ABSTRACT
Cyanobacterial blooms are serious eco-environmental problem, and cyanobacteria can produce large amounts of extracellular polymeric substances (EPS). EPS, as complex polymers, possess several characteristics, such as hydrophobicity, carbon-rich, nutrient-rich, metal adsorption, and stickiness, which influence the cell surface characteristics and the cycling of carbon, nutrients, metals, and rheological property in water, particularly during large blooms. All these characteristics are related to bloom outbreak. This review provides the classification, composition, extraction, and characteristics of cyanobacterial EPS and their corresponding roles in algal blooms. Further studies should investigate transparent exopolymer particles as a special and free form of EPS and their precise roles in cyanobacterial blooms in freshwater.
Introduction
Cyanobacterial blooms are abundant in eutrophic waters and widespread in summer throughout the world; these species are persistent in winter and appear year-round in eutrophic lakes (Ma et al. Citation2015). The health-affecting toxins and odorous compounds produced by cyanobacteria would restrict drinking water, irrigation, aquaculture, and fish breeding, and would seriously affect the human health (Saqrane and Oudra Citation2011). Thus far, the blooms have become a serious eco-environmental problem, and the mechanism of the bloom outbreak should be further investigated.
Cyanobacteria produce large amounts of extracellular polymeric substances (EPS). EPS have a defensive function and produce large quantities of EPS to reduce grazing by protists (Liu and Buskey Citation2000; Shnyukova and Zolotareva Citation2002; Sorrels et al. Citation2009). In addition, EPS have a metal-binding capacity (De Philippis et al. Citation2011) and have influence on carbon or nutrient cycling (Passow Citation2002; Pannard et al. Citation2016). They also participate in aggregate formation through adsorption and stickiness, which would influence the fate of blooms (Logan et al. Citation1995; Passow Citation2002). Thus, the in-depth study of Microcystis EPS attracts attention not only in terms of improving our comprehension of the roles of EPS in Microcystis blooms but also in improving the management of the harmful blooms by affecting the characteristics and production of EPS. Despite many efforts to elucidate the roles of algal EPS in aquatic systems, the roles of EPS in Microcystis blooms are still not well understood nor consistently reported. This situation may be attributed to the complex properties of EPS and the bloom outbreak mechanism. Meanwhile, studies on the algal EPS have generally been limited to single property. Therefore, the past research results should be summarized. This review aims to provide an overview on the classification, extraction, composition of Microcystis EPS and then review the characteristics and corresponding functions in cyanobacterial blooms emphatically. Finally, the main conclusions and the research needs are presented.
Classification, extraction, and composition of cyanobacterial EPS
EPS were generally fractionated into soluble EPS (sEPS) and bound EPS (bEPS), and bEPS were further divided into loosely bEPS and tightly bEPS (Sheng et al. Citation2010). These two types of bEPS were separated by a mild method for loosely bEPS (e.g. high speed centrifugation, heating at low temperatures) and a harsh method for tightly bEPS (e.g. heating at high temperatures, or chemical extraction methods) based on the harvested algae samples after sEPS collection (Sheng et al. Citation2010; Xu et al. Citation2013). However, transparent exopolymer particles (TEP), which were different from bEPS and sEPS, as a special type of EPS was proposed by Thornton (Citation2002) (). TEP exist as non-living and discrete particles rather than as dissolved substances, capsules, or surface coatings (Alldredge et al. Citation1993). The role of TEP in aquatic systems differs from other forms of EPS given that these individual particles in waters can aggregate and can be collected by filtration (Passow Citation2002). These transparent gel-particles are sticky particles and consist predominantly of acidic polysaccharides. In fact, sEPS and bEPS of cyanobacterial Microcystis also contained large amounts of acidic heteropolysaccharides (Chen et al. Citation2016). Similar components in EPS fractions indicate that a close connection was observed between the pools of sEPS, bEPS, and TEP in pelagic ecosystems as shown in (Thornton Citation2002), and the dynamic relationship would be strengthened during the algal blooming period.
