ABSTRACT
Identifying the spatial pattern of fish assemblages and the correlation between fish assemblages and environmental factors is basic for conserving and managing stream fishes. Based on data collected from 91 sampling segments within first-order through third-order streams of the Xin'an basin, China, we examined the spatial variation of fish assemblages in this area and related it to local, river-network and catchment factors. We found that fish species richness significantly increased from first-order to second- and third-order streams, but assemblage structures showed no among-stream variation. When the independent influence of the three categories of factors was considered, fish assemblages were significantly related to local habitat (e.g. wetted and substrate heterogeneity), tributary spatial position (e.g. confluence link and distance from mouth) and land use (e.g. agriculture and urbanization areas), respectively. However, when the effects of the these factors were considered jointly, local habitat and tributary spatial position were more important in influencing fish assemblages than land use. Our results suggest that fish assemblages in the headwater streams of the Xin'an basin were mainly determined by both local and spatial factors. The landscape data should be further refined in the future to provide more information for assessing how land use influence stream fishes.
Introduction
Distribution and abundance of stream fishes are influenced jointly by historical events (e.g. speciation, species differentiation and extinction), abiotic (e.g. climate, hydrology and physio-chemical factors) and biotic factors (e.g. competition and predation; Gilliam et al. Citation1993; Hoeinghaus et al. Citation2007; Dauwalter et al. Citation2008). At the local scale, due to the interspecific differentiations in physiological demand, ecological behavior and habitat preference of stream fishes (Jackson et al. Citation2001), local fish assemblages have been revealed to be affected by local habitat features, such as current velocity (Yan et al. Citation2011), water temperature (Wang, Lyons, Kanehl, et al. Citation2003), dissolved oxygen (Ostrand & Wilde Citation2001) and substrate size (Wang et al. Citation2013). Also, some descriptors on stream size, such as water depth (Harvey & Stewart Citation1991), stream width (Yan, Zhan, et al. Citation2010) and discharge (Chu, Wang, Yan, et al. Citation2015), may affect local fish diversity. At the stream-network scale, the nature of the continuities between stream segments may result in the spatial auto-correlation of abiotic and biotic factors and their ecological processes within a watershed network (Campbell-Grant et al. Citation2007). From this perspective, local fish assemblages are affected by, in addition to local habitat conditions, the tributary spatial position of each segment within the drainage network, which determines the rate of fish immigration and extinction (Taylor & Warren Citation2001; Grenouillet et al. Citation2004; Yan et al. Citation2011). Some descriptors on tributary spatial position, such as link magnitude, downstream link and confluence link, have been revealed to influence local species richness and assemblage structures of stream fishes (Osborne & Wiley Citation1992; Grenouillet et al. Citation2004; Smith & Kraft Citation2005; Yan et al. Citation2011; Li et al. Citation2014). In addition, at the catchment scale, the topography and land use/cover within a catchment may affect water-source supply and nutrient and sediment inflow (Omernik Citation2004; Wang et al. Citation2006), and influence the distributions and abundance of stream fishes thereafter (Allan Citation2004; Wang et al. Citation2006; Lange et al. Citation2014). Numerous researchers have documented the correlations between stream fish assemblages and forest cover (Jones et al. Citation1999; Sutherland et al. Citation2002), farmland use (Heitke et al. Citation2006; Chalar et al. Citation2013) and urbanization (Weaver & Garman Citation1994; Morgan & Cushman Citation2005).
The relative importance of different environmental factors in determining local fish assemblages may depend on the spatial scale at which a study is conducted (Jackson et al. Citation2001). Over a large spatial scale, one would expect precipitation, temperature, latitude, elevation and catchment size to be among the key factors influencing fish distribution; whereas, at a small spatial scale, current velocity, substrate size, canopy cover and stream gradient would be expected to be the key factors (Schlosser Citation1991; Jackson et al. Citation2001; Wang et al. Citation2006; Hoeinghaus et al. Citation2007). Also, the relative importance of different factors may depend on the features of environmental factors in a particular region (Wang et al. Citation2006). For a basin network with few anthropogenic disturbances, local species richness of fishes may be determined jointly by local habitat conditions and tributary spatial position (Grenouillet et al. Citation2004; Smith & Kraft Citation2005). However, in a basin network with numerous dams, the key factors influencing species richness are habitat variables, not tributary spatial position, because dams block fish passage and weaken the spatial auto-correlation in ecological factors and processes (Yan et al. Citation2011). In addition, studies on catchments with strong agriculture- or urban-forest gradients indicate that land uses are the key factors influencing stream fishes, and local-scale physical habitat plays a less important role (Wang et al. Citation2001, Citation2002; Wang, Lyons, Rasmussen, et al. Citation2003). In mixed forest-agriculture catchments, both catchment- and reach-scale factors may be important in influencing local fish assemblages (Richards et al. Citation1996; Allan et al. Citation1997; Burnett et al. Citation2006). According to Heitke et al. (Citation2006), in an agricultural catchment disturbed heavily, catchment-scale factors are less important than other environmental factors in influencing fish assemblages. However, this does not mean that agricultural land use shows no effect on fishes, but does mean that all study sites and rivers had similar levels of agricultural degradation.
