ABSTRACT
The suspended algal fraction in small lotic waterbodies has rarely been investigated as the ecological importance was questioned due to low abundances. Recent findings suggest, that substantial riverine plankton communities can establish in small lotic waterbodies. The gap of knowledge concerning the composition of suspended algal assemblages and influential factors determining their composition is addressed in this study by monitoring suspended and attached algal communities over a two year period in agriculturally influenced small lotic waterbodies using delayed fluorescence spectroscopy. A quantitative comparison of suspended and attached algae indicated, that suspended algae exceeded attached algal biomass in late summer and early autumn. Redundancy analyses and corresponding generalized linear models indicated that the suspended algal groups were most influenced by nutrients, temperature and velocity, whereas light regime was of minor importance. The attached algal groups were affected by the same factors, however with differing intensity. Regional factors, such as season, were identified as the major gradients along which both algal fractions varied, inducing temporal synchronous algal assemblages. This work represents a contribution towards a better understanding of algal ecology specifically suspended algae in small lotic waterbodies.
Introduction
Algae occur ubiquitously in high abundances throughout aquatic ecosystems. They are highly successful primary producers in stream habitats, especially in streams with low allochthonous input such as in agriculturally influenced streams and ditches (Simon & Travis Citation2011). In these habitats, the primary energy source for higher trophic levels, besides macrophytes, is algae (Stevenson Citation1996; Hötzel & Croome Citation1999). Algae are also critical components of small lotic waterbodies in that they participate in biogeochemical cycles; for example, participating in fluxes or transformations of nitrogen and phosphate (Nienhuis Citation1993; Lamberti Citation1996; Hamilton et al. Citation2001).
Attached, benthic algae, which are morphologically adapted to shear stress, play a key role in primary production in small streams and ditches (Nienhuis Citation1993; Lamberti Citation1996). In contrast, planktonic algae are more commonly found in lentic and slow-flowing waterbodies where a longer retention time allows for substantial growth within the water column (Reynolds & Descy Citation1996; Leland et al. Citation2001; Piirsoo et al. Citation2008). In small lotic waterbodies, free-floating algae (hereafter defined as suspended algae) are thought to originate from attached benthic algae in a dynamic interchange or to come from upstream lentic waterbodies rather than being truly riverine phytoplankton (Hötzel & Croome Citation1999; Bellinger & Sigee Citation2010). According to the river continuum concept, suspended algae in small lotic waterbodies are considered to be negligible contributors to primary production because of low retention times (Vannote et al. Citation1980). Contradicting this concept, however, Wu et al. (Citation2011) found that some algal species do develop substantial in situ planktonic populations in small lotic waterbodies which are relevant to the primary production and food web processes occurring in the systems. Because these findings are so recent, very few studies on suspended algae in small lotic waterbodies have been conducted so far. The result is a lack of knowledge concerning suspended algae community structure and their ecological relevance in these ecosystems. Hence, it is unknown whether the same environmental factors affect both algal fractions (suspended and attached) similarly.
Previous studies on planktonic algae in larger streams and rivers and on attached algae in streams and ditches have shown that temporal and spatial algal distribution, abundance and community composition are primarily affected by regional, large-scale factors such as climate, geology and land use (Munn et al. Citation2002; Black et al. Citation2011). These regional influences result in spatial and seasonal synchronous algal assemblage (Andrus et al. Citation2013). However, small-scale factors are crucial for short-term dynamics and are thus important for short-term temporal scales (Biggs & Gerbeaux Citation1993). For example, nutrients, as a small-scale factor, were identified as major influential factors on algal community composition and abundance in lotic waterbodies (Carpenter et al. Citation1998; EPA Citation1998; Biggs Citation2000). Aside from nutrients, other small-scale factors such as water temperature (Wu et al. Citation2011), light (influenced by canopy cover and turbidity; Munn et al. Citation1989), hydraulic disturbances, flow regime (Biggs Citation1995; Leland Citation2003) and herbivory (Rosemond et al. Citation2000; Dodds et al. Citation2002) have been found to considerably affect attached algal composition and abundance. However, a general understanding of which factors regulate suspended algal composition in small lotic waterbodies has yet to be established and it is unclear whether the same environmental and structural factors affect both suspended and attached algae within the same aquatic ecosystem (Basu & Pick Citation1995; Piirsoo et al. Citation2008; Wu et al. Citation2011).
In this study, three small streams and two ditches in central Germany were analyzed over a period of two years with the objectives: (1) to examine whether suspended algal fractions are, in terms of primary productivity, of importance and whether they differ from the attached algal fractions in species composition in small lotic waterbodies; (2) to identify the structural (morphological) and environmental factors which influence suspended algal composition in small lotic waterbodies; (3) to determine whether the same factors, and to what extent these factors, influence suspended and attached algal composition; (4) to verify the hypothesis of Munn et al. (Citation2002) and Black et al. (Citation2011) who assumed that regional factors superimpose local factors in the study area.
Methods
Study area and sampling sites
The study was conducted at five lotic waterbodies with 10 sampling sites in the Vogelsberg region in central Germany (Middle Hesse) from 2011 to 2013. The sampling sites were in three streams, Klein (K1, K2, K3), Haverbach (H) and Rulfbach (R1, R2), and two ditches, Ditch A (A1, A2) and Ditch B (B1, B2; ). A detailed description of the waterbodies is provided by Breuer et al. (Citation2016). No lentic waterbodies were connected to the investigated streams and ditches. Sites investigated represent typical waterbodies in the middle Hessen region regarding stream classifications (HLUG Citation2006b; ).
Table 1. Selected environmental and structural variables and algal data with the corresponding seasonal mean ± SE for ditches (n = 99) and streams (n = 269).
Algae sampling and analysis
Suspended and attached algae were sampled at 10 sites with differing sampling regimes. Samples from streams Klein (K1, K2, K3) and Haverbach (H) were taken at weekly intervals between April 2011 and July 2011 and from then on at two-week intervals until April 2013. Ditch A (A1, A2), Ditch B (B1, B2) and Rulfbach (R1, R2) were sampled from March 2012 to April 2013.
Width-integrated samples of suspended algae were collected over a lateral transect following Shelton (Citation1994). Along this transect, five samples were taken, combined, and a subsample was used for further analysis of suspended algae.
Attached algal biomass was sampled quantitatively using artificial substrate (1 dm2 clay tiles). Nine tiles were fixed horizontally at 30 cm below the water surface, or if the water level was lower than 30 cm, at the bottom of the waterbody. Following Cattaneo and Amireault (Citation1992), each tile was suspended at the sampling site for six weeks to allow for sufficient colonization. Clay tiles as an artificial substrate were better suited for this study than natural substrate, as they resulted in higher reproducibility and comparability between sites as well as quantitative samples (Tuchman & Stevenson Citation1980). On each sampling event, the biofilm of three tiles was scraped with a razor blade and rinsed in a beaker. Remaining scraped material on the tile or razorblade was carefully rinsed into the beaker. Tap water (350 mL) was used to dissolve the biofilm to be able to quantify the attached algal biomass with delayed fluorescence spectrometry (as described below). The suspended and attached algal samples were analyzed within two hours after being taken.