The extraction method of bEPS could affect the composition and function of EPS and could further influence the relative contents of matters in EPS matrix (D'Abzac et al. Citation2010; Bourven Citation2011; Liu, Qin, Zhang et al. Citation2014). We attempted to compare several common extraction methods for cyanobacterial Microcystis and found that some extraction methods could cause loss of some materials in bEPS (Liu, Qin, Zhang et al. Citation2014). Xu et al. (Citation2013) and Qu et al. (Citation2012) used different extraction methods to characterize the bEPS and obtained conflicting result. The extraction media of algal bEPS included hot water, NaCl, NaOH, ethylene diamine tetraacetid acid, cation exchange resin, and sonication (De Brouwer et al. Citation2002; Takahashi et al. Citation2009; Liu et al. Citation2014). Intracellular polymers such as 2-keto-3-deoxyoctonate (Klock et al. Citation2007), phycobilin proteins (Liu, Qin, Zhang et al. Citation2014), DNA (Brown and Lester Citation1980), ATP, and transmission electron microscope analysis (Takahashi et al. Citation2009) were used to evaluate the rupture of cells. We initially concluded that NaOH solution at pH = 10 would be the suitable extract for Microcystis bEPS through comparing these common methods by evaluation of excitation–emission matrix (EEM) spectroscopy and measuring the release of intracellular compounds (Liu, Qin, Zhang et al. Citation2014). sEPS samples were generally collected directly by low-speed centrifugation or filtration, and the supernatant or the filtrate was considered as sEPS fraction. TEP as the special type and discrete particles of EPS were filtered, and the TEP samples on the filters were then stained for further measurement (Passow Citation2002).
These three forms of EPS could be directly exuded by cyanobacteria. Cyanobacterial EPS mainly contained carbohydrates (or polysaccharides) and proteins (Liu et al. Citation2014). Monosaccharides such as glucose, fucose, mannose, arabinose, and rhamnose were included in cyanobacterial polysaccharides (Li et al. Citation2001). To learn more about cyanobacterial exopolysaccharides and their characteristics, readers are referred to reviews such as Li et al. (Citation2001), De Philippis and Vincenzini (1998) and De Philippis et al. (2001).
TEP components were generally produced by both actively growing (exponential phase) and nutrient-limited (stationary/death phase) algae, and the colloidal fraction (<0.4 μm) is likely the dominant fraction among algal-derived TEP (Villacorte et al. Citation2015). According to our research, the TEP concentration per cell of Microcystis sp. was 15 pg Xeq./cell (xanthan gum equivalents per cell) (Liu, Qin, Huang Citation2014), which was similar to that of diatom Skeletonema sp. (0.02 ng Xeq./cell) but was smaller than those of other diatoms (Coscinodiscus granii, Eucampia zodiacus, Rhizosolenia setigera) (Fukao et al. Citation2010).
The composition of EPS, characterized by fluorescence EEM spectroscopy, showed that large amounts of protein-like and humic-like substances were distributed in EPS fractions (Xu et al. Citation2013; Liu, Qin, Zhang et al. Citation2014). Among them, the tryptophan-like substances were correlated with the Microcystis growth, and the tyrosine-like substances showed their relationship with cell amount under nitrogen limitation (Xu et al. Citation2013; Liu et al. Citation2017). Other materials such as lipids and uronic acids were also reported in EPS fraction (Shifrin and Chisholm Citation1980; Verspagen et al. Citation2006). Besides, given that allelopathy is a manifold ecological/physiological phenomenon and it plays a crucial role in aquatic ecosystems, the active and allelopathic materials exuded by phytoplankton and the allelopathic effect were also noted and investigated widely (Zheng et al. Citation2013; Żak and Kosakowska Citation2014).
Characteristics and ecological roles of cyanobacterial EPS in algal blooms
Colony and bloom formation mechanism in the blooms
Recently, two conceptual frameworks as shown in (Xu et al. Citation2014) and (Qin et al. Citation2016) were proposed to explain the colony and bloom formation, respectively.
Figure 2. Schematic illustration of the formation of cyanobacterial Microcystis bloom (dark balls indicate Microcystis cells, blue shapes indicate extracellular polymeric substances, and circles within a line indicate the negative zeta potential).