There is no doubt that identifying the patterns of fish assemblage variation across different segments within a basin network and assessing how fish relate to local, stream-network and catchment factors are important for the conservation and management of stream fish. Regretfully, to our knowledge, reports that relate stream fish assemblages to different environmental factors from a riverscape perspective are quite rare in China, where stream fishes are being severely endangered due to many anthropogenic disturbances, such as dam construction, urbanization, agriculture production, industrial release and water pollution (Fu et al. Citation2003; Kang et al. Citation2009; Yan et al. Citation2013). In this study, based on the data collected in 91 sampling sites within first-order through third-order streams of the Xin'an basin, China, we examined the spatial variation in fish species richness and assemblage structures and assessed how fish assemblages related to local habitat, tributary spatial position and land use/cover within this basin. The aim of this study was to (1) examine the spatial variation in fish assemblages across first- to third-order streams, (2) determine how local habitat, tributary position and land use/cover influenced fish assemblages independently and jointly and (3) assess the relative importance of these factors in determining fish assemblages.
Methods
Study area
The Xin'an River originates the Wugujian Mountain in the Xiuning County, Anhui Province, and flows east toward its confluence with the Qiandao Lake, a reservoir located at the middle reach of the Qiantang River. This basin is more than 370 km long at the mainstem and about 11,000 km2 in area, of which 240 km of length and 6500 km2 of area upstream is located in the Anhui Province. The stream system of this basin is well developed and the tributaries longer than 10 km amount to more than 50. Because of the subtropical monsoon climate, the Xin'an basin is characterized by asymmetry in seasonal temperature and precipitation. Mean monthly air temperature ranges from 1–4 °C (January) to 27–29 °C (July). Annual rainfall is quite abundant (about 1800 mm/year), but about 80% of rainfall occurs during the period from April through September.
Fish sampling
A total of 56 first-order, 28 second-order and 7 third-order segments were surveyed once during July and August 2012 in this study (). Sample sites, each 50 m long and less than 1 m depth, were selected in the field based on habitat representativeness and accessibility. Each site involved at least two habitat-patch units (i.e. pool and riffle patches). Fish were collected using backpack electro-fishing gear (CWB-2000 P, China; 12 V import and 250 V export) by wading in two passes with comparable effort (approximately 30 min). Fish were identified to species (except for Ctenogobius fish due to the deficiency in identifying tools), counted and returned to the sampling sites if alive. Voucher specimens were stored in 8% formaldehyde solution for further identification in laboratory.
Local habitat survey
At each sampling site, we collected elevation (m), wetted width (m), water depth (m), current velocity (m/s), water temperature (°C), conductivity (mS/cm), dissolved oxygen (mg/L) and substrate coarseness and heterogeneity (). Elevation was determined by global positioning system. Wetted width was measured along three transects, equally spaced across the surveyed stream channel. Water depth, temperature, dissolved oxygen and conductivity were measured at three equal interval points along each transect (JENCO 6350, 9010, USA). Current velocity was taken at 60% of water depth at each point using FP111 (USA). Substrate was quantified with a 1-m lead corer divided into 10-cm sections, using the frequency size class method of Bain (Citation1999): particle size 1 = less than 0.06 mm; 2 = 0.06–1 mm; 3 = 2–5 mm; 4 = 15–63 mm; 5 = 64–256 mm; and 6 = greater than 256 mm. The mean and standard deviation of dominant substrate values represented substrate coarseness and heterogeneity, respectively.
Table 1. Summary statistic for predictor variables measured for local habitat, tributary spatial position, and catchment landscape condition.
Using ARC/INFO 9.3.1 software and the Digital Elevation Model Database with horizontal precision of 30 m, we established the vector diagram of the basin and determined the channel length of each sampling segment. Then, the channel slope of the sampling segment was calculated as the ratio between the vertical height and horizontal distance in the river channel as far as 200 m from the sampling sites.
Tributary spatial description
Following Yan et al. (Citation2011), three other basin-level metrics, in addition to stream order (Strahler Citation1957), were quantified to describe tributary spatial position for each surveyed segment, including stream link magnitude, confluence link and downstream link. Stream link magnitude is defined as the number of unbranched source tributaries upstream from a given segment in the drainage network (Shreve Citation1966). Confluence link is the number of confluences downstream from each stream segment (Fairchild et al. Citation1998). Downstream link is the link magnitude of the next downstream confluence (Osborne & Wiley Citation1992; ). Descriptions of these spatial variables were assigned to each segment derived from the Anhui Province Topographic Map (1:300 000 scale).
The other two spatial position variables () of each sampling point were measured on the above-mentioned vector diagram using ARC/INFO 9.3.1 software: (1) distance from headwater (m), i.e. the channel length from the sampling point to the headwater; (2) distance from mouth (m), i.e. the channel length from the sampling point to the mouth where Xin'an River flows to the Qiandao Lake ().
Catchment land use/cover measurement
Using ARC/INFO 9.3.1 software, Digital Elevation Model Database with horizontal precision of 30 m, and the above-mentioned vector diagram of rivers, we established the sub-catchments for each sampling segment. Without groundwater inflows, all sites in a single segment could have uniform hydrological, biological and physicochemical characteristics. Then, according to the Land Use Dataset of Anhui Province with scale of 1:100,000, we measured the relative area of five types of land use/cover for each sampling sub-catchment, including forest, meadow, agriculture, wetland and urbanization ().
