ABSTRACT
In order to investigate the effects of macrobenthos on the abundance and community composition of ammonia-oxidizing prokaryotes under different temperature conditions, laboratory microcosms containing two kinds of macrobenthos (Corbicula fluminea and Tubificid worms) were constructed. Real-time polymerase chain reaction (PCR) and clone libraries were applied to analyze the ammonia-oxidizing archaea (AOA) and bacteria (AOB) communities in the surface sediments. The lowest abundances of the archaeal and bacterial amoA gene were found in the samples cultured under 28 °C (archaeal amoA gene abundance, 2.71 × 106 copies/g dry sediment; bacterial amoA gene abundance, 1.17 × 107 copies/g dry sediment) of the C. fluminea group. However, there was no significant difference in terms of the abundance of archaeal amoA gene in all Tubificid worms treatment groups. Compared to the community composition of AOB, greater variations in the community composition of AOA were observed among the three different temperature groups.
Introduction
Nitrification plays a critical biogeochemical role in both individual ecosystems and the global nitrogen cycle (Beman & Francis Citation2006), in which the oxidation of ammonia is the rate-limiting and important step of the whole nitrogen cycling processes. For a long time, this process is considered to be performed by ammonia-oxidizing bacteria (AOB) (Kowalchuk & Stephen Citation2001). However, recent environmental genomic studies demonstrated that the ammonia-oxidizing archaea (AOA) have the similar function of AOB (Venter et al. Citation2004; Könneke et al. Citation2005; Francis et al. Citation2007). Previous studies have proved the important functions of AOA in various ecosystems including soils (Leininger et al. Citation2006), lake sediments (Zeng et al. Citation2012; Zhao et al. Citation2013), rhizosphere sediments (Zhao et al. Citation2014), marine water and sediments (Francis et al. Citation2005; Mincer et al. Citation2007) and hot springs (Zhang et al. Citation2008). Through feeding, growth and metabolism activity, the bioturbation of macrobenthos could affect the transportation of dissolved oxygen exchange at the sediment–water interface and these effects would also promote the release of nitrogen from the sediment (Aller Citation2001). Previous studies have investigated the effects of bioturbation of macrobenthos on the cycling of nutrient elements in the aquatic ecosystem (Mermillod-Blondin et al. Citation2004). Zhang et al (Citation2010) found that the bioturbation of Tubificid worms resulted in the destruction of the surface sediment, which influenced the properties and biochemical processes of lake sediments. The bacterial community composition in lake sediment would also be influenced by the bioturbation of macrobenthos (Zeng, Zhao, Liu et al. Citation2014). Moreover, bioturbation processes could increase the amount of electron acceptors for ammonia oxidation, and provide suitable microenvironments for the growth and metabolism of ammonia-oxidizing micro-organisms (Satoh et al. Citation2007). Additionally, previous studies have demonstrated that temperature could influence the community composition and activity of macrobenthos (Nie et al. Citation2011). Variations in the temperature could affect the activity of the macrobenthos (Nie et al. Citation2011), and then influence the sediment properties and the abundance and composition of the ammonia-oxidizing prokaryotes.
Corbicula fluminea and Tubificid worms are commonly found macrobenthos in eutrophic lake sediments (Cai et al. Citation2010). Previous studies have demonstrated that temperature would influence the activities of macrobenthos (Nie et al. Citation2011). However, it is still unknown whether the bioturbation of macrobenthos would influence the abundance and community composition of the ammonia-oxidizing micro-organisms under different temperatures.
In the present study, microcosms were constructed in the laboratory under different temperatures to investigate the response of ammonia-oxidizing micro-organisms under the bioturbation of two commonly found macrobenthos (Tubificid worms and C. fluminea). Real-time polymerase chain reaction (PCR) and clone libraries were applied to analyze the abundance and community composition of ammonia-oxidizing micro-organisms. The aim of this study is to seek the effects of temperature on the abundance and community composition of ammonia-oxidation micro-organisms under the bioturbation of macrobenthos.