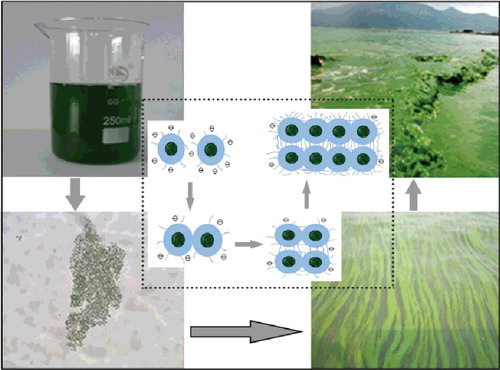
Figure 3. Conceptual explaining diagrams of cyanobacterial bloom ‘outbreak’ in Lake Taihu. (a) Cyanobacterial cell division and proliferation; (b) large colony formation through collision; (c) large colony floating upward to form visible bloom; and (d) cyanobacterial bloom transportation and migration along the onshore zone.
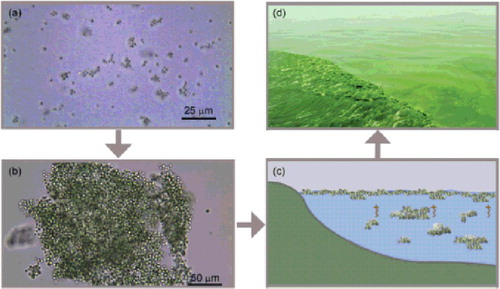
The conceptual frameworks of two theories were both proposed to elucidate Microcystis bloom formation mechanism in eutrophic Lake Taihu, China. Although one used extended Derjaguin–Landau–Verwey–Overbeek theory to explain the colony formation mechanism (Xu et al. Citation2014), another considered the abrupt appearance of Microcystis blooms within a short period and placed more emphasis on the collision and aggregation theory induced by hydrodynamic forces in shallow waters (Qin et al. Citation2016). However, both researchers focused on the important roles of EPS in colony and bloom formation. They both identified that EPS are involved in colony and mucilage formation. The colony formation was a survival strategy of Microcystis, which provides a competitive advantage over other phytoplankton species (Wu et al. Citation2007). Xu et al. (Citation2014) further pointed out that tightly bEPS were related to small colony formation, and loose bEPS might be closely linked with large colony or mucilage formation. Yang (Citation2010) gave a visual effect by using the scanning microscope to observe that there was more sticky and thick material enveloping the cell surface of colonial Microcystis than unicellular cells ().
Charge characteristics and hydrophobicity of cyanobacterial EPS
To some extent, the characteristics of cyanobacterial EPS determined the surface feature of cells, which directly affect the colony and bloom formation (Liu et al. Citation2016). The zeta potentials as the potential at the solid surface of a colloid electrical double layer are closely related to the charges on the particle surface. The surface charged characteristics of EPS were generally characterized with the parameters of zeta potential. According to our research based on zeta potential measurement, the surface charge of cyanobacterial Microcystis from culture and field were both negatively charged (Liu et al. Citation2016), and the isoelectric value of Microcystis cells calculated by the authors was similar to those of EPS from Microcystis (Henderson et al. Citation2008). Uronic acids might mainly contribute to the anionic character of cyanobacterial EPS (Verspagen et al. Citation2006; Klock et al. Citation2007).
The hydrophobic interaction could play a key role in the EPS assembly from phytoplankton (Ding et al. Citation2009), and the hydrophobicity of colony was stronger than that of single cell (Yang et al. Citation2011; Liu et al. Citation2016). Yang et al. (Citation2011) further specified that the extracellular polysaccharides may play a role in adhering cells together to form colonies. Zhang et al. (Citation2007) observed that the colonial Microcystis aeruginosa cells have thicker polysaccharides envelope than the unicellular cells. Monosaccharides such as fucose and rhamnose were considered to confer the hydrophobic property (Klock et al. Citation2007). However, the composition of monosaccharides of extracellular polysaccharides between the colonial and unicellular M. aeruginosa cells was not significantly different (Yang Citation2010).