Statistics
Each species collected in this study was examined for the frequency of occurrence (F) and relative abundance (RA) from Fi = 100(Si/S)% and RAi = 100(Ni/N)%, respectively, where Fi and RAi are F and RA of species i, Si and S are the numbers of samples in which species i were collected and of the total samples, and Ni and N are the individual numbers of species i and of total fish species, respectively.
One-way ANOVA was used to test the spatial variation in fish species richness across first-order to third-order streams. Tukey's test was used for post hoc comparison between different ordered streams after ANOVA if significance was observed. The data on species richness were log10(x + 1) transformed to meet the assumptions of ANOVA, including normality of distribution and homogeneity of variance using a Shapiro–Wilk normality test and Levene's test, respectively. Stepwise linear regression was used to identify the key explanatory variable(s) substantially influencing fish species richness. This procedure included separate stepwise analysis for local habitat, tributary spatial position and catchment land use to select the reduced subset of variables that maximally explained the variation in response variable. The predictor variables entering the analysis included pure habitat variables, pure spatial variables, pure land-use variables and the combination of the three types of variables. Prior to analysis, all variables were checked for normality using Shapiro–Wilk goodness-of-fit test; when necessary, they were log10(x + 1) transformed. The SPSS 13.0 statistics package was used to perform statistical analysis, and statistical significance was accepted at p < 0.05.
Discrete spatial variation in fish assemblage structures among first-order to third-order streams were identified using PRIMER 5.0 statistics package. Fish abundance data were log10(x + 1) transformed to moderate the influence of extreme data. Following a Bray–Curtis similarity matrix calculation, One-way ANOSIM, a method testing differences between groups of community samples (defined a priori) based on a similarity matrix, was performed to test the influence of stream order on fish assemblage structures. The relationships amongst assemblage structures from each sampling site were graphically represented using a non-metric multi-dimensional scaling analysis (NMS).
To evaluate how fish assemblage structures relate to explanatory variables, a redundancy analysis (RDA) was conducted using CANOCO 4.5 software package. We used RDA instead of a canonical correspondence analysis (CCA) in the relationship analysis because detrended correspondence analyses indicated that our fish data set had a short gradient length (a measure of species turnover) for which the linear model of RDA was more appropriate than CCA. Similar with that used in the above linear regression analysis, the predictor variables entering the RDA analysis also included pure habitat variables, pure spatial variables, pure land-use variables and their combinations. Both dependent and independent variables were log10(x + 1) transformed to minimize the influence of extreme data values. Species that occurred at less than two sites were excluded from the above analysis to avoid negligible weighting. Forward selection procedures were used to select independent variables before RDA analyses. The significance (p < 0.05) of the RDA was assessed by Monte Carlo permutation tests.
Results
Overview of fish diversity
A total of 7511 fish specimens were collected in this study, representing 33 species (including Ctenogobius spp. as one taxon), 11 families and 5 orders. Cyprinidae fish amounted to 18 species, comprising 54.5% of total species richness. Species richness per sample was 6.8 ± 3.8 (mean ± SD) species and abundance per site 82.5 ± 81.4 specimens. Zacco platypus, Acrossocheilus fasciatus, Ctenogobius spp., Vanmanenia stenosoma and Misgurnus anguillicaudatus were the most frequent (F > 40%) and abundant (RA > 10% except for Ctenogobius spp.). The frequency of occurrence for Odontobutis obscurus, Cobitis rarus, Sarcocheilichys parvus, Squalidus argentatus, Opsariichthys bidens, Pseudobagrus truncatus, Cobitis sinensis, Parabotia fasciata, Abbottina rivularis, Pseudorasbora parva, Carassius auratus and Rhodeus ocellatus were 10%–40%, among which seven species (e.g. O. obscurus, C. rarus, S. parvus) were relatively abundant (RA > 1%) while the others were relatively rare (RA < 1%). Parasinilabeo assimilis and Saurogobio dabryi were only collected in one site, and Phoxinus oxycephalu, Liobagrus styani, Channa argus and Oryzias latipes were collected in two sites ().
Table 2. Species composition, the frequency of occurrence (F) and relative abundance (RA) for fishes collected in the headwater streams of the Xin'an basin.
Local species richness
The data log10(x + 1)-transformed on fish species richness met to the assumptions of ANOVA (p > 0.05 both for Shapiro–Wilk's test and Levene's test). Results from One-way ANOVA showed that local species richness significantly varied among stream order (F = 14.2, p < 0.05). Species richness significantly increased from first-order (5.30 ± 2.70 species) to second-order (8.32 ± 3.91 species) and third-order streams (12.14 ± 3.24 species; p < 0.05), but the difference in species richness was not significant between second- and third-order streams (p > 0.05).
