ABSTRACT
In 2011, Texas experienced record heat and drought that escalated concerns about environmental flows for rivers. In response to these worries, fishes and water quality were sampled in the Lower Neches River, Texas, from May-August 2012 during continued drought. Potential effects of hydrology on environmental parameters and assemblages of small (seine samples) and large (gillnet samples) fishes were evaluated at multiple locations. Approximately 1 km downstream from the saltwater barrier, paper mill effluent discharges into the river. Salinity was higher and dissolved oxygen lower below the saltwater barrier during low-flow intervals. Fish species richness in seine samples, was higher when the barrier was open, and species richness for both gear types was lowest at sites closest to the paper mill effluent discharge. Overall, species richness was higher at sites below the barrier, with more estuarine and marine species present. When the barrier was closed, richness and abundance of sensitive freshwater species were lower below the barrier. Closure of the barrier during drought results in accumulation of dissolved organic compounds from paper mill effluent and lower dissolved oxygen in the reach below the barrier. To sustain biodiversity in the system, subsistence flows must pass across the saltwater barrier during droughts.
Introduction
Recurrent drought poses a challenge for water planning in semi-arid regions, such as Texas. Most climate models project that droughts will become more severe and frequent in Texas (Allen et al. Citation2011), and this, along with human demand for freshwater, will severely impact surface waters and freshwater inflows to bays and estuaries (Smith & Hunt Citation2010). For rivers that flow directly into the Gulf of Mexico, lower freshwater inflow results in saltwater intrusion that may extend several kilometers upstream into non-tidal reaches. For this reason, saltwater barriers were installed on the lower reaches of several Texas Rivers, including the Neches River in east Texas, the focus of this study. These barriers preserve water quality upstream by preventing saltwater intrusion; however, they also reduce delivery of freshwater to downstream channel reaches and associated freshwater wetlands (Nickerson Citation1998; Winemiller et al. Citation2013).
Many studies have shown a strong influence of salinity gradients on fish assemblages in coastal streams. For example, Martino and Able (Citation2003) identified salinity as one of the most important environmental factors affecting the fish assemblage of the Mullica River in New Jersey. An increase in salinity can change relative abundances of freshwater and marine species, local recruitment, predator–prey interactions, and abundance of migratory estuarine fishes that inhabit brackish coastal habitats during a portion of their life cycle (Garcia et al. Citation2003a, Citation2003b). Reduced freshwater flows can also lead to greater concentrations of pollutants in receiving water bodies. Below the saltwater barrier, the Lower Neches River receives effluent from the MeadWestvaco paper mill in Evadale, Texas (permitted to discharge 65 million gallons per day), that is diluted with river flow that carries it to the coast. Reductions in river discharge reduce dilution and transport of pollutants. Paper mill effluent contains high concentrations of dissolved organic matter that is difficult to treat, and when aquatic systems are overloaded, this material can induce high biochemical and chemical oxygen demand (BOD and COD, respectively) (Antony et al. Citation2012). High BOD can reduce dissolved oxygen concentration to levels harmful to many aquatic organisms (Lima Neto et al. Citation2007).
Texas experienced the hottest and driest year on record in 2011, and subsequent to precipitation events during December–March 2012, drought conditions persisted throughout the remainder of 2012. The present study documents spatiotemporal variation of water quality and fish assemblages in the Lower Neches River at survey locations above and below the saltwater barrier on the Lower Neches River. We address two specific questions: (1) To what degree does fish species richness, abundance and local assemblage structure differ during periods when the barrier is open or closed? and (2) To what degree does closure of the barrier and reduction of freshwater flows influence water quality and fish assemblages in the downstream reach? We predicted that reduced freshwater flow below the barrier would result in reduced dissolved oxygen concentrations owing to biological oxygen demand from high concentrations of dissolved organic matter in paper mill effluent as well as increased salinity from saltwater intrusion from the receiving bay system, Sabine Lake. During periods of low flow when the saltwater barrier was closed to prevent saltwater intrusion, fish assemblages below the barrier were expected to have lower diversity and altered composition that included more marine species and freshwater species tolerant of high salinity and poor water quality.
Materials and methods
Study system
The Neches River flows from Van Zandt County, Texas, to Sabine Lake, a shallow, brackish lake that is connected to the Gulf of Mexico. The Neches watershed is dominated by agriculture, forestry, and forest preserves, including the US National Park Service's Big Thicket Preserve, a network comprising nine separate conservation units and six water corridors. Flow of the Neches River has been altered by the operation of two major reservoirs, Lake Palestine and B.A. Steinhagen Reservoir. The Port of Beaumont shipping channel runs from the Neches River near the southern limit of Beaumont through Sabine Lake to the Gulf of Mexico. Deepening of this channel in the 1940s resulted in greater intrusion of the saltwater wedge, which threatened Beaumont's municipal water supply. Subsequently, a temporary saltwater barrier was placed on the Neches River near the northeastern limit of city of Beaumont, Texas, and in 2003, the US Army Corps of Engineers and the Lower Neches Valley Authority completed construction of a permanent saltwater barrier ( and ). The barrier has gates that can be raised to allow relatively unimpeded flow during periods of high discharge, and lowered to block saltwater intrusion during periods of low flow. Our study sites were located in a reach of the Lower Neches River bordered by the city of Beaumont to the west, and bordered to the east by the Beaumont Unit of the Big Thicket Preserve, a wetland containing diverse vegetation assemblages including bottomland hardwood swamps dominated by bald cypress (Taxodium distichum) and water tupelo (Nyssa aquatica). Located within the study reach are the saltwater barrier and the point of discharge from the canal carrying MeadWestvaco paper mill effluent ().
