ABSTRACT
Wetland acreage has declined continentally in the USA. With the loss of natural wetlands, farmed wetlands may be a surrogate for natural wetlands. Seasonally, flooded rice fields are commonly managed wetlands on the Gulf of Mexico Coastal Plain. This study had two objectives: (1) catalog arthropod diversity in rice fields; (2) investigate if detrital subsidies could elicit trophic cascades that reduce nuisance organisms. In 2013 and 2014, experimental rice plots were established, and detritus was applied to half of the plots in each year. Floating pitfall traps, aquatic D-net sweeping, Gee crawfish traps and root/sediment corers were used to sample for arthropods. Over the two growing seasons, 143 different species were sampled from 13 orders, totaling 49,251 individuals. Detrital subsidies neither elicited a detectable trophic cascade nor did they significantly alter the rice field arthropod community. Contrary to previous studies, results suggest that macroinvertebrate communities in farmed wetlands are resilient to alterations possibly due to the long-term agricultural use.
Introduction
Wetlands are habitats for diverse fauna and flora (Hook Citation1993; Lawler Citation2001), and provide many ecosystem services, and thus, are a conservation priority (O'Malley Citation1999; Zedler & Kercher Citation2005). Wetlands acreage in the USA has declined, with an estimated loss of 260,700 ha from 1986 to 1997, 26% of which was attributed to conversion of wetland to agriculture (Dahl Citation2000). Farmed wetlands, specifically rice and crayfish ponds, are a potential surrogate for natural wetlands, especially in regions where natural wetlands are less common (Lawler Citation2001; Huner et al. Citation2002; Richardson & Taylor Citation2003; Manley et al. Citation2004; Wilson et al. Citation2008; Wyss et al. Citation2013). Farmed wetlands, however, generally have different biotic and abiotic characteristics than natural wetlands (Huner et al. Citation2002). Farming requires specific management tactics that often differ from regional wetland hydrologic characteristics (Richardson & Taylor Citation2003; Wyss et al. Citation2013) and application of pesticides, and synthetic fertilizers can greatly alter aquatic biota and community dynamics (Cohen et al. Citation1994; Schoenly et al. Citation1995; O'Malley Citation1999; Mize et al. Citation2008; Stenert et al. Citation2009; Stenert et al. Citation2010). Biodiversity in these managed ecosystems is often lower than natural wetlands (Piatti et al. Citation2010; Chen et al. Citation2013; Wyss et al. Citation2013; Moreira & Maltchik Citation2014). Many current organic farming and integrated pest management strategies are promoting farming methods that require less chemical inputs, which may aid in the ability of farms to function as surrogate wetlands (Lawler Citation2001; Lou et al. Citation2013).
A large portion of the Gulf of Mexico Coastal Plain was historically composed of natural wetlands and is currently composed of farmed wetlands, specifically rice fields (Dahl Citation2000; Blanche et al. Citation2012). While not as structurally diverse as natural wetlands, rice fields can provide habitat for wetland biota (Richardson & Taylor Citation2003; Wilson et al. Citation2008; Stenert et al. Citation2010), the composition of which can be greatly influenced by farming practices (Elphick & Oring Citation1998; Stenert et al. Citation2009). Agronomic practices in rice vary in the USA, with a wide range of water management practices from continuous flooding to cycles of flooding and drying (Blanche et al. Citation2012). Rice cultivation in this region relies heavily on chemical control practices for both weed and insect pests, which can be detrimental to biodiversity (Cohen et al. Citation1994; Blackman et al. Citation2014). Farmers frequently till the soil before planting, which can have varying effects on insect community composition as well (Lawler Citation2001; Stenert et al. Citation2010; Foley Citation2015).
Detrital subsidies to rice fields as well as other aquatic systems have been found to initiate trophic cascades in which primary producer populations are increased, leading to increases in predator numbers and reductions in primary consumers from the larger predator population (Settle et al. Citation1996; Cross et al. Citation2006; Leroux & Loreau Citation2008). Generating this cascade, especially early in the growing season in rice fields, has been found to reduce the number of nuisance and invasive organisms in rice fields in Asia (Settle et al. Citation1996; Jiang & Cheng Citation2004). Biological control via detrital subsidies can reduce the need for pesticide applications that negatively affect wetland biota, making cultivated rice fields more suitable as a surrogate wetland. Hesler et al. (Citation1993) and the theses of Puissegur (Citation1976) and Foley (Citation2015) are the only three known surveys of arthropod diversity in rice in the USA (California (Hesler et al. Citation1993) and Louisiana (Puissegur Citation1976; Foley Citation2015)), and none of these North American studies investigated effects of detrital subsidies. Surveys of Louisiana rice fields indicate that arthropod predators are present (Puissegur Citation1976; Foley Citation2015); the use of detrital subsidies may increase their abundance and reduce populations of nuisance organisms (primary consumers of rice).
The objective of this study was twofold. The first objective was to catalog the arthropod community in simulated rice paddies using multiple sampling methods. The second objective was to investigate the effects of detrital subsidies on arthropod communities in the simulated rice paddies. In particular, we were interested in whether detrital subsidies could elicit a trophic cascade in these simulated rice paddies, reducing the number of nuisance organisms. To determine if this was taking place, populations of rice water weevil (RWW), Lissorhoptrus oryzophilus (Kuschel), the major nuisance consumer of rice in Louisiana (Stout et al. Citation2001), were monitored. We expected that populations of RWW would be negatively affected by detrital subsidies due to the trophic cascade.