When the independent effect of the three categories of environmental variables was considered, fish species richness significantly related to four habitat variables, including wetted width, conductivity, slope and water temperature, two spatial variables (i.e. link magnitude and distance from mouth) and one land-use variable (i.e. urbanization area), respectively (p < 0.05; ). Only slope had negative correlation with species richness, and other variables positively related to species richness (). According to the r2 values, tributary spatial variables explained the most variability in species richness and land-use variables explained the least. When the combined effect of these explanatory variables on fish species richness was considered, one habitat (i.e. conductivity) and two spatial variables (i.e. distance from mouth and confluence link) had significant correlation with species richness (p < 0.05), while land-use factors were less important (p > 0.05; ).
Table 3. Effects of environmental factors on fish species richness based on stepwise linear regression.
Fish assemblage structure
According to the result from One-way ANOSIM testing how fish assemblage structures vary spatially, fish assemblages substantially overlapped and did not significantly vary among first-order through third-order streams (Global R = 0.06, p > 0.05). The NMS diagram showed that the assemblage structures in first-order streams were relatively scattered, and those in third-order streams were relatively concentrated. Overall, the assemblage structures presented as a subset from third-order through first-order streams in turn ().
Figure 2. Spatial variations of fish assemblage structures across stream orders based on non-metric multi-dimensional scaling.
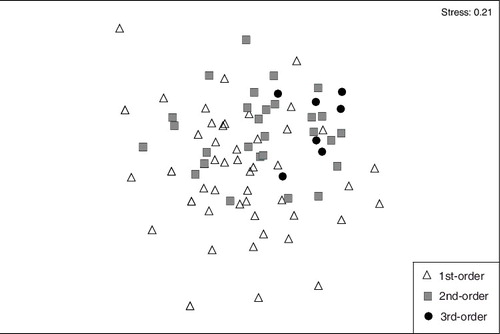
When analyzing the independent effect of the three types of environmental factors, results showed that six habitat, including elevation, dissolved oxygen, conductivity, wetted width, water depth and substrate heterogeneity, three spatial (i.e. stream order, confluence link and distance from mouth) and one land-use variables (i.e. agriculture area) had significant correlations on fish assemblage structures. These important variables explained 52.4% (local habitat), 31.4% (river-network) and 18.2% (land use) of variance of fish species, respectively. When analyzing the combined effects on assemblages by the three types of environmental factors, assemblage structures significantly related to five habitat (i.e. elevation, conductivity, wetted width, water depth and substrate heterogeneity) and two spatial variables (i.e. stream order and distance from mouth; p < 0.05), while no land-use variable showed significantly correlation with assemblage structure (p > 0.05; ).
Discussion
Influence of local habitat on stream fish assemblages
From headwaters to mouth, fluvial systems substantially vary in stream size and volume, habitat diversity and complexity and local habitat features, such as flow regime, current velocity, substrate and water temperature (Vannote et al. Citation1980; Matthews Citation1998). This may result in the upstream–downstream variation in species compositions and abundance of fishes and other aquatic organisms (Oberdorff et al. Citation1993; Chu, Wang, Yan, et al. Citation2015). Fish species richness often increases with stream size to reach a maximum at the mid-elevation reaches of streams (Minshall et al. Citation1985; Oberdorff et al. Citation1993; Sui et al. Citation2014). In the wadable headwater streams, at least, it may be a general pattern that fish species richness gradually increases downstream by species addition and/or replacement (Roberts & Hitt Citation2010). We found that local species richness of fishes increased from first-order through third-order streams, in spite of no significant difference occurring between second- and third-order streams. Also, we found that local species richness was significantly related to wetted width (when considering the correlation between fish assemblages and pure local habitat). Our results are consistent with this above upstream–downstream pattern because stream order may represent stream size and volume of each segment within a particular basin network (Strahler Citation1957). Associated with their different physiological demands and ecological behaviors, many stream fishes are specialists in habitat preference (Jackson et al. Citation2001). For example, some species those in the ‘riffle guild’ often inhabit shallow and fast-flowing water while other species those in the ‘pool guild’ are often observed in relatively deep and slow-flowing water (Schlosser Citation1982; Martin-Smith Citation1998; Zhu et al. Citation2015). At ‘riffle-pool’ sequence scale, habitat diversity and fish species richness may increase along the ‘riffle-pool’ gradient (Schlosser Citation1982), suggesting the negative correlation between slope and species richness because of the potential positive relationship of slope and water velocity in streams. This may explain our result that fish species richness negatively related to stream slope.
We also found that dissolved oxygen and substrate heterogeneity showed significant effect on fish assemblage structures in the headwater streams of the Xin'an basin. Dissolved oxygen positively related to the abundance of Z. platypus and Ctenogobius spp., and substrate diversity positively related to the abundance of V. stenosoma, A. fasciatus and V. barbatulus but is negatively to R. ocellatus, O. obscurus and A. rivularis. Many studies have revealed that, in the Qingyi (Yan, He, et al. Citation2010, Citation2011; Chu, Wang, Yan, et al. Citation2015) and Qiupu Rivers (Wang et al. Citation2013) at the Huangshan Mountain, Z. platypus, V. stenosoma, A. fasciatus, V. barbatulus and Ctenogobius spp. are the typical lotic species, often dwelling in small headwater streams. These streams are characterized by coarse substrate (e.g. bedrock, boulder and cobble), low water temperature, fast flowing and high dissolved oxygen. But A. rivularis, R. ocellatus and O. obscurus mainly inhabit the mid-low reach of these rivers, which is characterized by fine substrate, high water temperature, low flow and low dissolved oxygen.