Figure 1. Top photo – Neches River channel upstream from the saltwater barrier; bottom photo – saltwater barrier on Lower Neches with gates in open position.
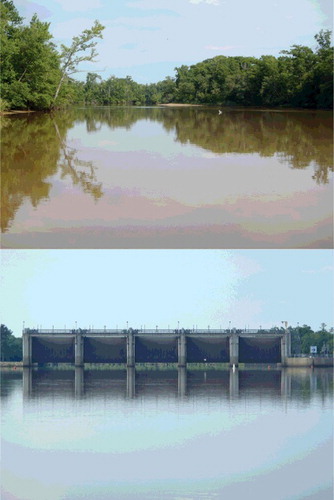
Figure 2. Seine (circles) and gillnet and water quality sites (both represented by triangles) during summer 2012. Differences in water quality (optics) above the saltwater barrier, below the saltwater barrier, and within the MeadWestvaco paper mill effluent delivery canal and rectangular collecting pond witin the Beaumont Unit are apparent in this GoogleEarth® image.
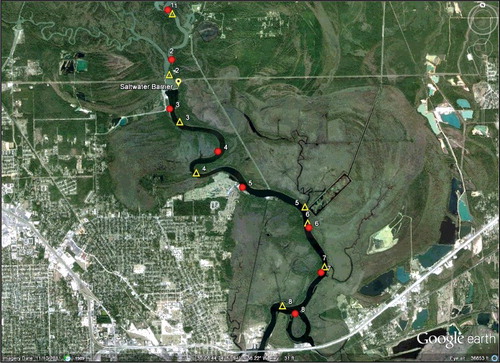
Field surveys
Fishes and water quality parameters were surveyed along the Neches River at localities above and below the saltwater barrier from May–August 2012, which are the warm summer months (). Sixteen sites were sampled over four days during each of four sampling months (16–19 May, 20–23 June, 18–22 July, 22–25 Aug.). Two methods were employed in order to assess relative abundance patterns of small fishes within shallow littoral habitats (seine surveys) and larger fishes within deeper areas (gillnet surveys). At eight sites, seine samples were taken between 1000 and 1200 h. Each monthly seine survey included intervals of rising and falling tides, except for May when tides were rising during the mornings when seine surveys were conducted; and diel tidal amplitude was low within the study reach (<0.3 m) during each of the monthly surveys. At the other eight sites, water quality data were collected between 0700 and 1000 h and gillnets were deployed at 1700 h and retrieved the next day at approximately 0800 h (each encompassing one tidal cycle). Seine sites were located near the shore in relatively shallow areas (<1.5 m), and water quality/gillnet sites were located near shore in deeper parts of the river channel. Four sites (two seine sites, two water quality/gillnet sites) were above the saltwater barrier, and twelve sites (six seine sites, six water quality/gillnet sites) were below the barrier. Six sites (three seine sites, three water quality/gillnet sites) were located below the MeadWestvaco paper mill effluent discharge canal ().
Multiple contiguous hauls were performed at the seine sites. The distance of each haul (3-m × 6-m seine with 0.3 cm mesh) was estimated in meters. The number of seine hauls and total distance of hauls per site depended on the area accessible for seining. These data were standardized as catch-per-unit effort (CPUE, number of individual fish or species obtained per meter of seine haul using the same seine). Four 38.1-m × 2.4-m experimental gillnets (each with five panels: 1.3-, 2.5-, 5.1-, 7.6-, and 8.9-cm bar mesh) were deployed at each site where water quality was measured. Gillnet data were standardized as CPUE (number of individuals or species per hour of deployment per 10 m of gillnet). Large fish specimens obtained by either method were identified in the field and released; small- and medium-sized specimens were anesthetized using tricaine methanesulfonate (MS-222), preserved in 10% formalin, and later transferred to 70% ethanol. Preserved specimens were sorted and identified to species (or, for immature specimens, lowest feasible taxonomic level) in the laboratory.
Environmental variables
To characterize water quality, pH was measured using a portable digital meter, and temperature (°C), DO (mg/L), salinity (ppt), and conductivity (μS/cm) were recorded using a Yellow Springs Instruments (YSI) model 85 multiprobe/meter. Water depth was measured using a metric pole. Lower Neches River discharge data for the study period were obtained from United States Geological Survey gauge #08041780 near the city of Beaumont. The saltwater barrier was open during the May and July surveys and closed during June and August surveys. We used two-way repeated-measures analysis of variance (ANOVA) to compare values of environmental variables when the saltwater barrier was open with those when the barrier was closed (hereafter referred to as ‘barrier periods’). Barrier period was included as a factor, and site nested within month as the error term. Before running the ANOVA, we loge(x)-transformed pH, DO, specific conductivity, and temperature, and loge(x + 1)-transformed salinity. We used R software for all statistical analyses.