Material and methods
Study area
This study was conducted during the 2013 and 2014 rice growing season at the H. Rouse Caffey Rice Research Station, Crowley, LA, USA (30o14′23.9″N, 92o20′44.0″W) () on silt-loam soils (fine, montmorillonitic, thermic Typic Albaqualf). The research area is located in a subtropical region and has been used for rice cultivation and experimentation since 1908. Specific information on land use and cover prior to 1908 is lacking; however, most of this region was converted from tall grass prairie to agriculture between 1870 and 1900 (Allen et al. Citation2001), presumably including this site. Once converted to a research station the fields were on a rice-cattle rotation for some time, followed by a rice soybean rotation and then switched to a rice-fallow rotation. The experimental plots in which studies were conducted are in a two-year rice-fallow rotation for over 20 years. Earthen levees surrounded the plots with access to a lateral channel for irrigation.
2013 Field survey and detrital subsidy study
The experimental area was divided in 2013 into 16 leveed areas (plots) measuring 24.2 m × 4.9 m. Each plot had separate access to a lateral pipe for irrigation. Due to space constraints, plots were separated only by a 1 m wide earth levee that prevented water transfer between plots. Detrital subsidy or control status was randomly assigned, yielding eight control and eight detrital subsidy plots.
Detrital subsidies were applied to treatment plots in the form of Hopi-Gro Cow Manure and Compost Mix (Hopi-Gro, Hope Agri Products Inc., Hope, AR, USA). Detritus was hand cast into treatment plots on 26 March and 16 April at a rate of 18.5 kg per plot (1554 kg/ha) per date. All plots were flooded on 28 March with well water and floods were maintained for the duration of the experiment. On 25 April, all plots were seeded by hand casting with 1.4 kg of rice seed (cv. ‘Cheniere’) that had been pre-germinated following Blanche et al. (Citation2012). While not a typical agronomic practice in southern Louisiana, water seeding with continuous flooding used to be consistent with the experimental practices of other detrital subsidy studies (Settle et al. Citation1996; Jiang & Cheng Citation2004). The 29 d delay between flooding and planting was employed to allow aquatic predators to become established as RWW only oviposit under flooded conditions (Settle et al. Citation1996; Stout et al. Citation2002). No pesticides were used.
Sampling of plots for arthropods began on 4 April and was repeated every two weeks thereafter using four different methods: Gee crayfish traps, sweeps with D-nets, floating pitfall traps and root/soil corer sampling. A 42 cm × 23 cm 1.27 cm mesh cylinder Gee crayfish trap with 2.5 cm indented coned openings at each end was baited with a pellet of Purina Southern Pride crawfish bait (Purina Southern Pride, Shreveport, LA, USA) and placed in a random location within the plot 48 hrs before sampling. Aquatic netting was performed with a standard 30 cm, 500 micron mesh, D-net with one 2 m sweep in each plot. Invertebrates collected by these two methods were placed in 80% ethanol and taken back to the lab where they were sorted under a dissecting scope. Identification was done to the lowest taxonomic level practical using relevant keys and voucher specimens in the Louisiana State University Arthropod Museum (Arnett & Thomas Citation2000; Arnett et al. Citation2000; Triplehorn & Johnson Citation2005; Merritt et al. Citation2008).
Floating pitfall traps (see Parys & Johnson Citation2011 for trap design) were deployed at the time of flooding every 6 m along the midline of the plot and tethered to a 0.6 m landscape stake that was hammered into the sediment yielding three traps per plot. Traps were left out for the duration of the experiment and only removed for servicing. Each pitfall trap contained 2.5 cm of ethylene glycol (Prestone® green anti-freeze). Floating pitfall traps were serviced by pouring their contents into plastic bags (Whirl-Pak® (Nasco©), refilling them with ethylene glycol and placing them back in the field. Bag contents were identified and stored using the same methods as Gee traps and D-netting.
Coring was done at a single random location within each plot with a 9.2 cm × 7.6 cm metal corer. Cored samples were washed through a 40 mesh (0.47 mm) screen sieve bucket, and the remaining material was collected, taken back to the lab and stored at −20 C until sorted. Sorting sediment samples was done in a 5 cm deep pan filled with 2 cm of water and arthropods were identified using the same methods as described previously. These four invertebrate sampling methods resulted in six samples per plot for a total of 96 samples per sampling date. Labeled voucher specimens from all samples were deposited in the Louisiana State Arthropod Museum at Louisiana State University.
Once rice plants had reached the third leaf stage and emerged from the flood water, monitoring of RWW populations began by taking an additional root/sediment core during sampling (see Stout et al. Citation2001 for RWW larvae sampling and counting methods). One of these cores from each plot was randomly chosen and recollected for arthropod sampling using the previously mentioned methods.
