carlo permutation test, n = 999, F = 6.94, p = 0.004) with larval fish assemblage structure at the family level. The 8 March 2013 sample had a large negative loading on CCA1 (−3.91) and a high positive loading on CCA2 (5.56). This outlier was not included in the ordination graph to allow better visualization of remaining survey dates. The 8 March 2013 sample differed from nearly all other samples by having no cyprinid protolarvae. Ordination of Brazos samples revealed three distinct groupings based largely on seasons: early spring (March and April), early to mid-summer (4 May through 8 August) and late summer (21 August through 18 September; ).
Figure 7. CCA ordination plot of Brazos River larval fish samples (protolarvae CPUE at the family level). Points are survey dates; vectors depict loadings for environmental variables. 8 March 2013 was included in the analysis, but was not plotted on the graph as explained in the text. Cat, Catostomidae; Cen, Centrarchidae; Clu, Clupeidae; Cyp, Cyprinidae; Mor, Moronidae; Per, Percidae.
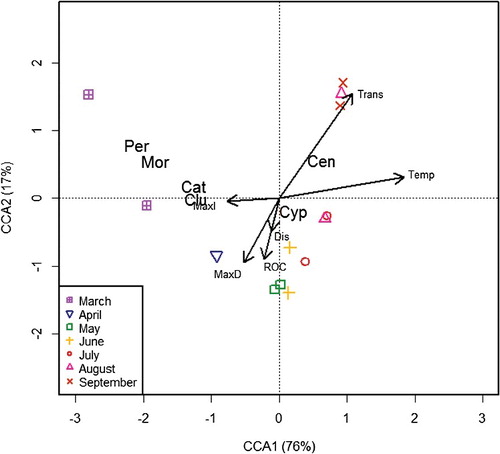
Catostomidae, Clupeidae, Moronidae and Percidae all had large negative loadings on CCA1, indicating that protolarvae of these species were most abundant during early spring. Centrarchidae had a high positive loading on CCA1 and CCA2, indicating that most centrarchid protolarvae were produced during summer. Percidae and Centrarchidae had large positive loadings on CCA2, indicating that the protolarvae were collected on survey dates when discharge tended to be low, fluctuation in discharge was low and water transparency was high. Cyprinid protolarvae were not strongly correlated with either CCA1 or CCA2 (), probably because cyprinid larvae were present in samples from late spring until mid-fall ().
Table 1. Summary statistics for axes 1 and 2 of CCA with the Brazos River dataset.
Ordination of Trinity River protolarvae CPUE and environmental data
Ordination of samples on the first two axes from CCA for the Trinity dataset revealed a pattern similar to that obtained for the Brazos. CCA1 (modeling 88.6% of total variation) reveals a general water temperature gradient, with higher water temperatures represented by higher positive scores. Positive scores on CCA2 were associated with greater transparency and higher temperature, and negative scores on CCA2 were associated with greater declines in mean discharge (max decrease) and higher discharge volumes. Both CCA1 and CCA2 were statistically significant axes (Monte Carlo permutation test, n = 999, F = 45.9, p = 0.001 and F = 3.8, p = 0.03, respectively); however, CCA2 only explained an additional 7.2% of variation in the dataset. Temperature and discharge had statistically significant relationships (Monte Carlo permutation test, n = 999, F = 32.3, p = 0.001 and F = 4.9, p = 0.045, respectively) between larval fish family assemblage structure and environmental variables.
Like the Brazos larval fish assemblage, the Trinity assemblage revealed seasonal shifts in structure (). Species loadings on CCA axes indicate that Percidae and Catostomidae spawned during spring when the water temperature was low. Clupeidae was less associated with low water temperatures in the Trinity compared to the Brazos. Protolarvae CPUE data indicated that spawning by Clupeidae was confined to early spring. Centrarchidae had the highest positive scores on CCA1 and CCA2, indicating that peak centrarchid spawning was during the middle and latter parts of summer when water turbidity was low. Cyprinid larvae in the Trinity River were not strongly associated with either CCA axis because, similar to the Brazos findings, cyprinid protolarvae generally were abundant throughout the year (). Clupeidae and Ictaluridae had negative scores on CCA2, indicating that their protolarvae were most abundant when discharge was high and declining (i.e. descending limb of a flow pulse). Larvae of these two families were not captured after May in 2013 ().