Analysis of species richness, abundance and assemblage structure
For each survey location, species richness (standardized as the total number of species per 10 m of habitat seined for seine samples, and as the number of species per 10 m of gillnet per hour of deployment for gillnet samples), standardized abundance (CPUE) of individual species and fishes overall, and species relative abundances (% of total number of individuals), we recalculated for seine and gill net samples separately. We investigated whether barrier periods yielded differences in fish species richness and total CPUE using two-way repeated measures ANOVA. Again, we included barrier period as a factor, with site nested within month as the error term. We analyzed gillnet and seine samples separately, and log-transformed the CPUE data before running ANOVAs. We also classified species as freshwater, estuarine or marine ( and ) according to the guild scheme for estuarine fishes proposed by Elliot et al. (Citation2007). Freshwater fishes include freshwater migrants and freshwater stragglers. Estuarine fishes include estuarine residents, migrants and putative amphidromous species. All marine species were marine migrants, and no species was identified as a marine straggler.
Table 1. Species % relative abundances for gillnet samples from the eight sites in the Neches River, Texas (F = freshwater, E = Estuarine, M = marine).
Table 2. Species % relative abundances for seine samples from the eight sites in the Neches River, Texas (F = predominantly freshwater, E = Estuarine, M = marine).
We examined associations between fish assemblage structure based on gillnet CPUE and water quality variables, daily mean discharge, barrier period (open vs. closed), and saltwater barrier location (i.e. above vs. below the saltwater barrier) using the vegan package in R. We first plotted gillnet CPUE in multivariate space using principal components analysis (PCA). We then fitted environmental variables and used permutation tests to assess their statistical significance using the envfit function. Next, we ran another PCA using seine CPUE and used the envfit function to assess whether daily mean discharge, barrier period and location had significant influences on fish assemblage structure. All continuous variables were log-transformed before analyses.
Results
Environmental variables
During summer 2012, Neches River flow was greater when the barrier was open (May average = 111 m3/s, July average = 429 m3/s) than closed (June average = 81 m3/s, August average = 134 m3/s) for preventing saltwater intrusion (). With a 5% type-I error threshold (P = 0.05), barrier period did not have a statistically significant effect on any of the environmental variables tested; however, statistically marginal results were observed for salinity (F1,4 = 11.68, P = 0.08), specific conductivity (F1,4 = 15.87, P = 0.06) and temperature (F1,4 = 6.25, P = 0.13). When the saltwater barrier was open, salinity for the six downstream sites averaged 0.06 ppt in May and 0.04 ppt in July. By comparison, when the saltwater barrier was closed, salinity averaged 0.31 ppt in June and 0.59 ppt in August. Similarly, average specific conductivity for the six sites was lower when the barrier was open (May = 192 μS/cm, July = 146 μS/cm) than when it was closed (June = 672 μS/cm, August = 1259 μS/cm). Average temperature also tended to be lower when the barrier was open (May = 26.6 °C, July = 28.2 °C) than when it was closed (June = 29.3 °C, August = 29.8 °C). When the saltwater barrier was closed, the two sites above the barrier had higher DO concentrations and lower specific conductivity than the sites below the barrier (June average DO above barrier = 6.5 mg/L, specific conductivity = 181 μS/cm; June average DO below barrier = 5.0 mg/L, specific conductivity = 839 μS/cm; August average DO above barrier = 5.4 mg/L, specific conductivity = 149 μS/cm; August average DO below barrier = 4.9 mg/L, specific conductivity = 1629 μS/cm).
Figure 3. Hydrograph showing mean daily discharge in the Neches River during the study period (2012) and mean daily discharge for April–August based on 2002–2015 records. Data from United States Geological Survey gauge #08041780 (Beaumont Saltwater Barrier) are adjusted for tidal influence.
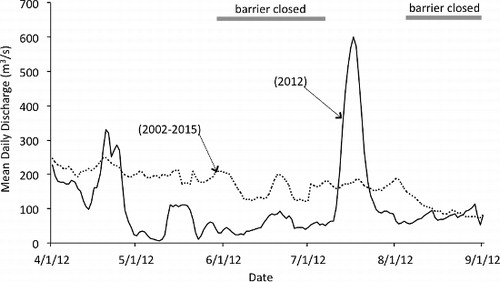
Paper mill effluent discharge affected water quality variables in the river. During June, July, and August surveys, the site directly adjacent to the canal discharge had higher salinity, lower DO, and higher specific conductivity than sites above and further below the canal discharge. For example, during July, DO concentration at the site adjacent to the canal discharge was 2.4 mg/L, and average DO at other sites was 3.3 mg/L.
Species richness and abundance
A total of 489 specimens (representing 38 species) were collected from gillnets. Gillnet samples were dominated by gars, gizzard shad, smallmouth buffalo, and blue catfish (). Twelve of the 38 species obtained in gillnet surveys were only captured during one month, whereas nine species were present during every month. Fish species richness of gillnet samples was higher below that barrier than above the barrier during every month and was not affected by barrier closure (). CPUE for gillnets was higher during the two months of barrier closure (). Percentages of freshwater and estuarine+marine fishes captured in gillnets did not vary greatly during periods when the barrier was closed or open, but freshwater fishes dominated gillnet catches at the two upstream sites, and estuarine and marine fishes dominated gillnet catches in the downstream reach ().