2014 Field survey and detrital subsidy study
The 2014 field experiment was conducted in a manner similar to the previous year with some modifications. The experiment was performed at a different location within the same station that had been fallowed the previous growing season. Plot size was increased to 62.5 m × 5.8 m. The eight plots were divided into two blocks, with four plots on the north side of a central lateral and four plots on the south side with a minimum of 24.4 m between plots. Plots in each block were assigned to detrital subsidy or control treatments, with two plots of each treatment in each block.
Methods for applying detritus were similar to those used in 2013, but the number of applications was increased from two to four starting on 22 April, then on 16 and 30 May, and 12 June. Amount of detritus applied per date was increased from 18.5 to 72.6 kg per plot (2074 kg/ha) reflecting the increase in plot size, roughly 3× larger, as well as to increase ratio of detritus to plot area. All plots were flooded permanently on 22 April. On 23 May, plots were seeded using the same methods as the previous year, except 3.47 kg per plot was planted to keep rice density similar to 2013. A single application of a commercial herbicide and surfactant mixture was applied to each plot on 17 June due to heavy weed infestation, as the objective of this study was to quantify the biota of rice fields, not grass wetlands. The herbicide and surfactant mixture consisted of 0.0167 ml 3-(1-methylethyl)-1H,1,3-benxothiadiazin-4(3H)-one 2, 2-dioxide (Basagran) and 0.0079 ml Alkyl phenol ethozylate (Voyager) per liter of water with a total rate of 6.3 l per plot. No other pesticides were applied.
Methods for sampling arthropods were similar to those used in 2013 with some exceptions, mostly to account for the larger plot size. The number of floating pitfall traps per plot was increased from three to four and traps were spaced 12.2 m apart instead of 6 m. A second Gee trap was added as well as an additional D-net sweep. Thus, a total of eight samples were taken from each of eight plots on each sampling date. The sediment corer was not used in 2014 as it was time consuming and yielded almost no arthropods (0.007% of individuals found in 2013). Moreover, those arthropods found in core samples in 2013 were not unique to that sampling method and were unlikely to normally inhabit the sediment (e.g. Corixidae).
Concerns were raised during the 2013 field season that detrital subsidies were altering water quality, potentially confounding the experiment. Chlorophyll a and dissolved oxygen measurements were added in 2014 as a measure of water quality (King & Brazner Citation1999; Cochard et al. Citation2014). Chlorophyll a concentration was measured by taking a 1 l water sample from each plot in the early afternoon of the sample day. Water filtration was performed by the Freshwater Ecology Lab, School of Renewable Natural Resources, by filtering through 0.45 micro-m filter (Standard Method 10200 H; APHA Citation2012) under vacuum, and water samples were analyzed by fluorometry (TD 700, Turner Designs, Inc., San Jose, CA, USA) for chlorophyll a content (minimum 0.02 micro-g/l; EPA [Environmental Protection Agency] method 445.0) by the Wetland Biogeochemistry Institute Analytical Services, Louisiana State University, Baton Rouge, LA, USA. Measurement of dissolved oxygen was taken using a HACH Dissolved Oxygen Test Kit Model OX-2P. RWW monitoring was the same in 2014 as in 2013 with the exception that three instead of two root/substrate cores were taken. Cores were used solely for RWW monitoring in 2014.
Data analysis
Principal component analysis, detrended correspondence analysis and non-metric multidimensional scaling (NMDS) were used to compare arthropod communities between detrital treatments. To select the ordination most appropriate for the data, axes length and goodness of fit (STRESS) from these analyses were assessed based on criteria described in ter Braak (Citation1995), Leps and Smilauer (Citation2003), and Hirst and Jackson (Citation2007). Multi-response permutation procedure (MRPP) was then performed to determine differences between treatment and controls plots.
A sample-based rarefaction curve was computed for each treatment using EstimateS v. 9.1.0 (Colwell Citation2013). EstimateS was performed with 100 randomizations of species orderings without replacement and 10 individuals as the cut-off for rare species. Differences among treatments in predator populations (determined by Merritt et al. (Citation2008) and Triplehorn and Johnson (Citation2005)) were analyzed by a general linear model (abundance was log transformed) with correlated errors to account for sequential sampling using R v 3.0.3 with the package GEE v 4.13-18 (R Core Team Citation2014; Carey et al. Citation2015). RWW populations, chlorophyll a and dissolved oxygen were compared among treatments with analysis of variance in R v 3.03 (R Core Team Citation2014).
Results
Rice field community composition
Over 14 total sampling dates in two growing seasons, 1057 samples (687 in 2013 and 370 in 2014) were taken, yielding 143 different species spanning 13 orders for a total of 49,251 individuals (). Of the 13 orders found in the 1057 samples, Collembola represented 57.76% (27,616) of the individuals collected, with Diptera being the next most abundant order at 24.13% (11,886). Of the Diptera collected, 87.35% (10,383) were Chironomidae. Araneae (5.83%, 2873) and Coleoptera (5.87%, 2889) were the next most abundant orders (). Floating pitfall traps caught the greatest number of individuals over both years, 44,297, while aquatic netting caught 3898 and Gee traps found 981.
Table 1. Arthropod taxa sampled from rice fields in 2013 and 2014 and their total abundance found in each year in both their manure and control plots. The differences in numbers of Collembola, Carabidae and Staphylinidae in 2013 and 2014 are attributable to differences in the resolution to which taxa were identified in 2013 and 2014.