Figure 8. CCA ordination plot of Trinity River larval fish samples (protolarvae CPUE at the family level). Points are survey dates; vectors depict loadings for environmental variables. Cat, Catostomidae; Cen, Centrarchidae; Clu, Clupeidae; Cyp, Cyprinidae; Ict, Ictaluridae; Per, Percidae.
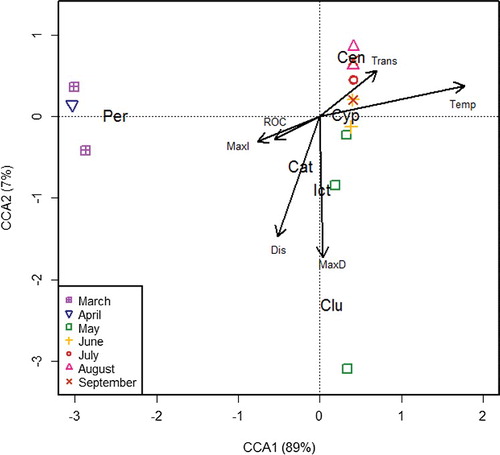
Table 2. Summary statistics for axes 1 and 2 of CCA with the Trinity River dataset.
Discussion
Drift net surveys conducted over a full year captured fish protolarvae from seven families in the Brazos River and six families in the Trinity River. Cyprinid larvae comprised 80% and 97% of the collective samples from the Brazos and Trinity, respectively, and small cyprinids constituted over 94% of the collective sample from seine surveys conducted from littoral habitats on each survey date at each survey site. Other fish families revealed greater between-river variation in relative abundance. Trinity samples tended to have more percid larvae and fewer clupeid and centrarchid larvae than Brazos samples. Total catches of centrarchid protolarvae were higher in the Brazos, probably due to low flows during summer 2013 that led to uncharacteristically clear water and abundant macrophyte growth, which are conducive to centrarchid nesting, brood care and recruitment.
Analysis of larval abundance at the family level compromised our ability to infer spawning dynamics of species classified as fluvial specialists, those with reproductive activity strongly associated with flow pulses. Species-rich families, such as Cyprinidae, probably were represented by fishes with divergent reproductive tactics. Several cyprinid species found in both the Brazos and Trinity rivers belong to a reproductive guild that broadcast spawns non-adhesive, semi-buoyant eggs within the water column. Many species in this guild have experienced population and range reductions in rivers of the Great Plains and arid southwest due to habitat fragmentation, alteration of the natural flow regimes and water withdrawals (Luttrell et al. Citation1999; Bonner & Wilde Citation2000; Hoagstrom et al. Citation2011; Perkin & Gido Citation2011). Because we were unable to identify protolarvae to species, it was not possible to discern whether or not flow pulses were associated with synchronized spawning by fluvial specialist cyprinids as reported for species in the upper Brazos River based on study of adult reproductive ecology (Durham & Wilde Citation2008, Citation2014). As taxonomic research on early life stages advances in the future, species-level identification should reveal interspecific variation in cyprinid protolarvae abundance in relation to discharge.