Roles of carbon, nutrients, and other materials in EPS
EPS have the function of the accumulation of nutrients; therefore, EPS as the complex materials could be characterized by carbon, nitrogen, and phosphorus (Comte et al. Citation2006). A significant portion of photosynthetic production in phytoplankton is released as EPS into waters (Fogg Citation1983), which contributed to the nutrients and carbon pool. Marine phytoplankton generally excretes 5% to 20% of the carbon they fix (Mague et al. Citation1980), but more may be released under stress (Fogg Citation1983). EPS released by phytoplankton are carbohydrate-rich and can thus increase the carbon-to-nutrient ratios of the organic materials. The organic carbon of EPS of cyanobacterium Synechococcus sp. was 0.19–0.27 pg/cell (Ikegaya et al. Citation2015), and the ratio of dissolved acidic polysaccharides (dAPS) to dissolved organic carbon (DOC) of cyanobacterium Microcystis sp. could reach up to 46% (Liu et al. 2015). Polysaccharides were considered as the largest fraction of organic carbon in seawater (Passow Citation2002; Hung et al. Citation2003). Ye et al. (Citation2012) reported the total dissolved polysaccharides in fresh lakes accounted for 26% of DOC during summer cyanobacterial Microcystis blooms in Lake Chaohu, China. The contribution of dAPS to DOC could reach up to 40% during the blooms in Lake Taihu, China (Liu, Qin, Zhu et al. Citation2014). These results suggested that sEPS are the large fraction of dissolved carbon in waters. Besides, the existence of non-living organic carbon (such as TEP) is two orders of magnitude larger than the biomass-carbon (Hara and Koike Citation2000). The C:N ratios of TEP were above the Redfield ratio in marine system (Engel Citation2001). TEP in Lake Taihu account for 25% of particulate organic carbon in waters and even reach up to 40.5% during the summer blooms (Liu et al. Citation2014). TEP were reported to be photo-degraded by UV radiation, and the products of TEP photo-degradation may be low molecular weight compounds and dissolved inorganic carbon (Ortega-Retuerta et al. Citation2009; Xavier Mari et al. Citation2017). Thus, EPS would continue to affect the biological carbon pump in aquatic waters and the regulation of atmospheric CO2, in turn, would be connected with global warming. The cyanobacterial bloom outbreak prefers hot climate (Paerl and Huisman Citation2008), and the bloom expansion and risk increase with global warming (Paerl et al. Citation2011). Therefore, EPS would play an important role in the biogeochemical cycling of carbon, even further regulate the climate change and influence the bloom outbreak.
Hassler et al. (Citation2014) indicated that EPS for other nutrients would enhance the phytoplankton growth due to EPS, which contain micronutrients to some extent. However, most studies were focused on the effect of nutrients (mainly N or P) on EPS production (Shnyukova and Zolotareva Citation2002; Wang et al. Citation2011); information about the various nutrient contents in EPS was limited. Proteins as nitrogen source with assistance from other organisms were generally considered as one important part of EPS (Sheng et al. Citation2010; Liu, Qin, Zhang et al. Citation2014). Nitrogen-rich compounds (e.g. Tryptophan-like and tyrosine-like substances) were abundant in Microcystis EPS (Liu et al. Citation2017). High nitrogen contents in cyanobacterial EPS were also reported (Klock et al. Citation2007). Besides, although TEP were mainly composed of polysaccharides, they could adsorb the organic nitrogen (Engel Citation2001); thus, the nitrogen fraction was also found in TEP. Pannard et al. (Citation2016) indicated that the presence of phytoplankton that produces large amount of EPS could control the elemental ratios (C:N, C:P) of the organic materials in pelagic ecosystems, with potential repercussions on the trophic network. EPS exuded in waters as the form of sEPS or TEP could participate in aggregation formation, which have been widely investigated in oceans (Passow and Alldredge Citation1995). Berman et al. (Citation2007) revealed that modulation of TEP formation could regulate the fate of marine cyanobacterial blooms (Berman-Frank et al. Citation2007). TEP in freshwaters might be used as efficient nutrient storage during the beginning of Microcystis blooms by investigating TEP abundance during spring in Lake Taihu, China (Huang et al. Citation2016). Abundant Microcystis detritus associated with TEP were also found in Lake Taihu, which would further affect the behavior and fate of cyanobacterial blooms or suspended particles (Huang et al. Citation2016).