2013 Detrital subsidy study
Samples were taken on eight dates from 4 April to 16 July in 2013. NMDS found no differences in arthropod communities among control and detrital subsidy plots (STRESS = 0.11) ((a)). MRPP agreed with this assessment, finding no significant differences in communities between treatments (F1,2 = 0.69, p = 0.83). Date and plot were significant predictors of community composition, irrespective of treatment, meaning that some taxa were more strongly associated with certain plots and dates ((a)).
Figure 2. Non-metric multidimensional scaling of sites in rice fields between detrital subsidy plots (▴) and no-treatment (•) plots at the LSU AgCenter Rice Research Station, Crowley, LA, USA in 2013 (a) and 2014 (b). Date and Plot (arrows) indicate that the date and the plot the sample was taken is a strong predictor of community composition.
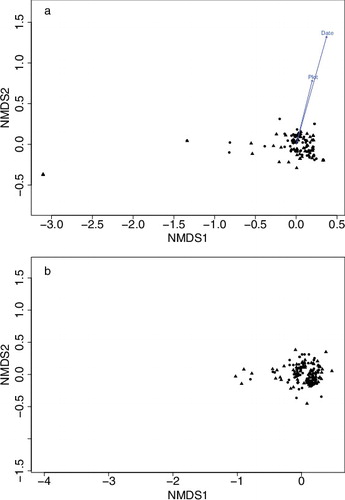
To generate sample-based rarefaction curves, the level of taxonomic resolution of adult insects and their immature stages must be consistent. For analysis of data in this study, higher-resolved taxa were downgraded to match less resolved life stages. For example, adult Tropisternus specimens were initially identified to species (T. lateralis and T. collaris), whereas immature Tropisternus sp. were not identified past genus. Consequently, for rarefaction curves, all Tropisternus counts were combined to the genus level. Comparison of control and treatment curves found no difference between treatments ((a)).
Figure 3. Sample based rarefaction curves for 2013 (a) and 2014 (b), comparing number of species expected to be found in a given number of samples in detrital subsidy and control plots. Note the difference in x- and y-axis scale.
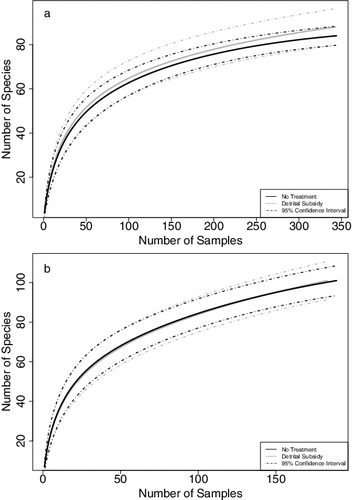
Predator populations, following the classification of Merritt et al. (Citation2008), did not differ between detrital subsidy and control plots (Z = −0.23, p = 0.22). RWW larvae counts were taken on the last four sampling dates, from 6 June to 16 July. RWW larvae numbers did not vary significantly among detrital subsidy and control plots on any sample date (6 June 2013 – Detritus 5.4 (±0.84 SE), Control 7.5(±1.1 SE); 20 June 2013 – Detritus 15.64 (±1.65 SE), Control 15.38 (±2.26 SE); 5 July 2013 – Detritus 12.38 (±1.08 SE), Control 9.82 (±0.98 SE); 18 July 2013 – Detritus 5.44 (±1.19 SE), Control 4.87 (±0.87 SE)).
2014 Detrital subsidy study
Samples were taken on six dates from 7 May to 16 July in 2014. NMDS and MRPP again found no differences among arthropod communities in control and detrital subsidy plots (STRESS = 0.14; MRPP, F1,2 = 0.83, p = 0.58) ((b)). Chlorophyll a concentrations did not differ between control and treatment plots (F1,2 = 0.97, p = 0.33). Similarly, dissolved oxygen levels were consistently 7 mg/l across plots of both treatments for all sampling dates. Monitoring chlorophyll a concentrations and dissolved oxygen ended on 18 June because compost-manure was no longer being added.
In 2014, the same taxonomic resolution adjustments were made as in 2013 for sample-based rarefaction curves. Comparison of treatment and control plots found no difference between rarefaction curves ((b)). Due to heavy weed pressure during the 2014 season, even after herbicide application, rice plants did not grow well and RWW larvae monitoring found only a few scattered larvae. Effects of detrital subsidies on abundance of RWW larvae were not analyzed for 2014. As in 2013, there was no effect of detrital subsidy on predators between treatments in 2014 (Z = −1.23, p = 0.66).
Discussion
The objectives of this study were to catalog arthropod diversity in farmed wetlands and to examine the influence of detrital subsidy on insect assemblages in rice fields. Samples from control plots indicate that insect families found in farmed wetlands are highly variable from year to year. Detrital subsidy did not substantially alter insect assemblages in either study year. These results suggest that rice fields are resilient to change from outside inputs, such as detritus.