Life history variation within Cyprinidae probably yields differential responses to environmental conditions and success of spawning and recruitment. For example, red shiner (Cyprinella lutrensis) probably conforms to the low flow hypothesis, whereas, Macrhybopsis spp. (reported members of the broadcast spawning guild) should conform to the flood pulse hypothesis (Platania & Altenbach Citation1998; Durham & Wilde Citation2006; Wilde & Durham Citation2008). Our samples of cyprinid protolarvae probably were dominated by red shiner and bullhead minnow (Pimephales vigilax), two extremely common minnows that are not members of the reproductive guild that relies on flow pulses for successful recruitment. Red shiner and bullhead minnow together accounted for 88% and 78% of juvenile and adult fishes captured with seines from littoral habitats in the Brazos and Trinity River. Both species possess demersal, adhesive eggs that are deposited in crevices (Holland-Bartels et al. Citation1990; Durham & Wilde Citation2006). This reproductive strategy is well suited for early life stage survival under low flow or stable-flow conditions; therefore, a positive relationship between increased or fluctuating discharge and reproductive effort would not be expected for these species (Platania & Altenbach Citation1998; Durham & Wilde Citation2006). Although data for later larval stages are not reported here, we note that metalarval and juvenile cyprinids in drift net samples were very abundant on 18 August 2013 in the Trinity River. This survey date also had the highest CPUE of cyprinid protolarvae. High abundance of different developmental stages on the same date indicates sequential bouts of reproduction and high larval survival during preceding weeks. The hydrograph preceding this survey date was characterized by prolonged subsistence and base flow conditions.
Temporal patterns of centrarchid larval abundance conformed with the low flow hypothesis (Schlosser Citation1985). Centrarchid protolarvae CPUE was positively correlated with water temperature and transparency, conditions observed in both rivers during late summer when flows were low. In contrast, other families, such as Percidae (e.g. Percina and Etheostoma spp.), apparently spawned under a wide range of hydrological conditions, but were strongly seasonal (abundance correlated with water temperature).
Prior research on the lower Brazos River (Williams Citation2011) documented greater densities of drifting larval fish during a wet year with elevated discharge compared to a dry year with low flows. This finding suggests that fishes of Texas Gulf Coast rivers conform to the flood pulse concept, whereby, greater discharge yields greater fish recruitment (Junk et al. Citation1989). However, the wet year had higher densities of metalarvae and juveniles, and densities of protolarvae and mesolarvae were similar between years. Williams’ (Citation2011) finding suggests that higher discharge does not have a large influence on spawning activity, but it may contribute to higher larval survival rates. Neither the low flow nor flood pulse hypothesis can entirely explain the relationship between discharge and protolarvae abundance in the Brazos and Trinity rivers, because these rivers have fish species with divergent life history strategies.
At both study sites, water temperature was statistically significant in explaining temporal and taxonomic variation in larval fish assemblage structure. In the CCA, water temperature alone explained 38% and 61% of variation defined by relationships between larval fish assemblages and environmental gradients in the Brazos and Trinity, respectively. This suggests that seasonality is the primary factor influencing spawning activity, with temperature or day length serving as a reliable indicator of seasons in this region (Fuiman et al. Citation1983; Holland-Bartels et al. Citation1990). Whereas centrarchid protolarvae were most abundant during late summer, a few specimens were captured earlier in the reproductive season. This may be explained by seasonal variations within the Centrarchidae family. Largemouth bass (Micropterus salmoides), spotted bass (Micropterus punctulatus) and orangespotted sunfish (Lepomis humilis) are reported to spawn as early as April (Holland-Bartels et al. Citation1990; Wallus & Simon 2008). Green sunfish (Lepomis cyanellus), bluegill sunfish (Lepomis macrochirus), white crappie (Pomoxis annularis) and longear sunfish (Lepomis megalotis) are reported to spawn later in the spring and during early summer (Holland-Bartels et al. Citation1990; Wallus & Simon 2008). Small cyprinids are known to have extended reproductive seasons, and this was confirmed by abundant protolarvae from spring until fall.