Materials and methods
Sediment collection and construction of the microcosms
C. fluminea and Tubificid worms were collected by Peterson Grab in Pukou, which is located in the western part of Lake Taihu (N 31.295556°, E 119.937499°) and in severe eutrophic status (Lu et al. Citation2011; Cai et al. Citation2012). Sediment samples were collected using a core sampler (DM60, Mingyu, China). Both the lake water and sediments were sampled and rapidly transferred to the laboratory.
Microcosms were constructed in polyethylene glass columns to simulate the natural lake environment. The top 12 cm of the collected sediment cores were sectioned into 2 cm intervals, and the samples at the same depth were pooled together. The sediments were then sieved with mesh to remove the macrofauna and large particles. The prepared sediment samples were then fully homogenized and placed in polyethylene glass columns with 2 cm intervals corresponding to their original depths. Each of the columns was wrapped with aluminum foil in the sediments part and added 10 cm of lake water with an intravenous needle. The constructed microcosms were stabilized at room temperature for a week. The constructed microcosms were divided into C. fluminea and Tubificid worms groups. The biomasses added in each column were 500 ind./m2 for C. fluminea and 9000 ind./m2 for Tubificid worms (Cai et al. Citation2010). The microcosms were cultivated in incubators at three different temperatures, 15, 22 and 28 °C, respectively. Each temperature had three replicates for each kind of macrobenthos. The microcosms were cultivated under a 12:12 h light/dark cycles for 60 days. The surface sediment samples (0–1 cm) were collected using the self-made PVC pipes, which could accurately collected the sediment samples at a certain depth without disturbing the surrounding sediment and had been authorized a patent from the State Intellectual Property office of China (ZL 201220054471) (http://www.pss-system.gov.cn/).
Chemical analysis
A vacuum freeze dryer was employed to dry the sediment samples (ALPHA1-2, CHRIST, Germany). Sediment properties of samples of both before and after the incubation were investigated. Total nitrogen (TN) and total phosphorus (TP) in the sediment samples were digested using K2S2O8 and analyzed with spectrophotometer (Jin & Tu Citation1990). Ammonia nitrogen (NH4+-N) and nitrate (NO3−-N) were extracted by 2 M KCl and measured with a continuous flow analyzer (San++, SKALAR, Netherlands).
DNA extraction and real-time quantitative PCR
Extraction of the total DNA from sediment samples was carried out with the DNA extraction kit (Mobio PowerSoil DNA Isolation kit, USA). The concentration of the extracted DNA was determined using a BioPhotometer (Eppendorf, Hamburg, Germany).
Real-time quantitative PCR was performed to analyze the copy numbers of the archaeal and bacterial amoA gene in different sediment samples on the basis of SYBR Green I using an IQ5 Thermocycler (RG65HD, Corbett, Australia). The primers Arch-amoAF/Arch-amoAR (Francis et al. Citation2005) and amoA-1F/amoA-2R (Rotthauwe et al. Citation1997) were chosen for the amplification of the archaeal and bacterial amoA genes, respectively. Plasmid DNA was prepared and the concentrations of DNA were measured. Plasmid DNA was tenfold serial diluted for the construction of the standard curves (3.40 × 102 − 3.40 × 108 archaeal amoA gene copies per μl and 1.59 × 102 − 1.59 × 108 bacterial amoA gene copies per μl). The 20 μl PCR mixtures included 5 ng of the DNA template, 1 × SYBR Premix Ex Taq™ buffer (Takara, Japan), and 0.2 μM of each archaeal or bacterial amoA primer. The thermal cycling program for archaeal amoA PCR amplification was 3 min at 95 °C, 45 cycles of 30 s at 95 °C, 1 min at 53 °C, 20 s at 72 °C, and finally, 7 min at 72 °C. The thermal cycling program for bacterial amoA PCR amplification was: 3 min at 95 °C, 45 cycles of 30 s at 95 °C, 1 min at 50 °C, 20 s at 72 °C, and finally, 7 min at 72 °C. The specificity of the PCR products was examined by both the 2% agarose gel electrophoresis and melting curve analysis. Data analysis was carried out with the Rotor-Gene 6000 software package. The amplification efficiencies were 0.96--1.04 for the archaeal amoA gene and 0.95–1.04 for the bacterial amoA gene.