Suspended and attached algal biomass were determined by analyzing the algal photopigment concentration via delayed fluorescence spectroscopy according to Gerhardt and Bodemer (Citation1998). Delayed fluorescence excitation spectroscopy is an in vivo rapid, non-destructive method for quantification and classification (algal group determination) of algal biomass (Bodemer Citation2002). Algal biomass quantification is based on the specific photosynthetically active pigment composition (PAP; chlorophylls, accessory pigments and phycobiliproteins), which is standardized to chlorophyll a. Delayed fluorescence is proportional to the PAP concentration which allows estimating algal biomass via photopigment concentration standardized to chlorophyll a (Gerhardt & Bodemer Citation1998).
Besides the quantification of algal biomass via photopigment concentrations, algae were classified with delayed fluorescence spectroscopy. Four different algal groups can be differentiated based on their distinct PAP composition: green algae (including Chlorophyceae, Euglenophyceae and Conjugatophyceae), diatoms (including Bacillariophyceae, Chrysophyceae, Dinophyceae and Xanthophyceae), cryptophytes, and cyanobacteria (Gerhardt & Bodemer Citation1998; Bodemer Citation2004). This work follows Gerhardt and Bodemer (Citation1998) and uses the term ‘algal group’ in the context of green algae and diatoms not in a taxonomic sense but rather for classification based on common pigment composition. Light intensity during algal growth influences pigment composition and concentration within a cell, thus altering delayed fluorescence measurements and outputs (Gerhardt & Bodemer Citation1998, Bodemer Citation2002). Photopigment alterations based on altering light conditions during algal growth were taken into consideration by the measuring device to ensure realistic measurement outputs (Bodemer Citation2002). Because interspecies differences in pigment composition occur (Bodemer Citation2002), the delayed fluorescence excitation spectrometer was calibrated with algal species expected to be dominant in German lotic waterbodies. The diatoms Navicula sp., the cyanobacteria Microcystis sp., the green algae Chlorella sp., and the cryptophyte Cryptomonas sp. were used for this purpose.
In addition to the aforementioned samples, one suspended and one attached algal sample from each sampling site in April 2012 was taxonomically identified. The samples were fixed in a 1% acid Lugol solution. The samples were microscopically evaluated using an Utermöhl inverted microscope (WILD, Heerbrugg, Schweiz) with a magnification of 1000 and a Hydrobios counting chamber (settled for 24h). Taxonomically, the algal cells were differentiated to the lowest level possible. Motile/no-motile and sestonic/benthic species were categorized according to Porter (Citation2008).
Structural and environmental parameters
In addition to algae sampling, site-specific, local environmental and structural parameters (secondary agents) were recorded at each sampling date. Parameters included dissolved oxygen (O2), conductivity (cond), pH, and water temperature (Tw), which were measured in situ with a WTW® multi parameter instrument 20 cm below the surface or 5 cm above the stream bed in shallow (<20 cm) systems. Additionally, channel width (width) and water depth (depth) were recorded along with flow velocity (vel) which was estimated using the drift method according to Schwoerbel (Citation1994). The vegetation canopy coverage (cano) and macrophyte coverage (macro) along a lateral line transect were estimated following Bonham (Citation1989). Turbidity (turb, in NTU) was measured photometrically in the laboratory.
Furthermore, chemical analysis measuring nitrate (N-NO3−), nitrite (N-NO2−), ammonium (N-NH4+), phosphate (P-PO43−) and chloride was conducted photometrically with WTW® cell test kits (14542 Nitrate NO3, N5/25 Nitrite NO2, 14559 Ammonium NH4, 14546 Phosphate PO4). Water samples to analyze nutrients and turbidity were collected using width-integrated sampling based on Shelton (Citation1994).
Structural classification (struct) following the German working group on water issues (LAWA; LAWA Citation2000) was assessed once in May 2012. Although the classification methods are only appropriate for natural waterbodies, they were used in this study in order to provide a comparison; the evaluation and classification methods were used for both natural streams and artificial ditches. Agricultural area within the drainage area (agric), distance of the sampling site to the origin (length), and drainage area (drain) at the sampling site were determined using ArcVIEW and ArcMAP 9.2 (ESRI, Inc.) with shape files and map data provided by the Hessian Agency for the Environment and Geology (HLUG Citation2006a). Weather data (air temperature, Ta; sunlight, sun, h/dmean; precipitation, precip, mm/dmean; cloud cover, cloud, okta/daymean) and day length (dayl, hrs) during the test period were obtained from the German Weather Service (DWD) measured at the weather station Neu-Ulrichstein (#10537) situated within a 15-km radius of the sampling sites. The mean of each climate parameter between the sampling dates was calculated and used in the analyses. Climatic data were considered as a regional parameter (primary agent).
Data analysis and statistics
Algal species data obtained in April 2012 was used to find similarities between suspended and attached species composition. For this purpose, the qualitative similarity measure, Sörensen's coefficient, and the quantitative measure, Bray–Curtis coefficient were calculated as described by Leyer and Wesche (Citation2007). On the basis of the quantitative and qualitative algal data based on PAPs, the following analyses were performed. To roughly estimate the contribution of suspended and attached algal fractions to primary production, a monthly, log-transformed ratio was calculated. In order to be able to compare suspended algae (expressed in biomass per volume) and attached algae (expressed in biomass per area), the suspended algal concentrations were transformed and related to the water column above the attached algal sampling device. Because of this, the suspended algal fraction was more abundant in PAP concentration when the log ratio was >0, whereas the attached algal fraction was more abundant in PAP concentration when the log ratio was <0.
The relationship between the 27 environmental and structural variables investigated and the suspended and attached algal group composition was tested using redundancy analysis (RDA). Prior to the analysis, a Pearson correlation matrix was determined with STATA 10.0 (StataCorp Citation2007), including all 27 structural and environmental variables to detect interrelated variables. Significances of the correlations were identified with a Bonferroni adjusted probability. If regression coefficients exceeded 0.85, only one of the correlating variables was used for further analyses. A regression coefficient >0.85 was found in correlations with water temperature, air temperature, and day length, oxygen concentration and saturation, as well as the drainage area and the distance to the origin. From these, the variables retained in the following analyses were water temperature, dissolved oxygen, and drainage area. Variables were ln(x + 1) transformed to achieve a normal distribution (van den Brink et al. Citation1995), with the exception of pH. Further analyses were conducted with CANOCO for Windows version 4.5 (terBraak & Šmilauer Citation2002) for suspended and attached algal data sets separately. Detrended correspondence analyses on the whole datasets were conducted, which revealed gradient lengths of <1.5 standard deviations, indicating the use of ordination techniques based on linear models (Van Wijngaarden et al. Citation1995). To mitigate the influence of interrelated variables, a series of partial RDAs was calculated constrained to one single environmental or structural variable. If variables explained a significant amount (p ≤ 0.05 assessed with a Monte Carlo permutation test of 499 permutations) of variation in suspended algae or attached algal data, variables were included in the final RDA. Partial RDAs revealed that five environmental or structural variables (cond, depth, macro, pH, width) had no significant contribution (p < 0.05) to variations in either of the two algal fractions and, thus, were excluded from the final RDA. Because canopy cover is a local variable, it was excluded in the final RDA of suspended algae and used only as an explanatory variable for the locally attached algae. The RDA was performed using forward selection in order to assess the minimum number of explanatory variables that explain species variation significantly (p ≤ 0.05, assessed with a Monte Carlo permutation test of 499 permutations). The significance (p ≤ 0.05) of the canonical axes was investigated using a Monte Carlo permutation test of 499 permutations.