Moreover, EPS as the storage of nutrients can be absorbed directly by cyanobacterial cells, and the labile materials in EPS would also be used by heterotrophic bacteria as carbon and energy sources (Sheng et al. Citation2010). However, the refractory materials in EPS could be photo-degraded through UV absorption (Ortega-Retuerta et al. Citation2009). Large amounts of fluorescent substances (humic-like, tryptophan-like, and tyrosine-like), which could absorb UV-radiation, were found in Microcystis bEPS and sEPS (Liu, Qin, Zhang et al. Citation2014). These materials not only can degrade into low-molecular weight materials, which could be further used by bacteria or even decompose into carbon, N, P, and other nutrients (Kieber et al. Citation1989), but also could form ‘sunscreen’ – scytonemin during the photo-degradation process (Sorrels et al. Citation2009), which would explain that the buoyant-blooming Microcystis could still prevail on the water surface during summer under the strong ultraviolet radiation. Thus, carbon and nutrients in EPS can be involved in element cycling, which would further influence the bloom outbreak.
Microcystis has allelopathic ability. Zheng et al. (Citation2013) indicated that extracellular secretion from Microcystis would inhibit submerged macrophytes and stronger allelopathic effects during active growth of cyanobacterial blooms than during breakdown. The exudate solution from Microcystis was prepared by filtration through a glass fiber filter, and these exudates were essentially sEPS as mentioned above. That is, algal allelopathic substances could be considered as one component of EPS. Żak and Kosakowska (Citation2014) found that dissolved extracellular secretion of Microcystis sp. could influence the growth of green algae. Wang et al. (Citation2017) demonstrated that exudates of M. aeruginosa from the exponential growth phase and the stationary phase significantly inhibited the growth of green algae and diatom. This kind of characteristic would be used as the power ‘weapon’ and would benefit the proliferation of blooming cyanobacteria.
Metal adsorption
Owing to the presence of a large number of negative charges on EPS, EPS that produce cyanobacteria have been considered as chelating agents for the removal of positively charged heavy metal ions from waters (Cavoukian and Stoianov Citation2011; De Philippis et al. Citation2011). sEPS are the most efficient fraction in the metal adsorption (Cavoukian and Stoianov Citation2011). A high diversity of ligands on EPS can displace the metals that are weakly bound to EPS and increase the metal buffering capacity of the aquatic environment, which supports higher metal inputs before toxic effects are detected in the biota (Tonietto et al. Citation2014). TEP as the discrete type of EPS might aggregate, move downward, or upward due to the low density of TEP (Passow Citation2002; Azetsu-Scott and Passow Citation2004; Xavier Mari et al. Citation2017). The metals would be involved in various forms of element cycling via association with TEP rather than be distributed by water movement alone.
In another aspect, the characteristic of metal adsorption can induce algal cells to compete in waters (Hassler et al. Citation2014). The metal adsorption of EPS could be affected by surface charges of EPS. More negative charges, larger capacity of binding cations, and hence the competition for trace elements such as iron ions were increased. M. aeruginosa could carry more negative charges than Microcystis flos-aquae (Liu et al. Citation2016) and this phenomenon might elucidate to some extent why M. aeruginosa exists in lakes with the largest scale and the longest time and was dominant in number and frequency of occurrence (Zhai et al. Citation2013).