Table 3. Fish species richness and average catch-per-unit effort for gillnet and surveys at two sites located above the saltwater barrier on the Lower Neches River and six sites below the saltwater barrier during months when the barrier was either open or closed (CPUE for gillnet samples is no. of specimens/10 m/h based on a standard experimental gillnet; CPUE for seine samples is no. of specimens/m based on a standard seine).
Table 4. Percent relative abundance of freshwater versus estuarine plus marine fishes from gillnet and seine surveys conducted when the saltwater barrier on the Lower Neches River was open or closed and at sites above or below the barrier.
A total of 27,180 specimens (representing 57 species) were collected with the seine. Seine samples were dominated by bay anchovy and species from the families Cyprinidae, Clupeidae, and Centrarchidae (). The effect of barrier period on fish species richness of seine samples was statistically non-significant, but only marginally, probably owing to few degrees of freedom (F1,4 = 12.6, P = 0.07). Fish abundance (CPUE) in seine samples () varied with barrier period (F1,4 = 104.4, P < 0.01), but this result was strongly influenced by the May sample that had large abundance of Gulf menhaden juveniles, a marine clupeid that exploits regional estuaries as nursery habitat during January–May. When the saltwater barrier was closed, the two sites below the barrier had higher species richness and CPUE of seine samples than the six sites below the barrier (). When the barrier was closed and freshwater flow was reduced, proximity to the paper mill effluent discharge appeared to have influenced species richness and abundance of seine samples. In June, species richness at the site directly adjacent to the canal was 6 and CPUE was 0.15 individuals/m. The next two closest sites above and below the effluent discharge had an average species richness of 9 (st dev = 3) and average CPUE of only 0.21 individuals/m (st dev = 0.04). Similarly, in August, species richness at the site adjacent to the canal was 4 and CPUE was just 0.10 individuals/m, and the next two sites above and below the canal had an average species richness of 5 (st dev = 0) and average CPUE of 0.17 individuals/m (st dev = 0.05). Percentages of freshwater and estuarine+marine fishes captured in the seine also did not vary greatly during periods when the barrier was closed or open (). Freshwater fishes dominated seine catches at both upstream and downstream sites, but with a greater percentage of freshwater species at sites above the saltwater barrier ().
Assemblage structure in relation to environmental gradients
Water quality variables varied according to barrier period, with specific conductivity, salinity, and temperature lower when the saltwater barrier was open, and as a consequence, barrier period was strongly associated with assemblage structure of gillnet captures. The first axis from PCA of gillnet CPUE data accounted for 51% of the total variance, and the second axis accounted for less than 10%. Permutation tests revealed that DO (F = 130.7, P < 0.01), temperature (F = 70.0, P < 0.01), salinity (F = 52.8, P < 0.01), pH (F = 51.1, P < 0.01), specific conductivity (F = 9.7, P < 0.05), depth (F = 6.5, P < 0.05), and flow (F = 4.0, P < 0.05) were associated with variation in fish assemblage structure based on gillnet data. The first PCA axis mainly contrasted assemblages from sites with low DO, including the four sites nearest the MeadWestvaco paper mill effluent delivery canal, with assemblages from sites with higher DO (). The second PCA axis contrasted assemblages from sites with high specific conductivity, salinity, and temperature with those from sites having lower specific conductivity, salinity, and temperature ().
Figure 4. The first two axes from principal components analysis (PCA) of gillnet catch-per-unit effort (CPUE) data in the Neches River. Dots indicate locations of site surveys in ordination space. In the top plot, dot shades indicate whether the saltwater barrier was open or closed when samples were collected and near or distant from the MeadWestvaco paper mill effluent discharge; in the bottom plot, dot shades indicate whether samples were collected above the saltwater barrier, below the barrier and distant from the paper mill effluent discharge, or below the barrier and near the paper mill effluent discharge. Locations of text reveal species (text containing first two letters of the genus name and first two letters of the species name) loadings on each axis and correlations of environmental variable (text at vector tips) with each axis.
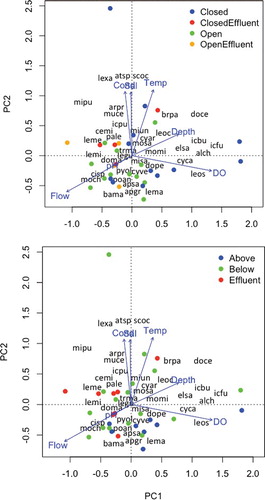
Seven of the thirty-eight species were exclusive to gillnet samples taken when the barrier was open (May or July), and seven species were exclusive to samples taken when the barrier was closed (June or August, ). Only four of the 38 species obtained in gillnet surveys were exclusive to sites above the barrier, whereas 21 were exclusive to sites below the barrier (). Certain species, such as pirate perch, blacktail shiner, common carp, and flathead catfish, were only captured in gillnets at sites above the barrier, whereas others, including alligator gar, channel catfish, sheepshead, red drum, and white bass, were only captured below the barrier. The site directly adjacent to the paper mill effluent delivery canal, which had poor water quality, was dominated by alligator gar, spotted bass, and mullet.