Influence of river-network factors on stream fish assemblages
We found that, in addition to local habitat conditions, tributary spatial variables (i.e. confluence link and distance from mouth) are important in determining fish assemblages in this study area. Some researchers have also revealed that local fish assemblages are related to downstream link (Osborne & Wiley Citation1992; Grenouillet et al. Citation2004), confluence link (Smith & Kraft Citation2005; Yan et al. Citation2011; Li et al. Citation2014; Li et al. Citation2016) and distance from mouth (Schaefer & Kerfoot Citation2009). Streams may be viewed as an entire interconnected network architecture with a complex but definable ‘network geometry’, instead of just a relatively simple linear hierarchy (Benda et al. Citation2004; Campell-Grant et al. Citation2007). The nature of interconnections among segments indicates the potential auto-correlations of abiotic and biotic factors and their ecological processes among these segments (Grenouillet et al. Citation2004). Moreover, the rates of fish immigration and emigration, being important in determining local fish assemblages (Kadmon Citation1995; Taylor Citation1997), not only depend on the ecological behaviors (e.g. long-distance or short-distance migration) and swimming capacity of fishes, but on the spatial position of a particular segment within a basin network (Taylor & Warren Citation2001; Robinson & Rand Citation2005). For the small adventitious streams directly flowing towards large rivers, the fish fauna is often similar to large rivers and species richness is higher than small headwater streams (Gorman Citation1986).
Influence of catchment-scale factors on stream fish assemblages
The landscape features of a catchment, influencing water-source supply, nutrient inflows, flow regime, nutrient level and thermal condition in streams (Omernik Citation2004; Wang et al. Citation2006), may determine the distributions and abundance of stream fishes (Allan Citation2004; Wang et al. Citation2006; Lange et al. Citation2014). As well as natural landscape conditions (i.e. topography, geology, climate and forest cover, etc.), anthropogenic land uses in catchments have been widely reported to affect abiotic and biotic factors and ecological processes in streams (e.g. Harding et al. Citation1998; Allan Citation2004; Wenger et al. Citation2008). Urbanization and agriculture may alter local fish assemblages through modifying instream flow regime, channel morphology and water quality, of which a general pattern is fish diversity and index of biotic integrity negatively relating to land-use area (Booth & Jackson Citation1997; Wang et al. Citation2001; Heitke et al. Citation2006; Wenger et al. Citation2008). However, we found that, when the independent effects of land-use variables on fish assemblages were considered, urban area showed a positive correlation with fish species richness. On one hand, the fact that most urban lands are located at the mid-low reach of the Xin'an basin may perhaps explain this positive ‘species richness-urban land area’ correlation, because fish species richness often increase from stream headwaters to mid-low reach of a basin network (Roberts & Hitt Citation2010; Yan et al. Citation2011; Sui et al. Citation2014). On the other hand, habitat homogenization resulting from anthropogenic disturbances may further induce fish fauna homogenization, which could manifest as endemic species decreasing and cosmopolitan species increasing (Scott & Helfman Citation2001; Rahel Citation2002; Chu, Wang, Zhu, et al. Citation2015). For example, water warming associated with land use may cause the replacement of cool-water species by warm-water species, which increases the total species richness because of the warm-water species gain outnumbering cool-water species loss (Wang, Lyons, Kanehl, et al. Citation2003). According to Chu, Wang, Zhu, et al. (Citation2015), anthropogenic disturbances (e.g. dam building and urbanization) may modify local habitat features in headwater streams of the Qingyi River creating favorable conditions for the native invaders. Fishes formerly inhabiting the slow-flowing water of the mid-lower reaches can establish populations in headwaters. If the invasive species outnumber the locally disappearing species, then the positive correlation between urban land use and fish species richness in this study area can be explained.
The relative importance of different factors in influencing fish assemblages
We found that, when the joint effects of habitat, network and catchment factors on fish assemblages were considered, catchment-scale factors were less important than others, suggesting fish assemblages in this study area are mainly determined by local and spatial factors and their ecological processes. Our results are similar to that observed by Burnett et al. (Citation2006) and Heitke et al. (Citation2006). These authors revealed that, in the basins with spatial heterogeneity in natural environmental conditions and spatial homogeneity in anthropogenic disturbances, it is difficult to screen out the substantial correlation between fish data and human-activity data, because all study sites have similar levels of habitat degradation. Is this explanation suitable for the results observed in this study? According to the coefficients of variability showing the extent at which a specific environmental factor varied from place to place, we found that the overall coefficients of land-use/cover variables were not lower than that of habitat and spatial position variables (). This suggests that this study area does not present the spatially heterogeneous natural environment and homogenous land use as mentioned by Burnett et al. (Citation2006) and Heitke et al. (Citation2006). Hence, the conclusions of Burnett et al. (Citation2006) and Heitke et al. (Citation2006) cannot explain our results, that catchment-scale factors are less important in influencing fish assemblages than local- and network-scale factors. It should be mentioned that the influences of agriculture and urbanization on lotic systems are often ‘uncertain’. For an example of urbanization, its effecting magnitude and the degree to which lotic systems are impacted may depend on the intensity of urbanization development and the spatial position of a town/city within a catchment (e.g. the distance from lotic system; Booth & Jackson Citation1997). Also, the correlation between urbanization and lotic systems may change among different descriptors to urbanization (Wang et al. Citation2001). Wang et al. (Citation2001) compared a variety of measures of urbanization, such as connected imperviousness, commercial land, urban land and highway, street and parking, to identify which one(s) had the strongest relation with fish habitat quality, fish assemblage structure and biotic integrity in 47 small south-eastern Wisconsin, USA. These authors found that the amount of connected impervious surface in the watershed is the best descriptor of urbanization for predicting fish density, species richness and index of biotic integrity score. Moreover, in the same study watershed, Wang et al. (Citation1997) did not observe the significant correlation between the whole land area of urbanization and stream fish assemblages and habitat quality. This perhaps suggests that our result, catchment-scale factors are not important in influencing local fish assemblages in the Xin'an basin, may possibly result from the relatively coarse land-use/cover variables used in this study. Hence, in future research, it is very necessary to refine and optimize the catchment-scale environmental data for assessing the influence of land use/cover on fish assemblages in the headwater streams of the Xin'an basin.