Construction of the clone libraries
Clone libraries were constructed for the three different temperature treatment groups. Archaeal and bacterial amoA gene fragments were amplified with the primers described earlier, whereas the cycle number of PCR amplification was reduced to 30 for both of the archaeal and bacterial amoA genes. Triplicate PCR products were pooled, gel purified (Axygen PCR cleanup purification kit) and cloned by the pGEM-T vector (Promega, Madison, WI, USA). The ligation products were transformed to Escherichia coli competent cells (DH5a, Takara, Japan). Picked transformants were grown overnight on Luria-Bertani (LB) agar plates containing 100 μg/ml ampicillin, 40 μg/ml X-Gal and 24 μg/ml Isopropyl β-D-Thiogalactoside (IPTG). The clones were checked by PCR amplification using vector primers (T7 and SP6) and sent to Shanghai Majorbio Biotechnology Co., Ltd. for DNA sequencing.
The vector sequences of the obtained results were removed by the DNAStar software package (Lasergene 14.1) (http://www.dnastar.com/). All of the sequences were compared with the sequences deposited in the GenBank database using BLAST. Operational taxonomic units (OTUs) were defined at 5% nucleotide differences. Multiple sequence alignments of the most related environmental sequences and clone sequences were performed with ClustalX (Thompson et al. Citation1997). Indices of diversity including the number of OTUs, Chao 1 (SChao1) and Shannon (H) indexes were calculated with the software program DOTUR (Schloss & Handelsman Citation2005). Neighbor-joining phylogenetic trees (based on Jukes–Cantor distances) were constructed based on alignments of the nucleic acid sequences using MEGA 4.0 (Tamura et al. Citation2007).
Nucleotide sequence accession numbers
The archaeal and bacterial amoA gene sequences obtained in this study have been deposited in the GenBank database under the accession numbers KJ688859-KJ689248.
Results
Sediment properties
Sediment properties of samples of both before and after the incubation were shown in . The concentrations of TN, TP, ammonia nitrogen and nitrate nitrogen differed among the treatment groups under different temperatures (). For the C. fluminea group, the sediment samples cultured under 15 °C maintained the highest concentrations of nitrate nitrogen (). For the Tubificid worms group, remarkable reduction in the concentrations of ammonia and nitrate nitrogen was found in 22 and 28 °C ().
Abundance of the archaeal and bacterial amoA gene
The abundances of both archaeal and bacterial amoA genes were analyzed by the real-time PCR analysis (). The abundances of the bacterial amoA gene were remarkably higher than those of the archaeal amoA gene in all groups. For the C. fluminea group, the abundances of both archaeal and bacterial amoA genes were initially increased and then decreased with the elevated temperatures and the lowest abundances of the archaeal and bacterial amoA genes were found in the samples cultured under 28 °C (archaeal amoA gene abundance, 2.71 × 106 copies/g dry sediment; bacterial amoA gene abundance, 1.17 × 107 copies/g dry sediment). However, there was no significant difference in terms of the abundance of archaeal amoA gene in all Tubificid worms treatment groups. The highest abundance of bacterial amoA gene was found in the sediment samples cultured under 22 °C (1.30 × 107 copies/g dry sediment) ().
Diversity and phylogenetic analysis of the archaeal and bacterial amoA gene
In order to understand the diversity of the archaeal and bacterial amoA gene in different sediment samples, clone libraries were constructed and the diversity indexes including Shannon (H) and SChao1 of the each clone library were calculated (). For the archaeal amoA gene, the highest diversity was found in the C. fluminea group cultured under 28 °C. Samples cultured under 22 °C maintained the lowest diversity of archaeal amoA gene for both kinds of macrobenthos (). For the bacterial amoA gene, the highest diversity was found in the samples cultured under 15 °C for both kinds of macrobenthos ().
Table 1. Diversity of the archaeal and bacterial amoA gene in sediment samples with the bioturbation of C. fluminea or Tubificid Worms under different temperatures.