Stickiness and rheological properties of EPS
TEP mainly composed of acidic polysaccharides have been reported to be highly sticky (Passow Citation2002), would adhere to different materials including the Microcystis cells or colonies. Therefore, large amounts of acidic polysaccharides in bEPS fraction would also make the surface of cells or colonies sticky. Sticky phytoplankton would enhance the efficiency in which two cell particles that collide stick together afterward (Jackson Citation1990) and would be more easier to change from small particle into larger sized colonies. The stickiness of Microcystis cells was reported in the range of 0–0.47 (Verspagen et al. Citation2006), and the stickiness values of single cells in culture were nearly zero in our study (Liu et al. Citation2016); this result was confirmed with the bath-cultured Microcystis cells that were consistently in the form of single cells (Wu et al. Citation2007). The stickiness of colonial Microcystis sp. must be further investigated.
EPS are generally flexible and elastic; thus, disentangling these polymers may require high energy to totally detach the EPS (Villacorte et al. Citation2015) and they can induce viscosity in waters and cause elasticity (Jenkinson et al. Citation2015). Viscosity controls most hydrodynamic processes at the micro-scale, within which most currently investigated planktonic processes occur (Jenkinson et al. Citation2015). In seawater, the biological modifications of viscosity are compatible with a habit modification that creates more favorable, turbulence-free, critical conditions for the development of plankton (Jenkinson and Sun Citation2010). Jenkinson et al. (Citation2015) indicated that rheological effects might modulate the sinking and rising of organic and other materials, associated with marine or lake snow, dynamics of encounter in swimming plankton, adhesion processes, allelopathy, and the dynamics of micro zones of nutrients, toxins, and dissolved allelopathic substances. Large amounts of EPS were produced during the blooming waters. These effects would be magnified, and the specific influence should be investigated.
Conclusions and prospects
From the results reported in this review, EPS played multiple roles in cyanobacterial blooms. Given that EPS contain carbon, nitrogen, metals, and other nutrients, their production and utilization can influence the flux and cycling of many elements, such as carbon, nutrients, metals in aquatic environments, all these were closely related to the growth and proliferation of cyanobacterial Microcystis sp. The compositions (e.g. acidic polysaccharides, uronic acids) and characteristics (e.g. zeta potentials, hydrophobicity, and stickiness) of EPS were the crucial factors in affecting the colony formation of Microcystis, which was a key session to form the blooms.
EPS as complex materials, many unknown components, and corresponding characteristics must be further identified. Considering that the importance of EPS and cyanobacterial blooms appear year-round in eutrophic waters, EPS may play crucial roles in maintaining bloom-forming cyanobacterial persistence throughout winter; the specific mechanism also deserves further study. TEP as a special form of EPS are an important agent in the transport of energy and nutrients through pelagic ecosystems and play a significant role in the biogeochemical cycling of elements. However, their roles in cyanobacterial blooms are also poorly identified. In addition, the role of EPS in modulating water viscosity is poorly established. Water viscosity increased by EPS would affect the motor behavior of organisms (e.g. phytoplankton, zooplankton, et al.) and change the collision chances and the formation of aggregates, the sinking and rising of organic or other materials, and the dynamic of micro zones of nutrients. All these factors could further affect the bloom outbreak.
Disclosure statement
No potential conflict of interest was reported by the authors.
Additional information
Funding
Notes on contributors
Lizhen Liu
Lizhen Liu is an assistant research scientist at Jiangxi Academy of Science (China) with interest in water eutrophication.
Qi Huang
Qi Huang is an assistant research scientist at Jiangxi Normal University (China) with interest in physical geography and lake ecology.
Boqiang Qin
Boqiang Qin is a researcher and professor at Nanjing Institute of Geography and Limnology, Chinese Academy of Sciences (China) with interest in lake ecology.
References
- Alldredge AL, Passow U, Logan BE. 1993. The abundance and significance of a class of large, transparent organic particles in the ocean. Deep Sea Res Part I. 40:1131–1140.
- Azetsu-Scott K, Passow U. 2004. Ascending marine particles: significance of transparent exopolymer particles (TEP) in the upper ocean. Limnol Oceanogr. 49:741–748.
- Berman-Frank I, Rosenberg G, Levitan O, Haramaty L, Mari X. 2007. Coupling between autocatalytic cell death and transparent exopolymeric particle production in the marine cyanobacterium Trichodesmium. Environ Microbiol. 9:1415–1422.