Larval fish assemblage structure in the Brazos River was not significantly associated with any of the hydrological variables entered in the CCA; however, in the Trinity River, discharge was significantly associated with larval fish assemblage structure, albeit to a lesser degree than water temperature (discharge, by itself, explained 9% of the variation in the Trinity dataset, and water temperature explained 61%). This difference could be due to the fact that the Trinity experienced higher flows, especially during spring which coincided with spawning by percids and certain species from other families. Centrarchids spawned during summer when water temperatures were high and discharge generally was low. We hypothesize that much like the pattern found in the Brazos, seasonality is the principal mechanism determining larval fish assemblage structure at the family level in the Trinity River. However, species-level surveys across multiple years with varying hydrological conditions would be required to elucidate relationships between spawning activity and hydrology. Unfortunately, the basis for species-level identification of protolarvae is lacking for most Gulf Coast fishes. For the time being, species-level studies of spawning phenology will have to rely on analysis of gonad developmental stages, oocytes and juvenile otoliths to estimate hatch dates (e.g. Durham & Wilde Citation2006, Citation2008, Citation2014). Nonetheless, patterns of protolarval abundance in the Brazos and Trinity rivers reveal strong seasonal patterns and a potential influence of hydrology on certain taxa, providing a foundation for future research on fish population dynamics in Gulf Coast rivers.
Acknowledgments
We thank C. Montana, C. Krabbenhoft, D. Lay, A. Vigneaux, M. Lisbon, S. Angel, C. Rivera, D. Saenz, L. Bower, D. Fitzgerald, A. Medellin, K. Mahavadi, N. Smolensky and D. Kim for assistance with field collections and/or laboratory work. We thank Dr K. Conway for assistance in larval cyprinid identification and L. Kleinsasser for study design and contract assistance. Partial funding for this project was through the Texas State Wildlife Grants program grant T-60-1 in cooperation with the U.S. Fish and Wildlife Service, Wildlife and Sport Fish Restoration Program. Additional financial support was provided by the Texas Water Resources Institute through a Mills Scholarship.
Disclosure statement
No potential conflict of interest was reported by the authors.
Additional information
Funding
Notes on contributors
Anthony W. Rodger
Anthony W. Rodger is currently a stream biologist with the Oklahoma Department of Wildlife Conservation. He received a B.S. in Wildlife and Fisheries Sciences from South Dakota State University in 2013 and a M.S. in Fisheries Sciences from Texas A&M University in 2015. He has broad interests in aquatic, stream fish and community ecology. He currently manages stream fish populations and communities across the state of Oklahoma.
Kevin B. Mayes
Kevin B. Mayes is an aquatic biologist with the Texas Parks and Wildlife Department, Inland Fisheries, River Studies Program. He received a B.S. in Biology from Oklahoma State University in 1987 and a M.S. in Aquatic Biology from Southwest Texas State University in 1991. He currently studies water quality and quantity needs across Texas and coordinates the Department's efforts in the implementation of the Texas Instream Flow Program. He provides technical guidance and expertise in evaluation of native fishes conservation, water development, and hydropower projects.
Kirk O. Winemiller
Kirk O. Winemiller received his PhD from the University of Texas in 1987. He is interested in evolutionary and community ecology, with most research centered on fish trophic ecology, life histories, functional traits and habitat associations, as well as food webs of rivers and estuaries. He currently is researching fish assemblage structure and dynamics in temperate and tropical rivers, and human impacts on hydrology, biodiversity and fisheries.
References
- Auer NA. 1982. Identification of larval fishes of the Great Lakes basin with emphasis on the Lake Michigan drainage. Ann Arbor (MI): Great Lakes Fishery Commission. ( Special Publication 82-3 ).
- Bone Q, Marshall NB, Blaxter JHS. 1995. Biology of fishes. 2nd ed. London: Chapman and Hall.
- Bonner TH, Wilde GR. 2000. Changes in the Canadian River fish assemblage associated with reservoir construction. J Freshw Ecol. 15:189–198.
- Bottrell CE, Ingersol RH, Jones RW. 1964. Notes on the embryology, early development, and behavior of Hybopsis aestivalis tetranemus (Gilbert). Trans Am Microsc Soc. 83:391–399.
- Bowen ZH, Bovee KD, Waddle TJ. 2003. Effects of flow regulation on shallow-water habitat dynamics and floodplain connectivity. Trans Am Fish Soc. 132:809–823.
- [Brazos BBEST] Brazos River Basin and Bay Expert Science Team. 2012. Brazos River basin and bay expert science team environmental flow regime recommendations report. Final submission to the Brazos River Basin and Bay Area Stakeholder Committee. Austin (TX): Environmental Flows Advisory Group and the Texas Commission on Environmental Quality.