Phylogenetic analysis was performed to investigate the community composition of both AOA and bacteria derived from different sediment samples (Figures S1 and S2). Compared to the community composition of AOB, greater variations in the community composition of AOA were observed among the three different temperatures. Nitrosopumilus was the dominant AOA group in all sediment samples except the samples cultured under 22 °C of the C. fluminea group (). Nitrososphaera became the most abundant AOA group in the samples cultured under 22 °C of the C. fluminea group. Nitrosotalea occupied small percentages of the AOA group in the samples cultured under 28 °C for both kinds of macrobenthos ().
Figure 3. Relative percentages of the different ammonia-oxidizing archaea and bacteria groups detected in surface sediment samples incubated under different temperatures.
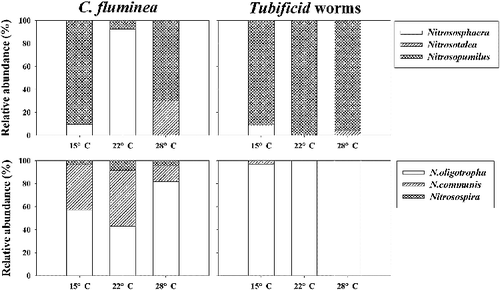
Nitrososmonas oligortopha was the dominant AOB group in all sediment samples (). Nitrososmonas communis covered 13.6%–48.6% of the AOB in the C. fluminea treatment groups; however, they just occupied a small proportion (2.9% under the 15 °C treatment group) of the AOB group in the Tubificid worms treatment groups. Nitrosospira was found in all of the C. fluminea treatment groups; however, it was absent in all of the Tubificid worms treatment groups ().
Relationships between environmental factors and abundance and diversity of archaeal and bacterial amoA gene
The bioturbation of macrobenthos changed the sediment properties of different treatment groups. In order to investigate the relationships between sediment properties and the abundance or diversity of the archaeal and bacterial amoA gene, the Spearman correlation coefficients were calculated and the results were shown in Table S1. The abundance of the archaeal amoA gene was positively correlated with the concentrations of TN (P < 0.05), whereas there was no significant correlation found between bacterial amoA gene abundance and TN (Table S1). Nitrate exhibited significantly positive relations with the number of detected OTUs of bacterial amoA gene (Table S1).
Discussion
In the present study, the bioturbation effects of C. fluminea and Tubificid worms on the abundance and community composition of AOA and bacteria under different temperatures were investigated. Remarkable differences in the abundance and community composition of ammonia-oxidizing micro-organisms in different treatment groups have been found.
Bioturbation results in the sediment reconstruction and biological flushing. For the Tubificid worms group, positive correlation was found between the temperature and the release amount of nitrogen from the surface sediment (). As the temperature increased from 15 to 28 °C, Tubificid worms would increase the frequency of fibrillation to get more dissolved oxygen, which would promote the release of nitrogen and phosphorus from the surface sediment (Nie et al. Citation2011). Huang et al. (Citation2016) constructed the microcosms to investigate the bioturbation effects of Tubificid worms in the lake ecosystem and found that the bioturbation of Tubificid worms promoted the release of nitrogen and phosphorus from the surface sediment to the overlying water. The highest abundance of the archaeal and bacterial amoA gene was found in the samples cultured under 22 °C of the C. fluminea group and no significant correlation was found for temperature and the abundance of the archaeal and bacterial amoA gene (). This was consistent with the results of Cao et al. (Citation2011), who investigated the abundances of archaeal and bacterial amoA genes in surface sediments from the Pearl River estuary to the South China Sea (from 2.9 to 21.3 °C). Wang et al. (Citation2014) found that the abundance of archaeal and bacterial amoA gene decreased with the increasing densities of C. fluminea in the microcosms cultured under 25 °C, suggesting the abundance of the amoA gene negatively correlated with the intensity of bioturbation. The results were not consistent with the present study if the intensity of bioturbation was positively correlated with the temperature. It could be inferred that temperature was the dominant factor controlling the abundance of ammonia-oxidizing micro-organisms from 15 to 22 °C; however, bioturbation turned to be the most important factor under 28 °C.