Additionally, regressions were modelled for those environmental and structural variables detected by the final RDA to have a significant influence (p ≤ 0.05, assessed with a Monte Carlo test of 499 permutations) on algal group composition. These regression models were used to identify the influence of each predictor variable on suspended and attached algal groups. Calculations were performed using CanoDraw version 4.1 (terBraak & Šmilauer Citation2002). Specifically, algal group response curves were modelled using generalized linear models (GLMs) set to a linear degree, Poisson distribution, and a log link function. F-ratio statistics were used to test for significant correlations (p ≤ 0.05). Only those variables with significant correlations were visualized in the graphs. In order to compare sampling sites independent of seasonal variations, seasonality was included in the final RDA calculations as a covariable. The variance explained by the covariable was calculated following ter Braak and Šmilauer (Citation2002). In order to assess minimal number of explanatory variables explaining species variation significance (p < 0.05), a forward selection, using a Monte Carlo test with 499 permutations, was conducted. To assess seasonal differences of environmental and structural variables, as well as algal abundance and colour group composition, a one-way ANOVA was performed using GraphPad Prism version 5.01 (Citation2007).
Results
Structural and environmental characteristics
Water quality and habitat characteristics in streams and ditches varied widely among seasons (). Seasonal variations were pronounced and significant for all parameters displayed in with the exceptions of width, conductivity and chloride (one-way ANOVA, p ≤ 0.05). Agriculture dominated the drainage area of each sampling site and waterbody (50.9 ± 4.9%). The structural quality was classified as degraded to highly degraded. The intense agricultural use within the study area was further reflected in large peak concentrations of phosphate (2.00 mg P/L), ammonium (2.60 mg N/L) and nitrate (21.80 mg N/L), and in large seasonal mean concentrations of these nutrients ().
Algal composition
During April 2012, 88 taxa and 65 taxa in the suspended and attached algal fraction were identified, respectively. Diatoms were predominant in both algal fractions (suspended: 62.1 ± 20.9%, attached: 80.1 ± 20.5%; ). In the suspended algal fraction, 16.6 ± 6.6% motile, 61.5 ± 20.2% sestonic and 29.8 ± 19.4% benthic species were distinguished. In the attached algal fraction, 17.6 ± 11.3% were motile, 23.3 ± 17.2% were sestonic and 69.7 ± 14.8% were benthic species (). The dominant species with a relative abundance >2% in the suspended and attached algal fractions are shown in . The suspended algal fraction classes were dominated by the sestonic diatom Cocconeis pediculus, the sestonic green algae Chlorella vulgaris and the sestonic cyanobacteria Nodularia spumigena. In the attached algal fraction, the classes were dominated by the benthic diatom Achnanthes conspicua, the green algae Chloromonas sp. and the benthic cyanobacteria Oscillatoria limosa sp. (). Cryptophytes were not found in either fraction in abundances >2%.
Table 2. Mean abundance of suspended and attached algal species counted at all sampling sites in April 2012.
Over the entire study period, PAP concentration of suspended algae ranged between 0.2 and 51.7 µg/L PAP (minimum and maximum measured). Diatoms dominated the suspended algal fraction during all seasons except summer, when green algae dominated (). Over the course of the study, attached algae had larger fluctuations in PAP concentration than those of the suspended algae. These fluctuations ranged from 0.4 to 272.2 µg/dm2 PAP (minimum and maximum measured). Like the suspended algal fraction, diatoms were also the predominant group represented in the attached algal fraction ().
Seasonal variations in total PAP and colour group composition in both suspended and attached algae observed during the study period were pronounced and significant (one-way ANOVA, p ≤ 0.05, ). Not only were there variations between seasons within the attached and suspended algae community but also the ratio between suspended and attached algal biomass changed with season. The contribution of the suspended algal fraction to the total algal community biomass was at its largest in late summer and early autumn (). During these seasons, the log suspended:attached algal ratio was above zero (including the confidence intervals; ). In spring and late autumn, the attached algal fraction was more abundant resulting in a negative ratio (). Hence, in these seasons, the attached algal fraction contributed considerably more to the total algal community biomass.
Figure 2. Monthly averaged, log transformed suspended:attached algal ratio calculated. It was calculated with the monthly mean of the suspended photosynthetically active pigments (PAP) above the area sampled for attached algae at all sites and the monthly mean of the attached algae PAP. Displayed are 95% confidence intervals for each monthly mean. The dashed reference line separates the two areas where either the suspended algal fraction (>0) or the attached algal fraction (<0) increases their contribution to the algal community.
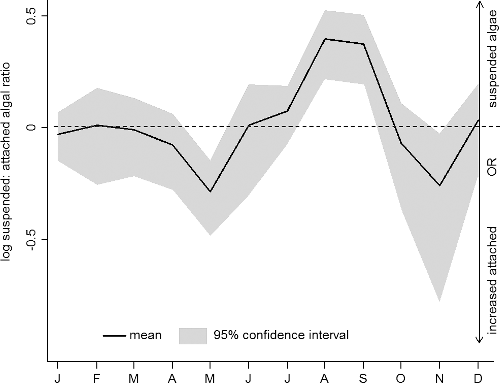
Evaluating the species composition, communities dissimilar in both species composition and abundances can be observed, even though many abundant taxa occurred in both algal fractions (). This finding is substantiated by the Sörensen similarity index, a qualitative index, which was 39.9% (mean of all sites), and the Bray–Curtis index, a quantitative index, which was 16.6% (mean of all sites).
Algal–environmental and structural factor correlation
The final RDA on the suspended algal fraction identified five environmental and structural variables that accounted for a significant amount (p ≤ 0.05, assessed with a Monte Carlo permutation test) of explainable variation in the suspended algal data ( and ). Temperature (11.5%), velocity (6.5%), and nutrients (sum of NO3−-N, NH4+-N and PO4−3-P 11.8%) had the highest explanatory power over the overall variance in suspended algal group variation (). The canonical axes collectively accounted for 39.3% of the overall variance within the suspended algal fraction (). Greater nitrate concentrations were primarily measured during spring (). This season was also associated with high abundances of diatoms and cryptophytes. These aforementioned values plotted in the upper left quadrant (). High temperatures and ammonium concentrations plotted in the lower left quadrant, and were so associated with samples from summer, green algae and cyanobacteria (). Winter samples plotted in the right quadrants and were associated with large velocities and oxygen concentrations (). Autumn samples distributed over all four quadrants ().
Figure 3. Redundancy analysis, showing suspended algal group scores (Diat, diatoms; Gre, green algae; Cry, cryptophytes; Cya, cyanobacteria), plotted relative to the significant environmental and structural variables (p ≤ 0.05, assessed with a Monte Carlo permutation test) and sampling sites. Sampling sites were classified according to season; spring: •, summer: ■, autumn: ▴, winter: x. In suspended algal composition, 39.2% of the variations can be explained by the environmental and structural variables, of which 96.7% is displayed by the first two axes.