- Copp GH. 1992. Comparative microhabitat use of cyprinid larvae and juveniles in a lotic floodplain channel. Environ Biol Fishes. 33:181–193.
- Dudley RK, Platania SP. 2007. Flow regulation and fragmentation imperil pelagic-spawning riverine fishes. Ecol Appl. 17:2074–2086.
- Durham BW, Wilde GR. 2006. Influence of stream discharge on reproductive success of a prairie stream fish assemblage. Trans Am Fish Soc. 135:1644–1653.
- Durham BW, Wilde GR. 2008. Asynchronous and synchronous spawning by smalleye shiner Notropis buccula from the Brazos River, Texas. Ecol Freshw Fish. 17:528–541.
- Durham BW, Wilde GR. 2014. Understanding complex reproductive ecology in fishes: the importance of individual and population-scale information. Aquat Ecol. 48:91–106.
- Finger TR, Stewart EM. 1987. Response of fishes to flooding regime in lowland hardwood wetlands. In: Matthews WJ, Heins DC, editors. Community and evolutionary ecology of North American stream fishes. Norman (OK): University Oklahoma Press; p. 86–92.
- Fuiman LA. 1989. Vulnerability of Atlantic herring larvae to predation by yearling herring. Mar Ecol Prog Ser. 51:291–299.
- Fuiman LA, Conner JV, Lathrop BF, Buynak GL, Snyder DE, Loos JJ. 1983. State of the art identification for cyprinid fish larvae from eastern North America. Trans Am Fish Soc. 112:319–332.
- Grossman GD, Farr MD, Wagner CM, Petty JT. 2010. Why there are fewer fish upstream. In: Gido KB, Jackson DA, editors. Community ecology of stream fishes: concepts, approaches, and techniques. Bethesda (MD): American Fisheries Society; p. 63–81.
- Grossman GD, Ratajczak RE, Crawford M, Freeman MC. 1998. Assemblage organization in stream fishes: effects of environmental variation and interspecific interactions. Ecol Monogr. 68:395–420.
- Harvey BC. 1991. Interaction of abiotic and biotic factors influences larval fish survival in an Oklahoma stream. Can J Fish Aquat Sci. 48:1476–1480.
- Hoagstrom CW, Brooks JE, Davenport SR. 2011. A large-scale conservation perspective considering endemic fishes of the North American plains. Biol Conserv. 144:21–34.
- Hoagstrom CW, Turner TF. 2013. Recruitment ecology of pelagic-broadcast spawning minnows: paradigms from the ocean advance science and conservation of an imperilled freshwater fauna. Fish Fish. 16:282–299.
- Holland-Bartels LE, Littlejohn SK, Huston ML. 1990. A guide to the larval fishes of the upper Mississippi River. La Crosse (WI): University of Minnesota Extension Press.
- Humphries P, King AJ, Koehn JD. 1999. Fish, flows and flood plains: links between freshwater fishes and their environment in the Murray-Darling River system, Australia. Environ Biol Fishes. 56:129–151.
- Junk W, Bayley P, Sparks R. 1989. The flood pulse concept in river-floodpain systems. In: Dodge D, editor. Proceedings of the International Large River Symposium. Canadian Special Publication of Fisheries and Aquatic Sciences 106. p. 110–127.
- Legendre P, Legendre L. 1998. Numerical ecology. Amsterdam: Elsevier Science.
- Luttrell GR, Echelle AA, Fisher WL, Eisenhour DJ. 1999. Declining status of two species of the Macrhybopsis aestivalis complex (Teleostei: Cyprinidae) in the Arkansas River basin and related effects of reservoirs as barriers to dispersal. Copeia. 1999:981–989.
- Mann RHK, Bass JAB. 1997. The critical water velocities of larval roach (Rutilus rutilus) and dace (Leuciscus leuciscus) and implications for river management. Regul Rivers: Res Manage. 13:295–301.
- Mims MC, Olden JD. 2012. Life history theory predicts fish assemblage response to hydrologic regimes. Ecology. 93:35–45.