For the Tubificid worms group, the results obtained in the present study indicated that the abundance of the archaeal amoA gene was comparable among the three temperature groups. Similar result was also found in the microcosms cultured under the 28 °C conditions (Wu et al. Citation2013). Zeng, Zhao, Yu et al. (Citation2014) reported the positive correlation between the abundance of archaeal amoA gene and temperature in the microcosms. However, the elevated temperature would increase the intensity of bioturbation (Nie et al. Citation2011), which would decrease the abundance of the archaeal amoA gene (Huang et al. Citation2016). The comparable abundance of the archaeal amoA gene among different groups could be attributed to the combined effects of temperature and bioturbation.
In the present study, the lowest diversity of the archaeal amoA gene was found in the samples cultured under 22 °C for both the C. fluminea and Tubificid worms groups. Similar result has been found by Zeng, Zhao, Yu et al. (Citation2014) that the lowest diversity of the archaeal amoA gene was found in the medium temperature group (25 °C). Other study also reported the insignificant correlation between temperature and the diversity of the archaeal amoA gene (Cao et al. Citation2011).
Compared to the community composition of AOB, greater variations in the community composition of AOA were observed among the three different temperatures in the present study. A previous study conducted in the soil microcosm also found clearly changed community structure of the archaeal ammonia oxidizers other than bacterial ammonia oxidizers under different temperatures (10–30 °C) (Tourna et al. Citation2008). AOA and AOB maintained different responses to the variation of temperatures. Some species of AOA isolated from terrestrial hot springs (Nitrosocaldus yellowstonii) can grow at temperatures as high as 74 °C (de la Torre et al. Citation2008). However, AOB have not been found in environments consistently over 40 °C (Hatzenpichler Citation2012).
The bacterial amoA gene sequences obtained in the present study were mostly belonged to Nitrososmonas oligotropha lineage, which is often found in freshwater lakes; however, its ecophysiological traits remain unclear (Zeng, Zhao, Yu et al. Citation2014). Genus of Nitrosomonas has been considered to have a competitive advantage in the eutrophic environment (Koops et al. Citation2003), which is consistent with the higher concentrations of nitrogen and phosphorus in sediments of the present study. Moreover, only small percentages of Nitrosospira lineage were found in the C. fluminea treatment groups; however, this cluster was absent from all the Tubificid worms treatment groups, suggesting the different effects induced by different macrobenthos.
Conclusions
In conclusion, the present study focused on the effect of bioturbation on the community composition and abundance of AOA and AOB under different temperatures. Remarkable differences in the abundance and community composition of ammonia-oxidizing micro-organisms were observed among different temperatures and macrobenthos groups. The lowest abundances of the archaeal and bacterial amoA genes were found in the samples cultured under 28 °C of the C. fluminea group, whereas there was no significant difference of the abundance of archaeal amoA gene in all Tubificid worms treatment groups. Compared to the community composition of AOB, greater variations in that of AOA were observed among the three different temperatures. The data obtained in the present study would be helpful for further understanding the nitrogen cycling process in the freshwater ecosystem.
Revised_supplementary_information.docx
Download MS Word (42.7 KB)Disclosure statement
No potential conflict of interest was reported by the authors.
Additional information
Funding
Notes on contributors
Dayong Zhao
Dayong Zhao is an associate professor at Hohai University, China. His research focused on the nitrogen cycling in the lake ecosystem.
Xinyi Cao
Xinyi Cao is a PhD candidate at Hohai University, China.
Jin Zeng
Jin Zeng is an associate professor at Nanjing Institute of Geography and Limnology, Chinese Academy of Sciences. Her reseach focused on the microbial ecology in the lake ecosystem.
Rui Huang
Rui Huang is a PhD candidate at Hohai University, China.
Zhongbo Yu
Zhongbo Yu is a professor at Hohai University, China. His reseach focused on the hydrology and water resources.
References
- Aller RC. 2001. Transport and reactions in the bio irrigated zone. In:Boudreau BP, Jorgensen BB, editors. The benthic boundary layer: transport processes and biogeochemistry. New York (NY): Oxford University Press; p. 269–301.