- Bourven I. 2011. Characterisation of the mineral fraction in extracellular polymeric substances (EPS) from activated sludges extracted by eight different methods. Bioresource Technology. 102:7124–7130.
- De Brouwer JFCD, Wolfstein K, Stal LJ. 2002. Physical characterization and diel dynamics of different fractions of extracellular polysaccharides in an axenic culture of a benthic diatom. Euro J Phycol. 37:37–44.
- Brown MJ, Lester JN. 1980. Comparison of bacterial extracellular polymer extraction methods. Appl Environ Microb. 40:179–185.
- Cavoukian A, Stoianov A. 2011. Using extracellular polymeric substances (EPS)-producing cyanobacteria for the bioremediation of heavy metals: do cations compete for the EPS functional groups and also accumulate inside the cell? Microbiology. 157:451–458.
- Chen M, Xie M, Wu W, Yu F, Li P. 2016. [Chemical characteristics of capsular polysaccharide and water-soluble released exopolysaccharide from Microcystis]. J Lake Sci. 28:609–615. Chinese.
- Comte S, Guibaud G, Baudu M. 2006. Biosorption properties of extracellular polymeric substances (EPS) resulting from activated sludge according to their type: soluble or bound. Process Biochem. 41:815–823.
- D'Abzac P, Bordas F, Joussein E, Hullebusch EV, Lens PNL, Guibaud G. 2010. Characterization of the mineral fraction associated to extracellular polymeric substances (EPS) in anaerobic granular sludges. Environ Sci Technol. 44:412–418.
- De Philippis R, Sili C, Paperi R, Vincenzini M. 2001. Exopolysaccharide-producing cyanobacteria and their possible exploitation: a review. J. Appl. Phycol. 13:293–299.
- De Philippis R, Vincenzini M. 1998. Exocellular polysaccharides from cyanobacteria and their possible applications. FEMS Microbiol. Rev. 22:151–175.
- Ding YX, Hung CC, Santschi PH, Verdugo P, Chin WC. 2009. Spontaneous assembly of exopolymers from Phytoplankton. Terr Atmos Ocean Sci. 20:741–747.
- Engel A. 2001. Carbon and nitrogen content of transparent exopolymer particles (TEP) in relation to their Alcian Blue adsorption. Marecolprogser. 219:1–10.
- Fogg, G E. 1983. The ecological significance of extracellular products of Phytoplankton photosynthesis. Bot Mar. 26:3–14.
- Fukao T, Kimoto K, Kotani Y. 2010. Production of transparent exopolymer particles by four diatom species. Fish Sci. 76:755–760.
- Hara S, Koike I. 2000. Dynamics of organic marine aggregates: nanometer-colloids to marine snow. In: Handa N, Tanoue E, Hama T, editors. Dynamics and characterization of marine organic matter. Dordrecht: Springer; p. 277–298.
- Hassler CS, Norman L, Nichols CAM, Clementson LA, Robinson C, Schoemann V, Watson RJ, Doblin MA. 2014. Iron associated with exopolymeric substances is highly bioavailable to oceanic phytoplankton. Mar Chem. 173:136–147.
- Henderson RK, Parsons SA, Jefferson B. 2008. Successful removal of Algae through the control of zeta potential. Sep Sci Technol. 43:1653–1666.
- Huang Q, Liu L, Qin B, Cai X, Zhu G, Zhang Y, Gong Z, Tang X. 2016. Abundance, characteristics, and size spectra of transparent exopolymer particles and Coomassie stainable particles during spring in a large shallow lake, Taihu, China. J Great Lakes Res. 42:455–463.
- Hung CC, Guo L, Santschi PH, Alvarado-Quiroz N, Haye JM. 2003. Distributions of carbohydrate species in the Gulf of Mexico. Mar Chem. 81:119–135.
- Ikegaya H, Suzuki S, Ichise S, Furuta S, Wakabayashi S, Ohigashi T, Bamba D, Namba H, Kihara H, Kishimoto N. 2015. Estimation of organic carbon content of the Cyanobacterium Synechococcus sp. by soft X-ray microscopy. Geomicrobiol J. 32:827–835.