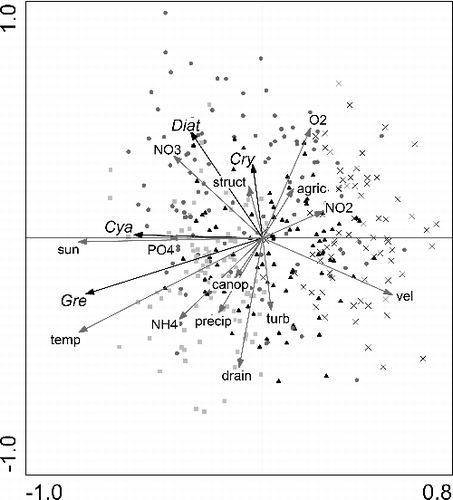
Table 3. Explanatory power of the environmental and structural variables (%) from RDA analyses.
Table 4. Summary of redundancy analyses (RDA) performed with the suspended and attached algal dataset with and without introducing season as a covariable.
Based on RDA and corresponding GLMs performed with the suspended algal dataset, diatoms were significantly (p < 0.05, determined with an F-ratio statistic) associated with seven of the fourteen environmental and structural variables ( and ). The diatoms showed greater abundance in nutrient- and oxygen-rich sites and during seasons with high radiation (sunlight) and sites with low flow velocity. Also, diatoms were the only group significantly correlated with turbidity (p < 0.05, determined with an F-ratio statistic, ). Green algae were significantly associated with all variables, except structural classification and turbidity (p < 0.05, determined with an F-ratio statistic, ). Specifically, sampling times and sites with increased temperature and nutrients were correlated with increased abundance of green algae (). Green algae were the only group significantly correlated with ammonium (p < 0.05, determined with an F-ratio statistic, ). The trend in the abundances of green algae and oxygen, drainage area and precipitation were opposing to those of trends in diatoms abundance. Cyanobacteria usually followed the same trends as that of the green algae, with the exceptions of ammonium and oxygen concentration as well as drainage area which were not significantly correlated with cyanobacteria abundance (p < 0.05, determined with an F-ratio statistic, ). Cryptophytes were weakly associated with the explanatory variables, but generally followed the trends of diatoms. Suspended algal group composition and abundance were dependant on season as visualized in the triplot (). The importance of season was further demonstrated, using the sampling dates as covariables in the RDA. Using the seasons as such, it explained 56.7% of the total variance in suspended algal group composition and abundance ().
Figure 4. Abundance of suspended algal groups (Diat, diatoms; Gre, green algae; Cry, cryptophytes; Cya, cyanobacteria) plotted versus significant (p ≤ 0.05) environmental and structural variables (log(x+1) transformed), assessed with a Monte Carlo permutation test. A line-of-fit, estimated by GLM, is plotted for suspended algal groups which are significantly correlated with environmental and structural variables (determined with F-ratio statistics p < 0.05).
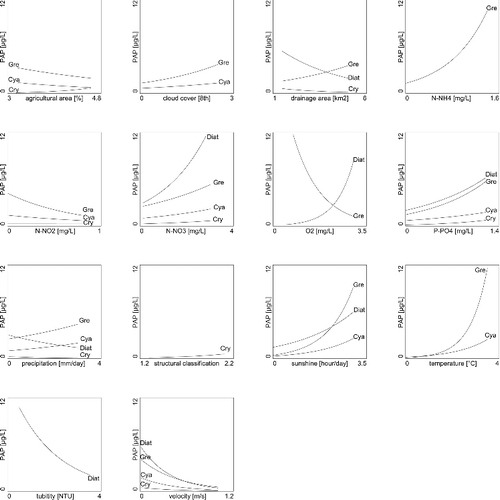
The final RDA, using environmental and structural variables as well as the attached algae dataset, identified five variables accounting for a significant amount of explainable variation in the attached algal community (). Ordered by explanatory power of the overall variance in suspended algae, light regime (12.2%) (represented by sunlight (7.5%), canopy cover (2.6%) and turbidity (2.1%)), velocity (2.6%), and nitrate (2.4%) were most influential factors ( and ). Within the attached algae data set, 25.6% of the variation could be explained collectively by the canonical axes ().
Figure 5. Redundancy analysis, showing attached algal group scores (Diat, diatoms; Gre, green algae; Cry, cryptophytes; Cya, cyanobacteria), plotted relative to the significant environmental and structural variables (p ≤ 0.05, assessed with a Monte Carlo permutation test) and sampling sites. Sampling sites were classified according to season; spring: •, summer: ■, autumn: ▴, winter: x. In attached algal composition, 27.4% of the variations can be explained by the environmental and structural variables, of which 95.3% is displayed by the first two axes.
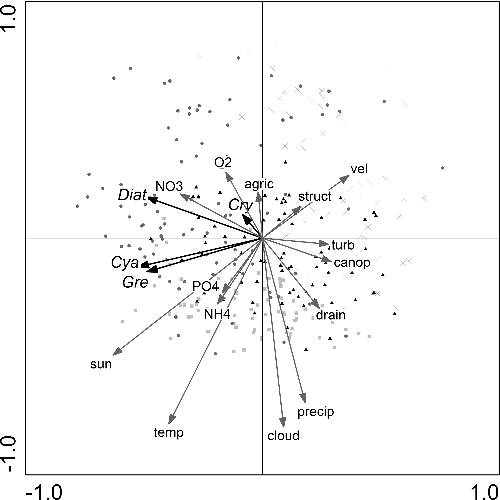
A triplot of attached algal group scores, significant environmental and structural variables (p ≤ 0.05, assessed with a Monte Carlo test) and sampling dates classified according to season illustrates overall trends (). Typically, sites of large nitrate and oxygen concentrations plotted in the upper left quadrant, and were associated with sites sampled in spring as well as diatoms and cryptophytes. High temperature and sunlight plotted in the lower left quadrant, and were associated with summer samples where Chlorophyta and cyanobacteria were most dominant. Winter samples usually plotted in the upper right quadrants, and were associated with large velocities. Autumn samples distributed over all four quadrants. Based on this RDA and the corresponding GLMs, diatoms were significantly associated with nine of the fourteen environmental and structural variables, and showed high associations with nitrate and light regime (induced by low canopy cover, low cloud cover and low turbidity; ). Diatoms were the only colour group significantly associated with cloud cover, drainage, and precipitation (p < 0.05, determined with an F-ratio statistic). Green algae and cyanobacteria were found in increasing abundance when temperature, sunlight and nutrient concentrations (NO3, PO4 and NH4) increased. The two groups followed similar trends only differing with respect to ammonium (significantly associated only with green algae, p < 0.05), oxygen concentration and agricultural area (only significantly associated with cyanobacteria p < 0.05; ). Cryptophytes were only significantly associated with structural classification (p < 0.05, determined with an F-ratio statistic). As in the suspended algal data, season was of major importance in attached algal group assemblage ( and ). This finding was substantiated by the RDA using the sampling dates as covariables, where 44.3% of the total variance in attached algal group composition was explained by the sampling data ().
Figure 6. Abundance of attached algal groups (Diat, diatoms; Gre, green algae; Cry, cryptophytes; Cya, cyanobacteria) versus significant (p ≤ 0.05) environmental and structural variables (log(x + 1) transformed), assessed with a Monte Carlo permutation test. A line-of-fit, estimated by GLM, is plotted for attached algal groups which are significantly correlated with environmental and structural variables (determined with F-ratio statistics p < 0.05).