- Beman JM, Francis CA. 2006. Diversity of ammonia-oxidizing archaea and bacteria in the sediments of a hypernutrified subtropical estuary: Bahia del Tobari, Mexico. Appl Environ Microbiol. 72:7767–7777.
- Cai YJ, Gong ZJ, Qin BQ. 2010. Community structure and diversity of macrozoobenthos in Lake Taihu, a large shallow eutrophic lake in China. Biodivers Sci. 18:50–59. Chinese.
- Cai L, Zhu G, Zhu M, Xu H, Qin B. 2012. Effects of temperature and nutrients on phytoplankton biomass during bloom seasons in Taihu Lake. Water Sci Eng. 5:361–374.
- Cao HL, Hong YG, Li M, Gu JD. 2011. Diversity and abundance of ammonia oxidizing prokaryotes in sediments from the coastal Pearl River estuary to the South China Sea. Anton Leeuw Int J G. 100:545–556.
- de la Torre JR, Walker CB, Ingalls AE, Ko¨nneke M, Stahl DA. 2008. Cultivation of a thermophilic ammonia oxidizing archaeon synthesizing crenarchaeol. Environ Microbiol. 10:810–818.
- Francis CA, Beman JM, Kuypers MMM. 2007. New processes and players in the nitrogen cycle: the microbial ecology of anaerobic and archaeal ammonia oxidation. ISME J. 1:19–27
- Francis CA, Roberts KJ, Beman JM, Santoro AE, Oakley BB. 2005. Ubiquity and diversity of ammonia-oxidizing archaea in water columns and sediments of the ocean. Proc Natl Acad Sci USA. 102:14683–14688.
- Hatzenpichler R. 2012. Diversity, physiology and niche differentiation of ammonia-oxidizing archaea. Appl Environ Microbiol. 78:7501–7510.
- Huang R, Zhao DY, Zeng J, Tian MY, Shen F, Jiang CL, Huang F, Yu ZB, Wu QL. 2016. Bioturbation of Tubificid worms affects the abundance and community composition of ammonia-oxidizing archaea and bacteria in surface lake sediments. Ann Microbiol. 66:1065–1073.
- Jin XC, Tu QY. 1990. Survey specification for lake eutrophication. Beijing: China Environmental Science Press.
- Könneke M, Bernhard AE, José R, Walker CB, Waterbury JB, Stahl DA. 2005. Isolation of an autotrophic ammonia-oxidizing marine archaeon. Nature. 437:543–546.
- Koops HP, Purkhold U, Pommerening-Röser A, Timmermann G, Wagner M. 2003. The prokaryotes: the lithoautotrophic ammonia-oxidizing bacteria. New York (NY): Springer; p. 778–811.
- Kowalchuk GA, Stephen JR. 2001. Ammonia-oxidizing bacteria: a model for molecular microbial ecology. Annu Rev Microbiol. 55:485–529.
- Leininger S, Urich T, Schloter M, Schwark L, Qi J, Nicol GW, Prosser JI, Schuster SC, Schleper C. 2006. Archaea predominate among ammonia-oxidizing prokaryotes in soils. Nature. 442:806–809.
- Lu G, Ma Q, Zhang J. 2011. Analysis of black water aggregation in Taihu Lake. Water Sci Eng. 4:374–385.
- Mermillod-Blondin F, Rosenberg R, François-Carcaillet F, Norling K, Mauclaire L. 2004. Influence of bioturbation by three benthic infaunal species on microbial communities and biogeochemical processes in marine sediment. Aquat Microb Ecol. 36:271–284.
- Mincer TJ, Church MJ, Taylor LT, Preston C, Karl DM, DeLong EF. 2007. Quantitative distribution of presumptive archaeal and bacterial nitrifiers in Monterey Bay and the North Pacific Subtropical Gyre. Environ Microbiol. 9:1162–1175.
- Nie XB, Wu SJ, Wu FT, Sun SQ, Jiang CB, Tan WC, Wan JL. 2011. Effects of the bioturbation activity of Tubifex tubifex on nitrogen release from sediments. Acta Scien Circum. 31:107–113. Chinese.