- Moore GA. 1944. Notes on the early life history of Notropis girardi. Copeia. 1944:209–214.
- Oksanen J, Blanchet FG, Kindt R, Legendre P, Minchin PR, O'hara RB, Simpson GL, Solymos P, Henry M, Stevens H, Wagner H. 2015. Vegan: community ecology package. R package version 2.2-1. Available from: http://CRAN.R-project.org/package=vegan.
- Olden JD, Kennard MJ. 2010. Intercontinental comparison of fish life history strategies along a gradient of hydrologic variability. In: Gido KB, Jackson DA, editors. Community ecology of stream fishes: concepts, approaches, and techniques. Bethesda (MD): American Fisheries Society; p. 83–108.
- Perkin JS, Gido KB. 2011. Stream fragmentation thresholds for a reproductive guild of Great Plains fishes. Fisheries. 36:371–383.
- Phillips JD, Slattery MC, Musselman ZA. 2004. Dam-to-delta sediment inputs and storage in the lower Trinity River, Texas. Geomorphology. 62:17–34.
- Platania SP, Altenbach CS. 1998. Reproductive strategies and egg types of seven Rio Grande basin cyprinids. Copeia. 1998:559–569.
- Poff NL, Allan DJ, Bain MB, Karr JR, Prestegaard KL, Richter BD, Sparks RE, Stromberg JC. 1997. The natural flow regime: a paradigm for river conservation and restoration. Bioscience. 47:769–784.
- Reeves KS, Galat DL. 2010. Do larval fishes exhibit diel drift patterns in a large, turbid river? Appl Ichthyol. 26:571–577.
- Roach KA, Winemiller KO. 2011. Diel turnover of assemblages of fish and shrimp on sandbanks in a temperate floodplain river. Trans Am Fish Soc. 140:84–90.
- Schlosser IJ. 1985. Flow regime, juvenile abundance, and the assemblage structure of stream fishes. Ecology. 66:1484–1490.
- Snyder DE. 1998. Catostomid larvae and early juveniles of the Rio Grande basin in Colorado. Final Report of Colorado State University Larval Fish Laboratory to Colorado Division of Wildlife. Monte Vista (CO): Larval Fish Laboratory, Colorado State University.
- Snyder DE, Seal SC. 2008. Computer interactive key to families of larval fishes in freshwaters of the United States and Canada (data set for use with DELTA Intkey). Fort Collins (CO):Larval Fish Laboratory, Colorado State University.
- [Trinity BBEST] Trinity and San Jacinto and Galveston Bay River Basin and Bay Expert Science Team. 2009. Basin and bay expert science team environmental flows recommendations report. Final submission to the Trinity and San Jacinto Rivers and Galveston Bay Basin and Bay Area Stakeholder Committee. Austin (TX): Environmental Flows Advisory Group and Texas Commission on Environmental Quality.
- Wallus R, Simon TP. 2008. Reproductive biology and early life history of fishes in the Ohio River Drainage. Volume 6: Elassomatidae and Centrarchidae. Boca Raton (FL): CRC Press.
- Wilde GR, Durham BW. 2008. A life history model for peppered chub, a broadcast-spawning cyprinid. Trans Am Fish Soc. 137:1657–1666.
- Williams CS. 2011. Life history characteristics of three obligate riverine species and drift patterns of lower Brazos River fishes [dissertation]. San Marcos (TS):Texas State University.
- Winemiller KO. 2010. Stream fish communities from patch dynamics to intercontinental convergences. In: Gido KB, Jackson DA, editors. Community ecology of stream fishes: concepts, approaches, and techniques. Bethesda (MD):American Fisheries Society; p. 23–28.
- Winemiller KO, Rose KA. 1992. Patterns of life-history diversification in North American fishes: implications for population regulation. Can J Fish Aquat Sci. 49:2196–2218.
- Zeug SC, Winemiller KO. 2008. Evidence supporting the importance of terrestrial carbon in a large-river food web. Ecology. 89:1733–1743.