Acknowledgments
We thank Xian Li and Chun Si for their help with field collections and lab work. We thank members of the Animal Ethic Committee of Anhui Normal University for technical advice and assistance.
Disclosure statement
No potential conflict of interest was reported by the authors.
Additional information
Funding
Notes on contributors
Ren Zhu
Ren Zhu is an assistant research scientist at Anhui Normal University (China) with interest in community ecology of freshwater fishes.
Qing Li
Qing Li was formerly a undergraduate student at Anhui Normal University (China) but now is an MSc candidate at the University of Chinese Academy of Sciences with interest in stem cell biology and embryonic development.
Wenjian Wang
Wenjian Wang is an MSc candidate at Anhui Normal University (China) with interest in biodiversity of stream fishes.
Ling Chu
Ling Chu is an associate professor at Anhui Normal University (China) with interest in biological invasion of aquatic systems.
Yunzhi Yan
Yunzhi Yan is a professor at Anhui Normal University (China) with interest in the utilization and conservation of freshwater fishery resources, community ecology of fishes in lotic systems, and invasion biology of freshwater fishes.
References
- Allan D, Erickson D, Fay J.1997. The influence of catchment land use on stream integrity across multiple spatial scales. Freshwater Biol. 37:149–161.
- Allan JD. 2004. Landscapes and riverscapes: the influence of land use on stream ecosystems. Annu Rev Ecol Evol Syst. 35:257–284.
- Bain MB. 1999. Substrate. In: Bain MB, Stevenson NJ, editors. Aquatic habitat assessment: common methods. Bethesda (MD): American Fisheries Society; p. 95–103.
- Benda L, Poff NL, Miller D, Dunne T, Reeves G, Pess G, Pollock M. 2004. The network dynamics hypothesis: how channel networks structure riverine habitats. BioScience. 54:413–427.
- Booth DB, Jackson CR. 1997. Urbanization of aquatic systems: degradation thresholds, stormwater detection and the limit of mitigation. J Am Water Resour Assoc. 33:1077–1090.
- Burnett KM, Reeves GH, Clarke SE, Christiansen KR. 2006. Comparing riparian and catchment influences on stream habitat in a forested, montane landscape. In: Hughes RM, Wang LZ, Seelbach PW, editors. Influences of landscapes on stream habitats and biological assemblages. Bethesda (MD): American Fisheries Society, Symposium 48; p. 179–197.
- Campbell-Grant EH, Lowe WH, Fagan WF. 2007. Living in the branches: population dynamics and ecological processes in dendritic networks. Ecol Lett. 10:165–175.
- Chalar G, Delbene L, González-Bergonzoni I, Arocena R. 2013. Fish assemblage changes along a trophic gradient induced by agricultural activities (Santa Lucía, Uruguay). Ecol Indic. 24:582–588.
- Chu L, Wang WJ, Yan LL, Yan YZ, Zhu R, Si C. 2015. Fish assemblages and longitudinal patterns in the headwater streams of the Chencun reservoir of the Huangshan area. Acta Ecol Sin. 35:900—910.
- Chu L, Wang WJ, Zhu R, Yan YZ, Chen YF, Wang LZ. 2015. Variation in fish assemblages across impoundments of low-head dams in headwater streams of the Qingyi River, China: effects of abiotic factors and native invaders. Environ Biol Fish. 98:101–112.
- Dauwalter DC, Splinter DK, Fisher WL, Marston RA. 2008. Biogeography, ecoregions, and geomorphology affect fish species composition in stream of eastern Oklahoma, USA. Environ Biol Fish. 82:237–249.
- Fairchild GW, Horwitz RJ, Nieman DA, Boyer MR, Knorr DF. 1998. Spatial variation and historical change in fish communities of the Schuylkill River drainage, southeast Pennsylvania. Am Midl Nat. 139:282–295.
- Fu C, Wu J, Chen J, Wu Q, Lei G. 2003. Freshwater fish biodiversity in the Yangtze River basin of China: patterns, threats and conservation. Biodivers Conserv. 12:1649–1685.