RWW numbers, predator numbers, chlorophyll a concentration and dissolved oxygen levels were also unaffected by detrital subsidy. The failure to observe an effect of detrital subsidy was unlikely to be the result of insufficient sampling, as four different sampling methods were used and a large number of samples were taken (Meyer et al. Citation2011). The lack of response to detritus was unexpected, as subsidies to aquatic systems have resulted in alterations to the community in prior studies, including rice (Settle et al. Citation1996; King & Brazner Citation1999; Jiang & Cheng Citation2004; Cross et al. Citation2006; Ogren & King Citation2008). Moreover, the two most prevalent groups collected in this study, Collembola and Chironomidae, are largely composed of detritivores and were expected to respond to subsidies (Merritt et al. Citation2008). There are a few possible explanations for the failure of detrital subsides to elicit a trophic cascade. One possible explanation is that not enough detrital subsidy was applied to the field, as previous studies in rice have found a trophic cascade (Settle et al. Citation1996; Jiang & Cheng Citation2004). The total subsidy applied in 2014, however, exceeded quantities used in either of the previously mentioned studies which may indicate that another factor is limiting arthropod response.
A second possibility is that using another form of detritus, such as mulch, may elicit cascades more effectively than manure (Halaj & Wise Citation2002; Mathews et al. Citation2004; Schmidt et al. Citation2004). Another possibility is that experimental plots were too small for detritus to noticeably affect diversity, and scaling plots up to a whole field may be required to elicit trophic cascades (Garratt et al. Citation2011). This latter explanation does not seem plausible, as Settle et al. (Citation1996) used plots measuring 20 m × 20 m and found a significant effect. The duration of the experiment may not have been sufficient to observe an effect, and monitoring for a longer period of time may have resulted in a change in arthropod community as secondary consumers may have a delay in their response to detrital subsidies (O'Brien et al. Citation1992; Cross et al. Citation2007). In Asia, where studies have found effects of detrital subsidies, rice is cultivated year-round (>1 crop per year). Moreover, rice paddies are flooded for a greater portion of the season and plants are transplanted into flooded fields (Fernando Citation1993). Due to this, the rice ecosystem in Asia may represent a more permanent habitat. Rice in the USA is much more of a transient habitat, one crop per year, and the long period between seeding and permanent flooding does not allow a permanent community to establish.
Alternatively, detritus may not be limiting in this system; rather, other factors, such as inherent wetland insect resilience to change (e.g. Brock et al. Citation2003; Batzer Citation2013) and biotic interactions (Batzer & Rhui Citation2013), may be structuring diversity (Halaj & Wise Citation2002; Batzer & Palik Citation2007). The agricultural research station where this study occurred has been used for rice research since 1908. Continuous use over the years can possibly alter the local arthropod community. Anthropogenic alterations in land use can change a biotic community to one that is composed of species more tolerant of disturbances, such as detrital subsidies (Harding et al. Citation1998; Baumgartner & Robinson Citation2015). Harding et al. (Citation1998) looked at past and current watershed use, finding that habitat type (agriculture or forest) in the 1950s was a better predictor of current community structure than current land use, indicating that agriculture causes lasting alterations to the habitat. Baumgartner and Robinson (Citation2015) found that detrital subsidies were insufficient to alter macroinvertebrate stream communities that had a history of agriculture association, most likely because the current community is composed of less sensitive taxa. Evidence that this change may have taken place is provided by Foley (Citation2015), who found Cambaridae (Decapoda) as a common taxon in commercial rice fields. Cambaridae is susceptible to insecticides used in rice (Barbee & Stout Citation2009) and completely absent from the current study which suggests that the local habitat is no longer suitable. The complete absence of many other non-insect arthropods or non-arachnids, such as Amphipoda and Isopoda, was unexpected and also points to alterations in habitat. Moreover, qualitative comparisons to a recent survey of natural coastal wetlands suggest numerous other taxonomic differences, specifically with natural wetlands supporting four Odonata genera, one Hemiptera genera, seven Coleoptera genera, one Megaloptera genera and two Trichopera genera not found in this study (Kang Citation2011). Taken together, taxonomic differences between this study with Foley (Citation2015) and Kang (Citation2011) reinforce the premise that habitat may have been altered. Performing this study in commercial fields that have a shorter history of rice cultivation than the current site may give a better indication the suitability of rice fields as surrogate wetlands.
RWW larval populations did not differ between treatments in 2013 and monitoring was unsuccessful in 2014. This study provided no information of the potential for biological control of RWW by aquatic invertebrates, primarily because detrital subsidies failed to generate a trophic cascade. Another possible hindrance to biological control is the late appearance of natural enemies in plots, even with an extended pre-flood. Many of the large predators, which are most likely to prey on adult and larval RWW, were not found in plots until July. RWWs, in contrast, are most abundant and most important as pests early in the growing season, when rice is smaller and more vulnerable to RWW attack (Stout et al. Citation2012). RWWs are present during mid to late summer (when large predators are present), but they are not as important pests at that time because rice is larger and because they begin to migrate back to overwintering sites at that time (Tindall & Stout Citation2003). Our results conflict with the majority of findings that detrital subsidies in wetlands generate a trophic cascade (Settle et al. Citation1996; Jiang & Cheng Citation2004; Cross et al. Citation2006; Leroux & Loreau Citation2008; Ogren & King Citation2008; Hagen et al. Citation2012; but see Batzer & Palik Citation2007; Batzer Citation2013), although the possibility of generating a cascade with an increase in detritus cannot be ruled out. Trophic cascades are desirable as they can aid in biological control of nuisance and invasive organisms and reduce the need for chemical applications to fields. Reducing the amount of chemicals applied to a rice field would likely increase its ability to act as a surrogate wetland (Lawler Citation2001) and help to mitigate the loss of unmanaged wetland habitat.