The first axis from PCA of seine CPUE data explained 42% of the total variation, and the second axis explained less than 10%. Permutation tests indicated that saltwater barrier period (F = 11.6, P < 0.01) and location relative to the saltwater barrier (F = 11.2, P < 0.01) had significant correlations with fish assemblage structure based on seine samples (). Flow had a marginal statistical association with fish assemblage structure (F = 2.3, P = 0.07). Samples taken when the barrier was closed had different assemblage structures than samples taken when the barrier was open (). When the barrier was closed, seine samples taken below the barrier were more similar to each other than to samples taken above the barrier. PCA of seine CPUE data revealed a clear difference in assemblage structure of samples taken above and below the barrier, regardless of whether the barrier was open or closed. Samples from above and below the barrier had no overlap in location in PCA ordination space.
Figure 5. The first two axes from PCA of seine CPUE data in the Neches River. Dots indicate locations of site surveys in ordination space. In the top plot, dot shades indicate whether the saltwater barrier was open or closed when samples were collected and near or distant from the MeadWestvaco paper mill effluent discharge; in the bottom plot, dot shades indicate whether samples were collected above the saltwater barrier, below the barrier and distant from the paper mill effluent discharge, or below the barrier and near the paper mill effluent discharge. Locations of text reveal species (text containing first two letters of the genus name and first two letters of the species name) loadings on each axis and correlations of environmental variable (text at vector tips) with each axis.
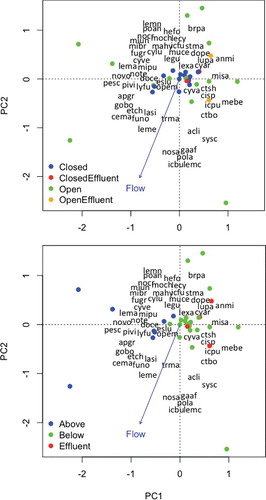
Among seine samples, 23 of 54 species were captured only when the saltwater barrier was open, whereas only two species were exclusively captured when the saltwater barrier was closed (). When the saltwater barrier was open, freshwater flow was higher, and therefore many of the species that were exclusively captured when the barrier was open were freshwater species. For example, smallmouth buffalo, several minnow species (e.g. shoal chub, golden shiner, and Sabine shiner), and several species of sunfish (flier, green sunfish, warmouth, redear sunfish, and redspotted sunfish) were only captured when the saltwater barrier was open. Only ladyfish and spot, marine species tolerant of high salinity, were exclusively captured when the saltwater barrier was closed.
CPUE and relative abundance data reveal that 10 species were exclusive to seine sites above the barrier, whereas 16 species were exclusive to sites below the barrier, regardless of whether the barrier was open or closed. Species solely captured above the saltwater barrier tended to be freshwater species, such as smallmouth buffalo, minnows (shoal chub, Sabine shiner, and mimic shiner), sunfish (flier), and darters (bluntnose darter and dusky darter). Species solely captured below the barrier were mainly marine and estuarine species, including sheepshead minnow, gulf killifish, rainwater killifish, and sailfin molly. Seine samples collected from the site nearest to the paper mill effluent discharge were dominated by bay anchovy (marine fish), blacktail shiner, threadfin shad, and inland silverside (freshwater fishes).
Discussion
Drought conditions from October 2010 through September 2011 resulted in the driest 12-month period in Texas’ recorded history (Nielsen-Gammon Citation2012). This lack of precipitation resulted in extreme low flows in the Neches River until rain fell during late November 2011. During 2011, salinity levels increased substantially below the Lower Neches saltwater barrier, and lack of instream flow allowed dissolved organic matter from the MeadWestvaco paper mill to accumulate in this region of the river. Water quality measurements taken just above and below the saltwater barrier revealed a large difference between these two segments of the river during summer and fall 2011, a period of extreme low flows and closure of the saltwater barrier (Winemiller et al. Citation2013), and this water quality difference is clearly visible as color differences in the GoogleEarth® aerial photo taken on 10 November 2011 (). Fish surveys in the reach below the saltwater barrier during October–November 2011 only yielded species, such as gars and mullets, tolerant of low DO and high concentrations of dissolved organic compounds as well as brackish and saline conditions (Winemiller et al. Citation2013). Those samples also lacked minnows, sunfishes, and other freshwater species intolerant of salinity. The lowest DO measurement was obtained just below the barrier during November 2011, indicating that saltwater intrusion affected the entire reach below the barrier. Lack of dilution of the paper mill effluent would have increased biological and chemical oxygen demands, thereby reducing DO levels (Harrel & Smith Citation2002; Lima Neto et al. Citation2007). Harrel and Smith (Citation2002) reported DO levels <2 mg/L at 5 m depth at two survey sites located nearest to the Mead Westvaco paper mill outfall on the Lower Neches River on August 1999, a period of low flow. Other sites above and below this reach had greater DO concentrations, providing evidence that biological oxygen demand associated with the waste load had degraded water quality. They found that water quality and biological integrity as indicated by benthic invertebrate assemblages improved between the early1970s and mid-1980s concordant with reductions in permitted waste load and reductions in toxic substances following implementation the US Clean Water Act. They also found that between 1985 and 1999, biological integrity was unchanged while the permitted waste load increased 19.5%.