- Gilliam JF, Fraser DF, Alkins-Koo M. 1993. Structure of a tropical stream fish community: a role for biotic interactions. Ecology. 74:1856–1870.
- Gorman OT. 1986. Assemblage organization of stream fishes: the effect of rivers on adventitious streams. Am Nat. 128:611–616.
- Grenouillet G, Pont D, Hérissé C. 2004. Within-basin fish assemblage structure: the relative influence of habitat versus stream spatial position on local species richness. Can J Fish Aquat Sci. 61:93–102.
- Harding JS, Benfield EF, Bolstad PV, Helfman GS, Jones EBD. 1998. Stream biodiversity: the ghost of land use past. Proc Natl Acad Sci USA. 95:14843–14847.
- Harvey BC, Stewart AJ. 1991. Fish size and habitat depth relationships in headwater streams. Oecologia. 87:336–342.
- Heitke JD, Pierce CL, Gelwicks GT, Simmons GA, Siegwarth GL. 2006. Habitat, land use, and fish assemblage relationships in Iowa streams: preliminary assessment in an agricultural landscape. In: Wang L, Hughes RM, editors. Influence of landscape on stream habitat and biological communities. Bethesda (MD): American Fisheries Society Symposium 48; p. 287–303.
- Hoeinghaus DJ, Winemiller KO, Birnbaum JS. 2007. Local and regional determinants of stream fish assemblage structure: inferences based on taxonomic vs functional groups. J Biogeogr. 34:324–338.
- Jackson DA, Peres-Neto PR, Olden JD. 2001. What controls who is where in freshwater fish communities the roles of biotic, abiotic, and spatial factors. Can J Fish Aquat Sci. 58:157–170.
- Jones EB, Helfman GS, Harper JO, Bolstad PV. 1999. Effects of riparian forest removal on fish assemblages in southern Appalachian streams. Conserv Biol. 13:1454–1465.
- Kadmon R. 1995. Nested species subsets and geographic isolation: a case study. Ecology. 76:458–465.
- Kang B, He D, Perrett L, Wang H, Hu W, Deng W, Wu Y. 2009. Fish and fisheries in the Upper Mekong: current assessment of the fish community, threats and conservation. Rev Fish Biol Fish. 19:465–480.
- Lange K, Townsend CR, Gabrielsson R, Chanut P, Matthaei CD. 2014. Responses of stream fish populations to farming intensity and water abstraction in an agricultural catchment. Freshwater Biol. 59:286–299.
- Li X, Li YR, Chu L, Zhu R, Wang LZ, Yan YZ. 2016. Influences of local habitat, tributary position, and dam characteristics on fish assemblages within impoundments of low-head dams in the tributaries in the Qingyi River, China. Zool Res. 37:67–74.
- Li YH, Yan YZ, Zhu R, Zhou K, Chu L, Wan A, Wang X. 2014. Spatial variations in fish assemblages within the headwater streams of the Wanhe watershed: a river network-based approach. J Fish Sci China. 21:988–999.
- Martin-Smith KM. 1998. Relationships between fishes and habitat in rainforest streams in Sabah, Malaysia. J Fish Biol. 52:458–482.
- Matthews WJ. 1998. Patterns in freshwater fish ecology. Norwell (MA): Kluwer Academic Publishers; p. 318–379.
- Minshall GW, Cummins KW, Petersen RC, Cushing CE, Bruns DA, Sedell JR, Vannote RL. 1985. Developments in stream ecosystem theory. Can J Fish Aquat Sci. 42:1045–1055.
- Morgan RP, Cushman SF. 2005. Urbanization effects on stream fish assemblages in Maryland, USA. J N Am Benthol Soc. 24:643–655.
- Oberdorff T, Guilbert E, Lucchetta JC. 1993. Patterns of fish species richness in the Seine River basin, France. Hydrobiologia. 259:157–167.
- Omernik JM. 2004. Perspectives on the nature and definition of ecological regions. Environ Manage. 34:27–38.
- Osborne LL, Wiley MJ. 1992. Influence of tributary spatial position on the structure of warmwater fish communities. Can J Fish Aquat Sci. 49:671–681.
- Ostrand KG, Wilde GR. 2001. Temperature, dissolved oxygen, and salinity tolerance of five prairie stream fish and their role in explaining fish assemblage patterns. Tran Am Fish Soc. 130:742–749.
- Rahel FJ. 2002. Homogenization of freshwater faunas. Annu Rev Ecol Syst. 33:291–315.
- Richards C, Johnson LB, Host GE. 1996. Landscape-scale influences on stream habitats and biota. Can J Fish Aquat Sci. 53:295–311.
- Roberts JH, Hitt NP. 2010. Longitudinal structure in temperate stream fish communities: evaluating conceptual models with temporal data. In: Gido KB, Jackson DA, editors. Community ecology of stream fishes: concepts, approaches, and techniques. Bethesda (MD): American Fisheries Society Symposium 73; p. 281–299.
- Robinson JL, Rand PS. 2005. Discontinuity in fish assemblages across an elevation gradient in a southern Appalachian watershed, USA. Ecol Freshwater Fish. 14:14–23.