This study constitutes the most comprehensive survey of arthropods in US rice paddies to date, as it used multiple sampling methods that sampled different rice paddy microhabitats (Meyer et al. Citation2011). This study demonstrated that intensively farmed wetlands may differ from less intensively farmed Gulf coast wetlands and are potentially not capable of supporting a trophic cascade response to detrital additions, unlike less intensively cultivated wetlands in other regions. Further arthropod surveys preferably in commercial rice plots and with increased duration of monitoring and detrital subsidies can elucidate possible ecosystem services.
Acknowledgments
The authors thank Marty J. Frey and student workers and staff at the H. Rouse Caffey Rice Research Station for assistance in maintaining rice plots and collecting samples. The authors also thank C.C. Carlton and the Louisiana State Arthropod Museum for assistance in identifying some of the insects.
Disclosure statement
The authors know of no conflict of interests or financial interests.
Additional information
Funding
Notes on contributors
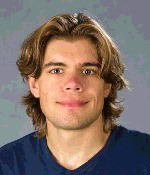
Nathan Mercer
Nathan Mercer is currently a graduate research assistant in graduate school working toward his Ph.D. in entomology. His focuses include ecology and integrated pest management.
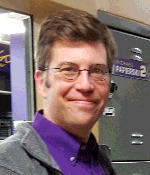
Michael D. Kaller
Dr Michael Kaller is an associate professor in the School of Renewable Natural Resources. His research interests in freshwater ecology and ecosystems are varied ranging from insects and invertebrates to feral hogs and alligators.
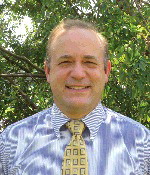
Michael J. Stout
Dr Michael Stout is professor and head in the Department of Entomology at Louisiana State University. His research interests include plant-insect interactions and management of insect pests in rice.
References
- Allen CM, Vidrine M, Borsari B, Allain L. 2001. Vascular flora of the Cajun Prairie of Southwestern Louisiana. In: Bernstein NP, Ostronder LJ,. Proceedings of the Seventeenth North American Prairie Conference, 2000 July 16–20, Mason City (IA): North Iowa Area Community College.
- [APHA] American Public Health Association. 2012. Standard methods for the examination of water and wastewater. 22nd ed. Washington (DC): American Public Health Association, American Water Works Association, Water Environment Federation; p. 1496.
- Arnett RHJ, Thomas MC. 2000. American beetles, volume I: Archostemata, Myxophaga, Adephaga, Polyphaga: Staphyliniformia. Boca Raton (FL): CRC Press LLC.
- Arnett RHJ, Thomas MC, Skelley PE, Frank JH. 2000. American beetles volume II: Polyphaga: Scarabaeiodea through Curculionoidae. Boca Raton (FL): CRC Press LLC.
- Barbee GC, Stout MJ. 2009. Comparative acute toxicity of neonicotinoid and pyrethroid insecticides to non-target crayfish (Procambarus clarkii) associated with rice-crayfish crop rotations. Pest Manage Sci. 65:1250–1256.
- Batzer DP. 2013. The seemingly intractable ecological responses of invertebrates in wetlands: a review. Wetlands. 33:1–15.
- Batzer DP, Palik BJ. 2007. Variable response by aquatic invertebrates to experimental additions of leaf litter input into seasonal woodland ponds. Fundam Appl Limnol. 168:155–167.
- Batzer DP, Rhui A. 2013. Is there a core set of organisms that structure macroinvertebrate assemblages in freshwater wetlands? Freshw Biol. 88:1647–1659.
- Baumgartner SD, Robinson CT. 2015. Land-use legacy and the differential response of stream macroinvertebrates to multiple stressors studied using in situ experimental mesocosms. Freshw Biol. 60:1622–1634.
- Blackman B, Autin T, Hummel N, Way MO, Stout MJ, Davis D. 2014. Management practices of Louisiana and Texas rice growers. Louisiana Agric Mag. [Internet]. [cited 2015 Jan 5]; 57:14–15. Available from: http://www.lsuagcenter.com/en/communications/publications/agmag/Archive/2014/Summer/Management-Practices-of-Louisiana-and-Texas-Rice-Growers.htm
- Blanche B, Harrell D, Saichuk J. 2012. General agronomic guidelines. In: Saichuk J, editor. Rice production handbook. Baton Rouge (LA): Louisiana State University; p. 3–15.
- Brock MA, Nielsen DL, Shiel RJ, Green JD, Langley JD. 2003. Drought and aquatic community resilience: the role of eggs and seeds in sediments of temporary wetlands. Freshw Biol. 48:1207–1218.
- Carey VJ, Lumley T, Ripley B. 2015. Generalized estimation equation solver [Internet]. R Foundation for Statistical Computing. Available from: http://cran.r-project.org/web/packages/gee/index.html
- Chen YH, Langellotto GA, Barrion AT, Cuong NL. 2013. Cultivation of domesticated rice alters arthropod biodiversity and community composition. Ann Entomol Soc Am. 106:100–110.