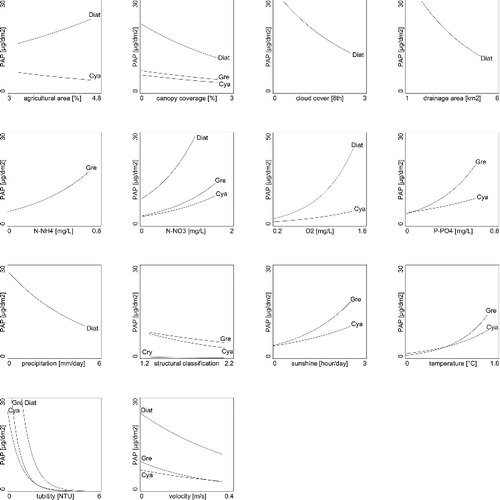
In summary, the same factors affected suspended and attached algae; however, the intensity of the influence varied between the two fractions. Light regime (represented by turbidity, sunlight and canopy cover) mainly influenced attached algae, whereas suspended algae were primarily influenced by temperature, nutrients and velocity. Also, algal groups were similarly affected by environmental and structural factors in suspended and attached algal fractions as visualized in the GLMs. Major differences could be found in the responses to turbidity, where the diatoms were the only group significantly affected among the suspended algae (p < 0.05, determined with an F-ratio statistic), whereas in attached algae, all groups were significantly and negatively correlated (p < 0.05, determined with an F-ratio statistic). Additionally, RDA-determined similar ordination of the groups along season was possible.
Discussion
Algal community and species composition
In agriculturally influenced ditches and streams in central Germany, suspended algal biomass contributes considerably to total algal biomass, exceeding attached algal biomass in late summer and early autumn. Even though the ratio is only a rough estimate, it indicates the contribution of both algal fractions to primary production. These findings contradicted previous research which indicated that benthic, attached algae should be the only abundant algal fraction in small lotic ecosystems (Vannote et al. Citation1980; Hötzel & Croome, Citation1999). In all waterbodies studied in this study, both algal fractions contributed considerably to primary productivity with the dominant fraction being dependant on season rather than on waterbody type. The findings in this study support the ones by Porter (Citation2008) which suggest the establishment of increased sestonic algal biomass in agriculturally influenced small lotic waterbodies as these ecosystems are usually autotrophic (Simon & Travis Citation2011).
In order to further evaluate the ecological significance of the suspended algal faction, the origin of this fraction needs to be considered. Historically, it was proposed, that suspended riverine algae in small lotic waterbodies were derived from attached algal populations, and were in a dynamic equilibrium within the two stages of their lifecycle (suspended and attached). It was further proposed that these populations were simply accidentally dislodged or originated from upstream lentic communities (Hötzel & Croome Citation1999; Bellinger & Sigee Citation2010). This point of view was questioned by Wu et al. (Citation2011) who found truly riverine algae species which developed substantial sestonic in situ populations in small streams in Germany. In agreement with Wu et al. (Citation2011), this study also showed that the suspended algal fraction (April 2012) was predominantly composed of sestonic algae. This finding is suggestive of a species composition with a large proportion of species possessing a photosynthetically active growth phase within the water column, rather than the suspended populations arising from accidentally dislodged benthic populations. The rather dissimilar suspended and attached species compositions (quantitative and qualitative) expressed with the Sörensen and Bray–Curtis similarity indices further support these findings. Nevertheless, interchanges between the two algal fractions occur, since a proportion of attached benthic algae were found in the suspended fraction and vice versa. The origin of the algae in both fractions were not the lentic ecosystems upstream, because no such systems are part of the waterbodies and because both algal fractions were composed of dominant riverine species such as Achnanthes, Clamydomonas, Cocconeis, Navicula and Nodularia (Bellinger & Sigee, Citation2010; Wu et al. Citation2011). This suggests a truly riverine algal community within the small lotic ecosystems such as that found by Wu et al. (Citation2011). However, the findings, based on species determination, only apply to one sampling date, which allows only for suggestions and which hinders drawing general conclusions. With regard to the findings based on community data over the whole study period and species data on one date, suspended algae should be considered from a new perspective, such as that of Wu et al. (Citation2011), and should be included in more study designs that investigate algal communities in small lotic ecosystems.
Factors explaining the suspended algal distribution
Because the suspended and attached algal fractions were found to be rather different from each other with dominant sestonic and benthic algal species, respectively, a separate factor analysis and discussion was conducted for the two algal fractions. Several factors, including temperature, nutrients, velocity, and turbidity could be related to community distribution and abundance in the suspended algal fraction. In previous studies, temperature was found to have large impacts on algal growth and on growth period duration, leading to seasonal variations in algal composition and abundance (Baker & Baker Citation1979; Munn et al. Citation2002; Yang et al. Citation2008). Temperature was identified in this study to be the most influential factor, causing seasonal variability in suspended algal abundance and composition. Green algae and cyanobacteria were especially influenced by temperature, being positively correlated and associated with those samples taken in summer. These observations for smaller lotic ecosystems were previously found in larger lotic waterbodies, where there was an increase in green algae and cyanobacteria abundance during summer (Stevenson & White Citation1995). The decreased solubility of oxygen with rising temperatures and the strong dependency on green algal growth and temperature resulted in the observed negative correlation with green algal abundance and oxygen concentration. Schwörbel's and Brendelberger (Citation2005) observation of increased diatom abundances in colder seasons such as spring and autumn was supported by this study. In this study, rising diatom populations were associated with sinking temperatures and, consequently increased solubility of oxygen.
Nutrients (NO3, NH4 and PO4, excluding NO2) were found in high concentrations reflecting the overriding role of agricultural land causing eutrophication in the whole Ohm basin. Not surprisingly, they were identified to be, besides temperature (season), the second most influential factors determining suspended algal group composition and abundance. Due to the high phosphate and nitrate concentrations at the sampling sites (suggested threshold for nutrient limitation: <30 µg P/L, <1 mg N/L; Hilton et al. Citation2006), nutrients were not the limiting factor determining suspended algal abundance as found in other sestonic ecosystems where nitrate and phosphate are a primary constraint on suspended algal abundance and community composition (Kohler Citation1994; Basu & Pick Citation1996; Leland Citation2003; Wu et al. Citation2011). As previously stated, eutrophic conditions as found at the study sites, are a main driver for suspended algal growth (e.g. Welch et al. Citation1988; Dodds et al. Citation2002; Hilton et al. Citation2006) and explain not only the RDA results but also the increased suspended algal concentrations at the study sites, which exceed concentrations found in other small streams (e.g. Yang et al. Citation2008). Nutrients affected the suspended algal colour groups equally in this study. All suspended algal colour groups were positively correlated with nutrients rather than a single algal group. This is supported by previous research (Bellinger & Sigee Citation2010; Wu et al. Citation2011). Rather than colour group composition, species composition was affected more by nutrient affinity or tolerance. In the waterbodies investigated, species such as A. conspicua, C. pediculus or Nitzschia palea known to be present at high nutrient concentrations (Porter Citation2008; Bellinger & Sigee Citation2010) dominated in April 2012.