Between December 2011 and May 2012, rainfall had reestablished sufficient flows in the Lower Neches to allow gates of the saltwater barrier to remain open most of the time. Drought conditions recurred in summer 2012. During this period, seine samples taken while the barrier was open had significantly higher fish species richness than samples taken while the barrier was closed. Overall, samples taken below the barrier had more species than samples taken above the barrier, and this was due to greater numbers of estuarine and marine species at downstream sites. This pattern held for gillnet samples that captured mostly larger fishes from deeper habitats as well as seine samples dominated by small fishes from littoral habitats. Multivariate analysis revealed distinct assemblage patterns at sites above and below the barrier across all months. Certain freshwater species, such as mimic shiner, dusky darter and freshwater drum, were confined to sites above the saltwater barrier during every month, and other species were primarily found at sites above the barrier when it was closed and were collected below the barrier only when it was open. Thus, the saltwater barrier might actually block dispersal by certain fish species regardless of whether the barrier is open or closed.
The overall spatial trend in species assemblage structure during summer 2012 differed from findings we obtained during preliminary fish surveys during 2011 (Winemiller et al. Citation2013) and results from earlier studies involving other taxa within the same river reach. Harrel (Citation1975) analyzed water quality and assemblages of benthic macroinvertebrates of the Lower Neches above and below a pair of temporary saltwater barriers that had been installed for the same purpose as the permanent barrier installed in 2003 and found lower water quality and species richness below the barriers compared to sites above them. Harrel and Smith's (Citation2002) study revealed higher species richness at sites below the barriers, except for the two sites closest to the paper mill outfall that continued to have high biological oxygen demand (6040 kg/d) from the waste load. Similar results were observed for fishes in our seine samples during summer 2012; regardless of whether the saltwater barrier was open or closed, species richness was lowest with a fairly distinct assemblage composition at sites closest to the location of the paper mill outfall. Although there seems to be general improvement in water quality between Harrel and Smith's studies and the current study, our results suggest that discharge of paper mill effluent continues to impact the aquatic biota, especially during periods with low instream flows when DOC accumulates in the aquatic ecosystem.
Variation in assemblage structure in relation to location along the fluvial gradient and survey period tended to be smaller for gillnet samples compared to seine samples. Gillnet samples were dominated by large species, many of which were predatory (longnose and spotted gars, channel and blue catfish, largemouth and spotted bass) and also tolerant of brackish conditions, low DO and high concentrations of DOC (Linam & Kleinsasser Citation1998; Roach & Winemiller Citation2011). A possible explanation for lower variation among gillnet samples is that larger fish are capable of moving greater distances over short time periods (Lonzarich et al. Citation1998; Hubert et al. Citation2012). Larger fish typically have larger home ranges (the area visited over a period of several days) presumably due to their higher energy demands that require foraging over larger areas (Gerking Citation1953; Lonzarich et al. Citation1998; Kramer & Chapman Citation1999). Furthermore, larger fish are less susceptible to predation than smaller fish, so that the former probably have less need to remain within or near structurally complex habitats that provide refuge from predators (Mittelbach Citation1981; Schlosser Citation1987; Chick & Mlvor Citation1997).
Closure of the saltwater barrier seemed to affect the longitudinal pattern of fish species richness and assemblage structure. Species richness of freshwater fishes in seine samples was the same at sites above and immediately below the barrier during the month of May when the barrier was open and flows were relatively high; however, sites further downstream (i.e. closer to Sabine Lake) were increasingly dominated by estuarine and marine fishes. Certain freshwater species, such as redear sunfish and white crappie, were only collected during May (following an interval of high flows), and several other freshwater species, such as red shiner and largemouth bass, had broader spatial distributions during May than any other month. Although salinity also was low during July, the other survey period when the barrier was open, several species may have been absent or rare below the barrier due to suboptimal DO concentrations (McKinsey & Chapman Citation1998; Kramer & Chapman Citation1999; Stevens et al. Citation2006).
Relationships between salinity gradients and fish assemblage structure have been observed in estuarine ecosystems worldwide (Keup & Bayless Citation1964; Peterson & Ross Citation1991; Garcia et al. Citation2003b; Martino & Able Citation2003; Whitfield et al. Citation2006). Variation in precipitation and runoff can shift longitudinal spatial patterns of salinity gradients and assemblage structure (Garcia et al. Citation2003a; Love et al. Citation2008; Vivier et al. Citation2010; Zampatti et al. Citation2010). Coastal streams and estuaries play important roles in recruitment of marine species that depend on estuarine gradients and access to oligohaline habitats (Rogers et al. Citation1984; Akin et al. Citation2003; Elliot et al. Citation2007; Potter et al. Citation2015), and chronic high salinities may have detrimental effects on these marine populations (Roessig et al. Citation2004; Dolbeth et al. Citation2008). In addition to closure of the saltwater barrier, saltwater intrusion in the Lower Neches may have significant impacts on estuarine-dependent species of this region, such as Gulf menhaden, red drum, and bay whiff. These fishes depend on oligohaline coastal ecosystems for optimal juvenile growth and survival, as well as production of crustacean populations that are important food resources (Deegan Citation1990; Reichert & van der Veer Citation1991; Raynie & Shaw Citation1994; Craig et al. Citation1995; Roessig et al. Citation2004).