- Cochard R, Maneepitak S, Kumar P. 2014. Aquatic faunal abundance and diversity in relation to synthetic and natural pesticide applications in rice fields of central Thailand. Int J Biodivers Sci Ecosyst Serv Manage. 10:157–173.
- Cohen JE, Schoenly KL, Heong KL, Justo H, Arida G, Barrion AT, Litsinger JA. 1994. A food web approach to evaluating the effect of insecticide spraying on insect pest population dynamics in a Philippine irrigated rice ecosystem. J Appl Ecol. 31:747–763.
- Colwell RK. 2013. EstimateS: Statistical estimation of species richness and share species from samples. User's guide and application [Internet]. Available from: http://purl.oclc.org/estimates
- Cross WF, Wallace JB, Rosemond AD. 2007. Nutrient enrichment reduces constraints on material flows in a detritus-based food web. Ecology. 88:2563–2575.
- Cross WF, Wallace JB, Rosemond AD, Eggert SL. 2006. Whole-system nutrient enrichment increases secondary production in a detritus-based ecosystem. Ecology. 87:1556–1565.
- Dahl TE. 2000. Status and trends of wetlands in the conterminous United States 1986 to 1997. Onalaska (WI): U.S. Fish and Wildlife Services.
- Elphick CS, Oring LW. 1998. Winter management of Californian rice fields for waterbirds. J Appl Ecol. 35:95–108.
- Fernando CH. 1993. Rice field ecology and fish culture — an overview. Hydrobiologia. 259:91–113.
- Foley CC. 2015. Wading bird food availability in rice fields and crawfish ponds of the Chenier Plain region of southwest Louisiana and southeast Texas [thesis]. Baton Rouge (LA): Louisiana State University.
- Garratt MPD, Wright DJ, Leather SR. 2011. The effects of farming system and fertilizers on pests and natural enemies: a synthesis of current research. Agric Ecosyst Environ. 141:261–270.
- Hagen EM, McCluney KE, Wyant KA, Soykan CU, Keller AC, Luttermoser KC, Holmes EJ, Moore JC, Sabo JL. 2012. A meta-analysis of the effects of detritus on primary producers and consumers in marine, freshwater, and terrestrial ecosystems. Oikos. 121:1507–1515.
- Halaj J, Wise DH. 2002. Impact of detrital subsidy on trophic cascades in a terrestrial grazing food web. Ecology. 83:3141–3151.
- Harding JS, Benfield EF, Bolstad PV, Helfman GS, Jones EB. 1998. Stream biodiversity: the ghost of land use past. Proc Natl Acad Sci U.S.A. 95:14843–14847.
- Hesler LS, Grigarick AA, Oraze MJ, Palrang AT. 1993. Arthropod fauna of conventional and organic rice fields in California. J Econ Entomol. 86:149–158.
- Hirst CN, Jackson DA. 2007. Reconstructing community relationships: the impact of sampling error, ordination approach, and gradient length. Divers Distrib. 13:361–371.
- Hook DD. 1993. Wetlands: history, current status and future. Environ Toxicol Chem. 12:2157–2166.
- Huner JV, Jeske CW, Norling W. 2002. Managing agricultural wetlands for waterbirds in the coastal regions of Louisiana, USA. Waterbirds. 25:66–78.
- Jiang MX, Cheng JA. 2004. Effects of manure use on seasonal patterns of arthropods in rice with special reference to modified biological control of whitebacked planthopper, Sogatella furcifera Horvath (Homoptera: Delphacidae). J Pest Sci. 77:185–189.
- Kang SR. 2011. Aquatic macroinvertebrate and nekton community structue in a chenier marsh ecosystem: implications for whooping crane prey availability. [Dissertation]. Baton Rouge (LA): Louisiana State University.
- King RS, Brazner JC. 1999. Coastal wetland insect communities along a trophic gradient in Green Bay, Lake Michigan. Wetlands. 19:426–437.
- Lawler SP. 2001. Rice fields as temporary wetlands: a review. Isr J Zool. 47:513–528.
- Leps J, Smilauer P. 2003. Multivariate analysis of ecological data using CANOCO. Cambridge: Cambridge University Press.
- Leroux SJ, Loreau M. 2008. Subsidy hypothesis and strength of trophic cascades across ecosystems. Ecol Lett. 11:1147–1156.
- Lou YG, Zhang GR, Zhang WQ, Hu Y, Zhang J. 2013. Biological control of rice insect pests in China. Biol Control. 67:8–20.
- Manley SW, Kaminski RM, Reinecke KJ, Gerard PD. 2004. Waterbird foods in winter-managed ricefields in Mississippi. J Wildl Manage. 68:74–83.
- Mathews CR, Bottrell DG, Brown MW. 2004. Habitat manipulation of the apple orchard floor to increase ground-dwelling predators and predation of Cydia pomonella (L.) (Lepidoptera: Tortricidae). Biol Control. 30:265–273.
- Merritt RW, Cummins KW, Berg MB. 2008. An introduction to the aquatics insects of North America. 4th ed. Dubuque (IA): Kendall Hunt.
- Meyer CK, Peterson SD, Whiles MR. 2011. Quantitative assessment of yield, precision, and cost-effectiveness of three wetland invertebrate sampling techniques. Wetlands. 31:101–112.