- Rotthauwe JH, Witzel KP, Liesack W. 1997. The ammonia monooxygenase structural gene amoA as a functional marker: molecular fine-scale analysis of natural ammonia-oxidizing populations. Appl Environ Microbiol. 63:4704–4712.
- Satoh H, Nakamura Y, Okabe S. 2007. Influences of infaunal burrows on the community structure and activity of ammonia-oxidizing bacteria in intertidal sediments. Appl Environ Microbiol. 73:1341–1348.
- Schloss PD, Handelsman J. 2005. Introducing DOTUR, a computer program for defining operational taxonomic units and estimating species richness. Appl Environ Microbiol. 71:1501–1506.
- Tamura K, Dudley J, Nei M, Kumar S. 2007. MEGA4: molecular evolutionary genetics analysis (MEGA) software version 4.0. Mol Biol Evol. 24:1596–1599.
- Thompson JD, Gibson TJ, Plewniak F, Jeanmougin F, Higgins DG. 1997. The CLUSTAL_X windows interface: flexible strategies for multiple sequence alignment aided by quality analysis tools. Nucleic Acids Res. 25:4876–4882.
- Tourna M, Freitag TE, Nicol GW, Prosser JI. 2008. Growth, activity and temperature responses of ammonia-oxidizing archaea and bacteria in soil microcosms. Environ Microbiol. 10:1357–1364.
- Venter JC, Remington K, Heidelberg JF, Halpern AL, Rusch D, Eisen JA, Wu D, Paulsen I, Nelson KE, Nelson W. 2004. Environmental genome shotgun sequencing of the Sargasso Sea. Science. 304:66–74.
- Wang X, Zhao DY, Zeng J, Yu DW, Wu QL. 2014. Effects of Corbicula fluminea bioturbation on the community composition and abundance of ammonia-oxidizing archaea and bacteria in surface sediments. Environ Sci. 35:2314–2321. Chinese.
- Wu Y, Ke X, Herna´ndez M, Wang B, Dumont MG, Jia Z, Conrad R. 2013. Autotrophic growth of bacterial and archaeal ammonia oxidizers in freshwater sediment microcosms incubated at different temperatures. Appl Environ Microbiol. 79:3076–3084.
- Zeng J, Zhao DY, Huang R, Wu QL. 2012. Abundance and community composition of ammonia-oxidizing archaea and bacteria in two different zones of Lake Taihu. Can J Microbiol. 58:1018–1026.
- Zeng J, Zhao DY, Liu P, Yu ZB, Huang R, Wu QL. 2014. Effects of benthic macrofauna bioturbation on the bacterial community composition in lake sediments. Can J Microbiol. 60:517–524.
- Zeng J, Zhao DY, Yu ZB, Huang R, Wu QL. 2014. Temperature responses of ammonia-oxidizing prokaryotes in freshwater sediment microcosms. PLoS One. 9:e100653.
- Zhang L, Gu X, Wang Z, Shen Q, Fan C, Zhong J. 2010. The influence of Tubificid worms bioturbation on the exchange of phosphorus across sediment-water interface in lakes. J Lake Sci. 22:666–674. Chinese.
- Zhang CL, Ye Q, Huang Z, Li W, Chen J, Song Z, Zhao W, Bagwell C, Inskeep WP, Ross C, et al. 2008. Global occurrence of archaeal amoA genes in terrestrial hot springs. Appl Environ Microbiol. 74:6417–6426.
- Zhao DY, Luo J, Zeng J, Wang M, Yan WM, Huang R, Wu QL. 2014. Effects of submerged macrophytes on the abundance and community composition of ammonia-oxidizing prokaryotes in a eutrophic lake. Environ Sci Pollut Res. 21:389–398.
- Zhao DY, Zeng J, Wan WH, Liang HD, Huang R, Wu QL. 2013. Vertical distribution of ammonia-oxidizing archaea and bacteria in sediments of a eutrophic lake. Curr Microbiol. 67:327–332.