The Lower Neches River, like all estuaries, is a dynamic system with variable flows, salinity and local fish assemblages comprising freshwater, estuarine and marine species that use different channel reaches during different periods under different conditions for different purposes. Even so, species vary in their ability to cope with extreme abiotic environmental conditions, such as high salinity or aquatic hypoxia, and consequently human impacts can stress the adaptive capacity of native biota. In the Lower Neches River, lack of precipitation and flows degraded water quality in reaches below the saltwater barrier during 2011–2012, including the period of our field surveys. The saltwater barrier on the Lower Neches protects upstream water users from increases in salinity when flows are low, but at the same time reduces instream flows in the reach below the barrier and inhibits longitudinal movements by freshwater, estuarine and marine fishes alike. The current state water plan for Texas (TWDB Citation2016) proposes new water diversions from streams and rivers, especially from the Neches and other rivers in the relatively water-rich, eastern portion of Texas, which would further reduce instream flows. Should this occur, the Neches River fish fauna in the reach below the saltwater barrier would become strongly dominated by estuarine and marine fishes, and water quality and aquatic biodiversity in the Lower Neches River could decline further. Given the lack of downstream flushing of paper mill effluent in the Lower Neches during times of drought and barrier closure, the current permit for allowable daily discharge from the paper mill should be re-evaluated in light of saltwater barrier operation with regard to recent and projected future hydrology. To protect environmental quality, aquatic biodiversity and ecosystem services, allowable discharge should be reduced during droughts.
Acknowledgments
The authors thank D. Roemer, J. Cummings, and B. Lockwood for valuable advice and collaboration in setting up this study. We also thank N. Lujan, C. Montana, D. Fitzgerald, M. Libson, and C. Lozada for assistance in data collection and laboratory analysis.
Disclosure statement
No potential conflict of interest was reported by the authors.
Additional information
Funding
Notes on contributors
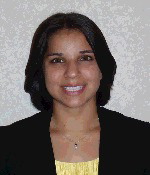
Rebecca I. Pizano-Torres
Rebecca I. Pizano-Torres received a Master's degree from Texas A&M University where she studied effects of hydrology on water quality and biotic indicators. She currently is a water manager in Houston, TX.
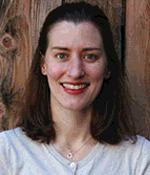
Katherine A. Roach
Katherine A. Roach teaches biology at La Crescent Montessori Academy & STEM School. She received her doctorate from Texas A&M University and was a postdoctoral researcher at the University of Québec at Trois-Rivières. She has conducted research on river ecology in six countries, with a principal focus on food web dynamics and fish community ecology.
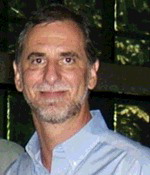
Kirk O. Winemiller
Kirk O. Winemiller is a regents professor at Texas A&M University. Over the past 35 years, he has conducted research with his students and other collaborators on the ecology of fishes and food webs of rivers and estuaries in several regions of the world.
References
- Akin S, Winemiller KO, Gelwick FP. 2003. Seasonal and spatial variations in fish and macrocrustacean assemblage structure in Mad Island Marsh estuary, Texas. Estuar Coast Shelf Sci. 57:269–282.
- Allen PM, Harmel RD, Dunbar JA, Arnold JG. 2011. Upland contribution of sediment and runoff during extreme drought: a study of the 1947–1956 drought in the Blackland Prairie, Texas. J Hydrol. 407:1–11.
- Antony A, Bassendeh M, Richardson D, Aquilina S, Hodgkinson A, Law I, Leslie G. 2012. Diagnosis of dissolved organic matter removal by GAC treatment in biologically treated papermill effluents using advanced organic characterisation techniques. Chemosphere. 86:829–836.
- Chick J, Mlvor C. 1997. Habitat selection by three littoral zone fishes: effects of predation pressure, plant density and macrophyte type. Ecol Freshw Fish. 6:27–35.
- Craig SR, Neill WH, Gatlin DM, III. 1995. Effects of dietary lipid and environmental salinity on growth, body composition, and cold tolerance of juvenile red drum (Sciaenops ocellatus). Fish Physiol Biochem. 14:49–61.
- Deegan LA. 1990. Effects of estuarine environmental conditions on population dynamics of young-of-the-year gulf menhaden. Mar Ecol Prog Ser. 68:195–205.
- Dolbeth M, Martinho F, Viegas I, Cabral H, Pardal M. 2008. Estuarine production of resident and nursery fish species: conditioning by drought events? Estuar Coast Shelf Sci. 78:51–60.
- Elliott M, Whitfield AK, Potter IC, Blaber SJM, Cyrus DP, Nordlie FG, Harrison TD. 2007. The guild approach to categorizing estuarine fish assemblages: a global review. Fish Fish. 8:241–268.
- Garcia AM, Raseira MB, Vieira JP, Winemiller KO, Grimm AM. 2003b. Spatiotemporal variation in shallow-water freshwater fish distribution and abundance in a large subtropical coastal lagoon. Environ Biol Fish. 68:215–228.
- Garcia AM, Vieira JP, Winemiller KO. 2003a. Effects of 1997–1998 El Niño on the dynamics of the shallow-water fish assemblage of the Patos Lagoon Estuary (Brazil). Estuar Coast Shelf Sci. 57:489–500.
- Gerking SD. 1953. Evidence for the concepts of home range and territory in stream fishes. Ecology. 34:347–365.
- Harrel RC. 1975. Water quality and salt water intrusion in the Lower Neches River. Texas J Sci. 26:107–117.
- Harrel RC, Smith ST. 2002. Macrobenthic community structure before, during, and after implementation of the Clean Water Act in the Neches River estuary (Texas). Hydrobiologia. 474:213–222.