- Schaefer JF, Kerfoot JR. 2009. Fish assemblage dynamics in an adventitious stream: a landscape perspective. Am Midl Nat. 151:134–145.
- Schlosser IJ. 1982. Fish community structure and function along two habitat gradients in a headwater stream. Ecol Monogr. 52:395–414.
- Schlosser IJ. 1991. Stream fish ecology: a landscape perspective. BioScience. 41:704–712.
- Scott MC, Helfman GS. 2001. Native invasions, homogenization, and the mismeasure of integrity of fish assemblages. Fisheries. 26:6–15.
- Shreve RL. 1966. Statistical law of stream numbers. J Geol. 74:17–37.
- Smith TA, Kraft CE. 2005. Stream fish assemblages in relation to landscape position and local habitat variables. Tran Am Fish Soc. 134:430–440.
- Strahler AN. 1957. Quantitative analysis of watershed geomorphology. Tran Am Geophys Union. 38:913–920.
- Sui XY, Lu Z, Yan YZ, Chen YF, Jia YT. 2014. Influence of a large dam on the longitudinal patterns of fish assemblages in the Qingyi stream. Zool Res. 35:362–372.
- Sutherland AB, Meyer JL, Gardiner EP. 2002. Effects of land cover on sediment regime and fish assemblage structure in four southern Appalachian streams. Freshwater Biol. 47:1791–1805.
- Taylor CM. 1997. Fish species richness and incidence patterns in isolated and connected stream pools: effects of pool volume and spatial position. Oecologia. 110:560–566.
- Taylor CM, Warren ML. 2001. Dynamics in species composition of stream fish assemblage: environmental variability and nested subsets. Ecology. 82:2320–2330.
- Vannote RL, Minshall GW, Cumins KW, Sedell JR, Cushing CE. 1980. The river continuum concept. Can J Fish Aquat Sci. 37:130–137.
- Wang L, Lyons J, Kanehl P, Bannerman R. 2001. Impacts of urbanization on stream habitat and fish across multiple spatial scales. Environ Manage. 28:255–266.
- Wang L, Lyons J, Kanehl P. 2002. Effects of watershed best management practices on habitat and fish in Wisconsin streams. J Am Water Resour Assoc. 38:663–680.
- Wang L, Lyons J, Kanehl P, Rasmussen P, Seelbach P, Simon T. 2003. Impacts of urban land cover on trout streams in Wisconsin and Minnesota. Tran Am Fish Soc. 132:825–839.
- Wang L, Lyons J, Kanehl P, Gatti R. 1997. Influences of watershed land use on habitat quality and biotic integrity in Wisconsin streams. Fisheries. 22:6–12.
- Wang L, Lyons J, Rasmussen P, Seelbach P, Simon T, Wiley M, Kanehl P, Bake E, Niemela S, Stewart PM. 2003. Watershed, reach, and riparian influences on stream fish assemblages in the northern lakes and forest ecoregion, USA. Can J Fish Aquat Sci. 60:491–505.
- Wang L, Seelbach PW, Hughes RM. 2006. Introduction to landscape influences on stream habitats and biological assemblages. In: Hughes RM, Wang L, Seelbach PW, editors. Landscape influences on stream habitats and biological assemblages. Bethesda (MD): American Fisheries Society Symposium 48; p. 1–23.
- Wang WJ, Chu L, Si C, Zhu R, Chen WH, Chen FM, Yan YZ. 2013. Spatial and temporal patterns of stream fish assemblages in the Qiupu Headwaters National Wetland Park. Zool Res. 34:417–428.
- Weaver L, Garman G. 1994. Urbanization of a watershed and historical changes in a stream fish assemblage. Tran Am Fish Soc. 123:162–172.
- Wenger SJ, Peterson JT, Freeman MC, Freeman BJ, Homans DD. 2008. Stream fish occurrence in response to impervious cover, historic land use, and hydrogeomorphic factors. Can J Fish Aquat Sci. 65:1250–1264.
- Yan YZ, He S, Chu L, Xiang XY, Jia YT, Tao J, Chen YF. 2010. Spatial and temporal variation of fish assemblages in a subtropical small stream of the Huangshan Mountain. Curr Zool. 56:670–677.
- Yan YZ, Xiang XY, Chu L, Zhan YJ, Fu CZ. 2011. Influences of local habitat and stream spatial position on fish assemblages in a dammed watershed, the Qingyi stream, China. Ecol Freshwater Fish. 20:199–208.
- Yan YZ, Zhan YJ, Chu L, Chen YF, Wu CH. 2010. Effects of stream size and spatial position on steam-dwelling fish assemblages. Acta Hydrobiol Sin. 34:1022–1030.
- Yan YZ, Wang H, Zhu R, Chu L, Chen YF. 2013. Influences of low-head dams on the fish assemblages in the headwater streams of the Qingyi watershed, China. Environ Biol Fish. 96:495–506.
- Zhu R, Si C, Chu L, Rui M, Wu TT, Yan YZ. 2015. The spatio-temporal distribution of fish assemblages in the headwaters of the Qingyi River: a study based on the habitat patches. Acta Hydrobiol Sin. 39:686–694.