A large percentage of variability in suspended algal group composition, identified by the RDA, was ascribed to velocity. Velocity influences resources (e.g. nutrient availability, suspended particles, and, thus, light availability) and other abiotic factors (e.g. temperature gradients), thereby substantially affecting algae biomass (Biggs Citation1995; Stevenson Citation1997; Townsend et al. Citation2012). Also, residence time of the water, which determines the opportunity for converting nutrients into new biomass, has been found to be an important aspect of velocity, affecting both suspended algal abundance and composition (Baker & Baker Citation1979; Stevenson & White Citation1995; Leland Citation2003; Bukaveckas et al. Citation2011). Hence, previous findings applying to larger lotic ecosystems are transferrable to smaller lotic waterbodies. At the algal group level, diatoms especially have been found to be strongly and negatively correlated with velocity (Leland et al. Citation2001), which are findings that were observed in this study.
In the suspended algal fraction, turbidity explained only a small proportion of algal group variability in these smaller shallow waterbodies. In eutrophic, turbid environments, a shift in species composition towards species that harvest light energy efficiently, independent of the algal group affiliation (green algae, diatoms, cyanobacteria or cryptophytes), has previously been observed (Leland et al. Citation2001). This previously observed shift in species towards a community with species that harvest light more efficiently could not be transferred to algal group level, because in this study, light regime explains only small parts of the variation in suspended algal group composition and abundance. Hence, it is not a primary constraint on suspended algal group composition. Only diatoms were significantly correlated with turbidity, a finding that matches previous findings, where relative suspended diatoms abundance decreased with decreasing light availability (turbidity and depth; Sanchez et al. Citation2013).
Factors explaining attached algal distribution
Attached algal group assemblage and environmental and structural factors related to the distributional abundance in this study confirm findings of previous studies. Attached benthic algae have been extensively studied in small lotic ecosystems where light availability, determined by canopy cover, day length, sunlight and turbidity, for example, was one of the main influencing factors (Roberts et al. Citation2004; Hillebrand Citation2005; Matheson et al. Citation2012). Deviations observed in previous findings (e.g. Dodds et al. Citation1998; Leland et al. Citation2001; Dodds Citation2006; Black et al. Citation2011) could be found in the influence of nutrients and velocity. Even though high nutrient concentrations were found and waterbodies could usually be classified as eutrophic (Dodds et al. Citation1998; Dodds Citation2006), excessive benthic algal concentrations (biomass >100–150 µg/m2) as defined by Welch et al. (Citation1988) were not encountered in this study. This contrasts findings of many other studies, where nutrients are the most influential factor determining attached algal growth (Leland et al. Citation2001; Dodds Citation2006; Black et al. Citation2011). As stated by Hilton et al. (Citation2006), all environmental factors required for plant growth (e.g. light availability, trace nutrients) must be accessible for plants to reach their full growth potential. Thus, the results in this study indicate a limitation owing to other environmental or structural factors (e.g. light attenuating factors) as previously documented by Munn et al. (Citation2010). Despite the lack of nuisance benthic algal concentrations, high abundance of nutrient-tolerant species in April 2014 (e.g. A. conspicua or N. palea) could be found, showing that on a species level, nutrients influence community composition as previously demonstrated (e.g. Dodds Citation2006; Black et al. Citation2011) but at a colour group level, the changes were not noticeable as all colour group growth was equally enhanced.
Because velocity was always below the shear stress inducing threshold of 1 m/s (Biggs Citation1995), this parameter had less influence on attached algal group composition in the small waterbodies investigated. Furthermore, the positive correlations of velocity and attached algae abundance due to higher nutrient availability found by Townsend et al. (Citation2012) could not be confirmed in this study, possibly due to a generally large nutrient concentration in the water column.
Light regime, temperature, velocity and nutrients had primary influence on both algal fractions in the waterbodies investigated. However, the extent of the influence of these structural and environmental factors differed between suspended and attached algae. The distinct habitat of suspended algae in the water column and attached algae on the streambed is the probable cause for a difference in dominant environmental and structural factors affecting suspended and attached algal group assemblage. Since attached algal group composition is affected by different main influential environmental and structural factors than suspended algal group composition, conclusions based on one algal fraction may not be transferred to the other fraction in the same ecosystem or region. This finding is further substantiated, when the strongly differing species composition of the two algal fractions is considered.
Regional- and site-specific parameters
Suspended and attached algae can be significantly influenced by a variety of environmental and structural factors which range from large-scale, regional variables (e.g. climate), to intermediate-scale, basin-specific variables (e.g. land use), to finer-scaled, channel-or site-specific factors (e.g. turbidity, velocity; Stevenson Citation1997; Kent et al. Citation2007). As shown in this study, in small streams and ditches in central Germany, different factors acted on different scales and simultaneously affected algae composition. Andrus et al. (Citation2013) showed that seasonal variation is the major gradient along which algal assemblages (both suspended and attached algae) vary. Seasonal variations at the study sites were shown by RDAs (for both algal fractions), clearly differentiating samples from different seasons. Additionally, season (sampling dates) explained a large proportion of the total variance in both algal communities, when introduced as covariables in the analyses. Thus, the results support the findings by Andrus et al. (Citation2013).
Alongside other regional- and basin-specific factors, such as geology and land use, seasonality ultimately determines algal assemblage, function and structure (Biggs & Gerbeaux Citation1993; Stevenson Citation1997; Soininen et al. Citation2004; Kent et al. Citation2007; Bernot et al. Citation2010). The seasons are critical to long-term average production (Biggs & Gerbeaux Citation1993; Munn et al. Citation2002) and they induce spatial synchronicity (e.g. Andrus et al. Citation2013). Spatial synchronicity in both supended and attached algal fractions was also shown in this study, where seasonal differentiation independent of sampling site was generally possible.
Even though season was shown to be a very important variable influencing suspended and attached algae, the influence and importance of local, basin-specific factors should not be neglected or underestimated. Although constrained by primary agents, local site-specific factors influence algal group composition and abundance substantially, especially short-term temporal dynamics (Biggs & Gerbeaux Citation1993). Thus, investigations should include environmental and structural parameters at different scales.
Suspended algae were shown to reach substantial concentrations in comparison with attached algae in small waterbodies in central Germany. This demonstrated that suspended algae contribute to the productivity of these small lotic waterbodies and might be of relevance in algal analysis and algal monitoring. Environmental and structural parameters, such as climate, nutrients, flow and light regime, were determined to have the largest influence on suspended and attached algal group composition. Even though both algal fractions were characterized by distinct habitats, similar influential parameters were found to determine attached algal group composition. However, the intensities of the influence of each variable varied between algal communities and conclusions based on solely attached algae may not be extrapolated to suspended algal fractions. The different intensity of the influential variables acting on the suspended and attached algal fraction emphasizes the recommendation that suspended algae should be included in algal investigations in small lotic waterbodies.