- Hubert WA, Pope KL, Dettmers JM. 2012. Passive capture techniques. In: Zale AV, Parrish DL, Sutton TM, editors. Fisheries techniques. Bethesda (MD): American Fisheries Society; p. 223–265.
- Keup L, Bayless J. 1964. Fish distribution at varying salinities in Neuse River basin, North Carolina. Chesapeake Sci. 5:119–123.
- Kramer DL, Chapman MR. 1999. Implications of fish home range size and relocation for marine reserve function. Environ Biol Fish. 55:65–79.
- Lima Neto IE, Zhu DZ, Rajaratnam N, Yu T, Spafford M, McEachern P. 2007. Dissolved oxygen downstream of an effluent outfall in an ice-covered river: natural and artificial aeration. J Environ Eng. 133:1051–1060.
- Linam G, Kleinsasser L. 1998. Classification of Texas freshwater fishes into trophic and tolerance groups. Austin (TX): Texas Parks and Wildlife Press. ( River studies report).
- Lonzarich DG, Warren J, Melvin L, Lonzarich MRE. 1998. Effects of habitat isolation on the recovery of fish assemblages in experimentally defaunated stream pools in Arkansas. Can J Fish Aquat Sci. 55:2141–2149.
- Love JW, Gill J, Newhard JJ. 2008. Saltwater intrusion impacts fish diversity and distribution in the Blackwater River drainage (Chesapeake Bay watershed). Wetlands. 28:967–974.
- Martino EJ, Able KW. 2003. Fish assemblages across the marine to low salinity transition zone of a temperate estuary. Estuar Coast Shelf Sci. 56:969–987.
- McKinsey DM, Chapman LJ. 1998. Dissolved oxygen and fish distribution in a Florida spring. Environ Biol Fish. 53:211–223.
- Mittelbach GG. 1981. Foraging efficiency and body size: a study of optimal diet and habitat use by bluegills. Ecology. 62:1370–1386.
- Nickerson BA. 1998. Trans-Texas water program, southeast area: environmental analysis for the Neches salt water barrier Beaumont, Texas. Fort Worth (TX): Freese and Nichols, Inc.
- Nielsen-Gammon JW. 2012. The 2011 Texas drought. Texas Water J. 3:59–95.
- Peterson MS, Ross ST. 1991. Dynamics of littoral fishes and decapods along a coastal river-estuarine gradient. Estuar Coast Shelf Sci. 33:467–483.
- Potter IC, Tweedley JR, Elliot M, Whitfield AK. 2015. The ways in which fish use estuaries: a refinement and expansion of the guild approach. Fish Fish. 16:230–239.
- Raynie RC, Shaw RF. 1994. A comparison of larval and postlarval gulf menhaden, Brevoortia patronus, growth rates between an offshore spawning ground and an estuarine nursery. Fish Bull. 92:890–894.
- Reichert MJ, van der Veer HW. 1991. Settlement, abundance, growth and mortality of juvenile flatfish in a subtropical tidal estuary (Georgia, USA). Netherlands J Sea Res. 27:375–391.
- Roach KA, Winemiller KO. 2011. Diel turnover of assemblages of fish and shrimp on sandbanks in a temperate floodplain river. Trans Am Fish Soc. 140:84–90.
- Roessig JM, Woodley CM, Cech JJ, Jr, Hansen LJ. 2004. Effects of global climate change on marine and estuarine fishes and fisheries. Rev Fish Biol Fish. 14:251–275.
- Rogers SG, Targett TE, Van Sant SB. 1984. Fish-nursery use in Georgia salt-marsh estuaries: the influence of springtime freshwater conditions. Trans Am Fish Soc. 113:595–606.
- Schlosser IJ. 1987. The role of predation in age-and size-related habitat use by stream fishes. Ecology. 68:651–659.
- Smith BA, Hunt BB. 2010. A comparison of the 1950s drought of record and the 2009 drought, Barton Springs segment of the Edwards Aquifer, Central Texas. Gulf Coast Assoc Geol Soc Trans. 60:611–622.
- Stevens PW, Blewett DA, Casey JP. 2006. Short-term effects of a low dissolved oxygen event on estuarine fish assemblages following the passage of Hurricane Charley. Estuar Coast. 29:997–1003.
- TWDB. 2016. Water for Texas: 2017 state water plan. Austin (TX): Texas Water Development Board. Available from: http://www.twdb.texas.gov/waterplanning/swp/2017/index.asp
- Vivier L, Cyrus DP, Jerling HL. 2010. Fish community structure of the St Lucia Estuarine System under prolonged drought conditions and its potential for recovery after mouth breaching. Estuar Coast Shelf Sci. 86:568–579.
- Whitfield AK, Taylor RH, Fox C, Cyrus DP. 2006. Fishes and salinities in the St Lucia estuarine system–a review. Rev Fish Biol Fish. 16:1–20.
- Winemiller KO, Pizano R, Montaña CG. 2013. Freshwater flow recommendations to mitigate effects of climate change to the Lower Neches River and Beaumont Unit of the Big Thicket Preserve. College Station (TX): Final Project Report for the US National Park Service.
- Zampatti BP, Bice CM, Jennings PR. 2010. Temporal variability in fish assemblage structure and recruitment in a freshwater-deprived estuary: the Coorong, Australia. Mar Freshw Res. 61:1298–1312.