- Mize SV, Porter SD, Demcheck DK. 2008. Influence of fipronil compounds and rice-cultivation land-use intensity on macroinvertebrate communities in streams of southwestern Louisiana, USA. Environ Pollut. 152:491–503.
- Moreira LFB, Maltchik L. 2014. Does organic agriculture benefit anuran diversity in rice fields? Wetlands. 34:725–733.
- O'Brien WJ, Hershey AE, Hobbie JE, Hullar MA, Kipphut GW, Miller MC, Moller B, Vestal JR. 1992. Control mechanisms of arctic lake ecosystems: a limnocorral experiment. Hydrobiologia. 240:143–188.
- O'Malley RE. 1999. Agricultural wetland management for conservation goals invertebrates in California ricelands. In: Batzer DP, Rader RB, Wissinger S, editors. Invertebrates in freshwater wetlands of North America: ecology and management. [place unknown]: Wiley; p. 857–885.
- Ogren SA, King DK. 2008. The effect of large woody debris on macroinvertebrate communities and epilithon detritus composition in a channelized headwater stream. J Freshw Ecol. 23:65–77.
- Parys KA, Johnson SJ. 2011. Collecting insects associated with wetland vegetation: an improved design for a floating pitfall trap. Coleopt Bull. 65:341–344.
- Piatti L, Souza FL, Filho PL. 2010. Anuran assemblage in a rice field agroecosystem in the Pantanal of central Brazil. J Nat Hist. 44:1215–1224.
- Puissegur WJ. 1976. Predators of the rice water weevil, Lissorhoptrus oryzophilus Kuschel and the effects of buferecarb, carbofuran and a dimilinpropanil mixture on these and other non-target aquatic species [thesis]. Baton Rouge, LA: Louisiana State University.
- R Core Team. 2014. R: a language and environment for statistical computing [Internet]. Available from: http://www.r-project.org
- Richardson AJ, Taylor IR. 2003. Are rice fields in southeastern Australia an adequate substitute for natural wetlands as foraging areas for egrets? Waterbirds. 26:353–363.
- Schmidt MH, Thewes U, Thies C, Tscharntke T. 2004. Aphid suppression by natural enemies in mulched cereals. Entomol Exp Appl. 113:87–93.
- Schoenly KG, Cohen JE, Heong KL, Arida GS, Barrion AT, Litsinger JA. 1995. Quantifying the impact of insecticides on food web structure of rice-arthropod populations in a Philippine farmer's irrigated field: a case study. In: Polis G, Winemiller K, editors. Food webs: integration of patterns and dynamics. New York (NY): Chapman & Hall; p. 343–351.
- Settle WH, Ariawan H, Astuti ET, Cahyana W, Hakim AL, Hindayana D, Lestari AS. 1996. Managing tropical rice pests through conservation of generalist natural enemies and alternative prey. Ecology. 7:1975–1988.
- Stenert C, Bacca RC, Ávila AC, Maltchik L, Rocha O. 2010. Do hydrologic regimes used in rice fields compromise the viability of resting stages of aquatic invertebrates? Wetlands. 30:989–996.
- Stenert C, Bacca RC, Maltchik L, Rocha O. 2009. Can hydrologic management practices of rice fields contribute to macroinvertebrate conservation in southern Brazil wetlands? Hydrobiologia. 635:339–350.
- Stout MJ, Hamm JC, Abbe I, Bergeron C. 2012. The influence of rice plant age on susceptibility to the rice water weevil, Lissorhoptrus oryzophilus. J Appl Entomol. 137:241–248.
- Stout MJ, Rice WC, Linscombe SD, Bollich PK. 2001. Identification of rice cultivars resistant to Lissorhoptrus oryzophilus (Coleoptera: Curculionidae), and their use in an integrated management program. J Econ Entomol. 94:963–970.
- Stout MJ, Riggio RM, Zou L, Roberts R. 2002. Flooding influences ovipositional feeding behavior of the rice water weevil (Coleoptera: Curculionidae). J Econ Entomol. 95:715–721.
- ter Braak CJF. 1995. Ordination. In: Jongman RHG, ter Braak CJF, van Tongeren OFR, editors. Data analysis in community landscape ecology. Cambridge: Cambridge University Press; p. 91–173.
- Tindall KV, Stout MJ. 2003. Use of common weeds of rice as hosts for the rice water weevil (Coleoptera: Curculionidae). Environ Entomol. 32:1227–1233.
- Triplehorn CA, Johnson NF. 2005. Study of insects. 7th ed. Belmont (CA): Brooks/Cole.
- Wilson AL, Watts RJ, Stevens MM. 2008. Effects of different management regimes on aquatic macroinvertebrate diversity in Australian rice fields. Ecol Res. 23:565–572.
- Wyss LA, Dugger BD, Herlihy AT, Gerth WJ, Li JL. 2013. Effects of grass seed agriculture on aquatic invertebrate communities inhabiting seasonal wetlands of the southern Willamette Valley, Oregon. Wetlands. 33:921–937.
- Zedler JB, Kercher S. 2005. Wetland resources: status, trends, ecosystem services, and restorability. Annu Rev Environ Resour. 30:39–74.