So far, there is no general understanding as to which factors regulate suspended algal communities in lotic ecosystems, especially in small waterbodies. Thus, additional information provided in this study is valuable for ecological evaluation and modelling of algae and to understand ecosystem functioning since both algal fractions may affect ecosystem processes, function and stability. In order to further verify the findings of this study and draw general conclusion on suspended and attached algal communities in small streams, further research especially in other ecoregions is necessary. Future research should focus on suspended algal communities, and their community composition especially in terms of species composition. But also the influence of environmental factors acting on these algal communities needs to be investigated further as suspended algae in small lotic ecosystems are still poorly investigated and understood.
Acknowledgments
The Hessian State Agency for Environment and Geology (HLUG) is acknowledged for their support and provision of data and digital maps. Thanks to JörgÖhlmann, LászlóDören and Susanna Dart for their suggestions to improve the manuscript.
Disclosure statement
No potential conflict of interest was reported by the authors.
Additional information
Funding
Notes on contributors
Friederike Breuer
Friederike Breuer is a PhD student at Mesocosm GmbH and the Goethe University, Frankfurt where she works in the field of aquatic ecotoxicology and ecology, mainly investigating the application of algal measuring techniques.
Philipp Janz
Philipp Janz is a PhD student at Mesocosm GmbH where he develops lotic mesocosms.
Eamonn Farrelly
Eamonn Farrelly is a Senior Technical Expert at Syngenta.
Klaus-Peter Ebke
Klaus-Peter Ebke is the leading scientist and study director of Mesocosm GmbH where he leads and manages aquatic ecotoxicological studies.
References
- Andrus JM, Winter D, Scanlan M, Sullivan S, Bollman W, Waggoner JB, Hosmer AJ, Brain RA. 2013. Seasonal synchronicity of algal assemblages in three Midwestern agricultural streams having varying concentrations of atrazine, nutrients, and sediment. Sci Total Environ. 458:125–139.
- Baker AL, Baker KK. 1979. Effects of temperature and current discharge on the concentration and photosynthetic activity of the phytoplankton in the upper Mississippi River. Freshw Biol. 9:191–198.
- Basu BK, Pick FR. 1995. Longitudinal and seasonal development of planktonic chlorophyll-a in the Ridequ River, Ontario. Can J Fish Aquat Sci. 52:804–815.
- Basu BK, Pick FR. 1996. Factors regulating phytoplankton and zooplankton biomass in temperate rivers. Limnol Oceanogr. 41:1572–1577.
- Bellinger E, Sigee D. 2010. Freshwater algae. Chinchester: Wiley.
- Bernot MJ, Sobota DJ, Hall RO, Mulholland PJ, Dodds WK, Webster JR, Tank JL, Ashkenas LR, Cooper LW, Dahm CN, et al. 2010. Inter-regional comparison of land-use effects on stream metabolism. Freshw Biol. 55:1874–1890.
- Biggs BJF. 1995. The contribution of flood disturbance, catchment geology and land-use to the habitat template of periphyton in stream ecosystems. Freshw Biol. 33:419–438.
- Biggs BJF. 2000. Eutrophication of streams and rivers: dissolved nutrient–chlorophyll relationships for benthic algae. J N Am Benthol Soc. 19:17–31.
- Biggs BJF, Gerbeaux P. 1993. Periphyton development in relation to macroscale (geology) and microscale (velocity) limiters in 2 gravel-bed rivers, New-Zealand. N Z J Mar Fresh. 27:39–53.
- Black RW, Moran PW, Frankforter JD. 2011. Response of algal metrics to nutrients and physical factors and identification of nutrient thresholds in agricultural streams. Environ Monit Assess. 175:397–417.
- Bodemer U. 2002. Verzögerte Fluoreszenz - In vivo Analyse von Algenpigmenten und ihre Anwendung in der Limnologie [In vivo analysis of algal pigments and their application in limnology] [ dissertation]. Vienna: University of Vienna.
- Bodemer U. 2004. Variability of phycobiliproteins in cyanobacteria detected by delayed fluorescence excitation spectroscopy and its relevance for determination of phytoplankton composition of natural water samples. J Plankton Res. 26:1147–1162.
- Bonham CD. 1989. Measurements for terrestrial vegetation. New York: Wiley.
- Breuer F, Janz P, Farrelly E, Ebke P. 2016. Seasonality of algal communities in small streams and ditches in temperate regions using delayed fluorescence. J Freshwater Ecol. 1:1–5.
- Bukaveckas PA, MacDonald A, Aufdenkampe A, Chick JH, Havel JE, Schultz R, Angradi TR, Bolgrien DW, Jicha TM, Taylor D. 2011. Phytoplankton abundance and contributions to suspended particulate matter in the Ohio, Upper Mississippi and Missouri Rivers. Aquat Sci. 73:419–436.
- Carpenter SR, Caraco NF, Correll DL, Howarth RW, Sharpley AN, Smith VH. 1998. Nonpoint pollution of surface waters with phosphorus and nitrogen. Ecol Appl. 8:559–568.
- Cattaneo A, Amireault MC. 1992. How artificial are artificial substrata for periphyton. J N Am Benthol Soc. 11:244–256.
- Dodds WK. 2006. Eutrophication and trophic state in rivers and streams. Limnol Oceanogr. 51:671–680.
- Dodds WK, Jones JR, Welch EB. 1998. Suggested classification of stream trophic state: distributions of temperate stream types by chlorophyll, total nitrogen, and phosphorus. Water Res. 32:1455–1462.
- Dodds WK, Smith VH, Lohman K. 2002. Nitrogen and phosphorus relationships to benthic algal biomass in temperate streams. Can J Fish Aquat Sci. 59:865–874.
- EPA U. S. 1998. National strategy for the development of regional nutrient criteria. In: U.S. EPA: 822-F-98-002. Washington (DC): U.S. Environmental Protection Agency Office of Water Fact Sheet.
- Gerhardt V, Bodemer U. 1998. Delayed fluorescence excitation spectroscopy: a method for automatic determination of phytoplankton composition of freshwaters and sediments (interstitial) of algal composition of benthos. Limnologica. 28:313–322.
- GraphPad-Software. 2007. GraphPad Prism 5.00 for Windows. San Diego (CA): GraphPad-Software.
- Hamilton SK, Tank JL, Raikow DF, Wollheim WM, Peterson BJ, Webster JR. 2001. Nitrogen uptake and transformation in a midwestern US stream: a stable isotope enrichment study. Biogeochemistry. 54:297–340.
- Hillebrand H. 2005. Light regime and consumer control of autotrophic biomass. J Ecol. 93:758–769.
- Hilton J, O'Hare M, Bowes MJ, Jones JI. 2006. Ho green is my river? A new paradigm of eutrophication in rivers. Sci Total Environ. 365:66–83.
- HLUG. 2006a. Geodatenkatalog Hessen [Geodata catalogue for Hesse]. Wiesbaden: Hessisches Landesamt für Umwelt und Geologie [Hessian State Office for Environment and Geology] (HLUG).
- HLUG. 2006b. WRRL in Hessen version 2.3 [Water Framework Directive in Hesse, version 2.3]. In: HMUELV (Hessisches Ministerium für Umwelt, Energie, Landwirtschaft und Verbraucherschutz) [HMUELV (Hessian Ministry for rhe Environment, Energy, Agriculture and Consumer Production)] [Internet]. Wiesbaden: HLUG; [cited 2014 Mar 21]. Available from: http://wrrl.hessen.de/viewer.htm
- Hötzel G, Croome R. 1999. A phytoplankton methods manual for Australian freshwaters. Canberra: Land and Water Resources Research and Development Corporation Occasional Paper 22/99.
- Kent AD, Yannarell AC, Rusak JA, Triplett EW, McMahon KD. 2007. Synchrony in aquatic microbial community dynamics. ISME J. 1:38–47.
- Kohler J. 1994. Origin and succession of phytoplankton in a river–lake system (Spree, Germany). Hydrobiologia. 289:73–83.
- Lamberti GA. 1996. The role of periphyton in benthic food webs. In: Stevenson RJ, Bothwell ML, Lowe RL, editors. Algal ecology: freshwater benthic ecosystems. San Diego (CA): Elsevier Academic Press.
- [LAWA] Bund/Länder-Arbeitsgemeinschaft Wasser [Government/State Water Working Group]. 2000. Gewässerstrukturgütekartierung in der Bundesrepublik Deutschland, Verfahren für kleine und mittelgroße Fließgewässer [Maping the structure of waterbodies in Germany]. Berlin: Kulturbuch-Verlag GmbH.
- Leland HV. 2003. The influence of water depth and flow regime on phytoplankton biomass and community structure in a shallow, lowland river. Hydrobiologia. 506:247–255.
- Leland HV, Brown LR, Mueller DK. 2001. Distribution of algae in the San Joaquin River, California, in relation to nutrient supply, salinity and other environmental factors. Freshw Biol. 46:1139–1167.
- Leyer I, Wesche K. 2007. Multivariate Statistik in der Ökologie [Multivariat statistics in ecology]. Heidelberg: Springer.
- Matheson FE, Quinn JM, Martin ML. 2012. Effects of irradiance on diel and seasonal patterns of nutrient uptake by stream periphyton. Freshw Biol. 57:1617–1630.
- Munn MD, Black RW, Gruber SJ. 2002. Response of benthic algae to environmental gradients in an agriculturally dominated landscape. J N Am Benthol Soc. 21:221–237.
- Munn MD, Frey J, Tesoriero A. 2010. The influence of nutrients and physical habitat in regulating algal biomass in agricultural streams. Environ Manage. 45:603–615.
- Munn MD, Osborne LL, Wiley MJ. 1989. Factors influencing periphyton growth in agricultural streams of central Illinois. Hydrobiologia. 174:89–97.
- Nienhuis PH. 1993. Nutrient cycling and foodwebs in Dutch estuaries. Hydrobiologia. 265:15–44.
- Piirsoo K, Pall P, Tuvikene A, Viik M. 2008. Temporal and spatial patterns of phytoplankton in a temperate lowland river (Emajogi, Estonia). J Plankton Res. 30:1285–1295.
- Porter SD. 2008. Algal attributes: an autecological classification of algal taxa collected by the national water-quality assessment program [Internet]. U.S. Geological Survey Data Series 329; [cited 2014 Oct 15]. Available from: http://pubs.usgs.gov/ds/ds329/
- Reynolds CS, Descy JP. 1996. The production, biomass and structure of phytoplankton in large rivers. Arch Hydrobiol Suppl. 113:161–187.
- Roberts S, Sabater S, Beardall J. 2004. Benthic microalgal colonization in streams of differing riparian cover and light availability. J Phycol. 40:1004–1012.
- Rosemond AD, Mulholland PJ, Brawley SH. 2000. Seasonally shifting limitation of stream periphyton: response of algal populations and assemblage biomass and productivity to variation in light, nutrients, and herbivores. Can J Fish Aquat Sci. 57:66–75.
- Sanchez LM, Perez LG, Izaguirre I, Pizarro H. 2013. Influence of underwater light climate on periphyton and phytoplankton communities in shallow lakes from the Pampa plain (Argentina) with contrasting steady states. J Limnol. 72:62–78.
- Schwoerbel J. 1994. Methoden der Hydrobiologie [Methods of hydrobiology]. Stuttgart: Gustav Fischer Verlag.
- Schwoerbel J, Brendelberger H. 2005. Einführung in die Limnologie [Introduction to limnology]. München: Elsevier GmbH.
- Shelton LR. 1994. Field guide for collecting and processing streamwater samples for the National Water-Quality Assessment Program. Reston (VA): U.S. Geological Survey. (U.S. Geological Survey Open-File Report).
- Simon TN, Travis J. 2011. The contribution of man-made ditches to the regional stream biodiversity of the new river watershed in the Florida panhandle. Hydrobiologia. 661:163–177.
- Soininen J, Paavola R, Muotka T. 2004. Benthic diatom communities in boreal streams: community structure in relation to environmental and spatial gradients. Ecography. 27:330–342.
- StataCorp. 2007. Stata statistical software: release 10. College Station (TX): StataCorp LP.
- Stevenson RJ. 1996. An introduction to algal ecology in freshwater benthic habitats. In: Stevenson RJ, Bothwell ML, Lowe RL, editors. Algal ecology: freshwater benthic ecosystems. San Diego (CA): Elsevier Academic Press.
- Stevenson RJ. 1997. Scale-dependent determinants and consequences of benthic algal heterogeneity. J N Am Benthol Soc. 16:248–262.
- Stevenson RJ, White KD. 1995. A comparison of natural and human determinants of phytoplankton communities in the Kentucky River basin, USA. Hydrobiologia. 297:201–216.
- ter Braak CJF, Šmilauer P. 2002. CANOCO reference manual and CanoDraw for windows user's guide: software for canonical community ordination (v 4.5). Ithaca (NY): Microcomputer Power.
- Townsend SA, Garcia EA, Douglas MM. 2012. The response of benthic algal biomass to nutrient addition over a range of current speeds in an oligotrophic river. Freshw Sci. 31:1233–1243.
- Tuchman ML, Stevenson RJ. 1980. Comparison of clay tile, sterilized rock, and natural substrate diatom communities in a small stream in southeastern Michigan, USA. Hydrobiologia. 75:73–79.
- van den Brink PJ, van Donk E, Gylstra R, Crum SJH, Brock TCM. 1995. Effects of chronic low concentrations of the pesticides Chlorpyrifos and Atrazine in indoor fresh-water mesocosms. Chemosphere. 31:3181–3200.
- Vannote RL, Minshall GW, Cummins KW, Sedell JR, Cushing CE. 1980. River continuum concept. Can J Fish Aquat Sci. 37:130–137.
- Van Wijngaarden RPA, Van den Brink PJ, Voshaar JHO, Leeuwangh P. 1995. Ordination techniques for analyzing response of biological communities to toxic tress in experimental ecosystems. Ecotoxicology. 4:61–77.
- Welch EB, Jacoby JM, Horner RR, Seeley MR. 1988. Nuisance biomass levels of periphytic algae in streams. Hydrobiologia. 157:161–168.
- Wu N, Schmalz B, Fohrer N. 2011. Distribution of phytoplankton in a German lowland river in relation to environmental factors. J Plankton Res. 33:807–820.
- Yang Y, He Z, Lin Y, Phlips EJ, Yang J, Chen G, Stoffella PJ, Powell CA. 2008. Temporal and spatial variations of nutrients in the ten mile creek of South Florida, USA and effects on phytoplankton biomass. J Environ Monitor. 10:508–516.