- Jackson GA. 1990. A model of the formation of marine algal flocs by physical coagulation processes. Deep Sea Res Part A. 37:1197–1211.
- Jenkinson IR, Sun J. 2010. WITHDRAWN: rheological properties of natural waters with regard to plankton thin layers. a short review. J Mar Syst. 83:287–297.
- Jenkinson IR, Sun XX, Seuront L. 2015. HORIZONS: thalassorheology, organic matter and plankton: towards a more viscous approach in plankton ecology. J Plankton Res. 37:1100–1109.
- Kieber DJ, Mcdaniel J, Mopper K. 1989. Photochemical source of biological substrates in sea-water – implications for carbon cycling. Nature. 341:637–639.
- Klock JH, Wieland A, Seifert R, Michaelis W. 2007. Extracellular polymeric substances (EPS) from cyanobacterial mats: characterisation and isolation method optimisation. Mar Biol. 152:1077–1085.
- Li P, Liu Z, Ren X. 2001. Chemical characterisation of the released polysaccharide from the cyanobacterium Aphanothece halophytica GR02. J Appl Phycol. 13:71–77.
- Liu H, Buskey EJ. 2000. The exopolymer secretions (EPS) layer surrounding Aureoumbra lagunensis cells affects growth, grazing, and behavior of protozoa. Limnol Oceanogr. 45:1187–1191.
- Liu L, Huang Q, Qin B, Zhu G, Wu P, Wu Y. 2016. Characterizing cell surface of blooming Microcystis in Lake Taihu, China. Water Sci Technol. 73:2731–2738.
- Liu L, Huang Q, Zhang Y, Qin B, Zhu G. 2017. Excitation-emission matrix fluorescence and parallel factor analyses of the effects of N and P nutrients on the extracellular polymeric substances of Microcystis aeruginosa. Limnologica – Ecol Manage Inland Waters. 63:18–26.
- Liu L, Qin B, Huang Q. 2014. [Advances in transparent exopolymer particles (TEP) in freshwaters [J]]. Adv Earth Sci. 29(10):1149–1157. Chinese.
- Liu L, Qin B, Zhang Y, Zhu G, Gao G, Huang Q, Yao X. 2014. Extraction and characterization of bound extracellular polymeric substances from cultured pure cyanobacterium (Microcystis wesenbergii). Journal of Environmental Sciences. 26:1725–1732.
- Liu L, Qin B, Zhu G, Zhang Y, Gao G, Gong Z, Huang Q. 2015. Distribution of dissolved acidic polysaccharides (dAPS) during cyanobacteria blooms in northern Lake Taihu. Limnology. 16:21–29.
- Logan BE, Passow U, Alldredge AL, Grossartt HP, Simont M. 1995. Rapid formation and sedimentation of large aggregates is predictable from coagulation rates (half-lives) of transparent exopolymer particles (TEP). Deep Sea Res Part II. 42:203–214.
- Ma J, Qin B, Paerl HW, Brookes JD, Hall NS, Shi K, Zhou Y, Guo J, Li Z, Xu H. 2015. The persistence of cyanobacterial (Microcystis spp.) blooms throughout winter in Lake Taihu, China. Limnol Oceanogr.
- Mague TH, Friberg E, Hughes DJ, Morris I. 1980. Extracellular release of carbon by marine phytoplankton; a physiological approach. Limnol Oceanogr. 25:262–279.
- Ortega-Retuerta E, Passow U, Duarte CM, Reche I. 2009. Effects of ultraviolet B radiation on (not so) transparent exopolymer particles. Biogeosciences. 6:3071–3080.
- Paerl HW, Hall NS, Calandrino ES. 2011. Controlling harmful cyanobacterial blooms in a world experiencing anthropogenic and climatic-induced change. Sci Total Environ. 409:1739–1745.
- Paerl HW, Huisman J. 2008. Blooms like it hot. Science. 320:57–58.
- Pannard A, Pédrono J, Bormans M, Briand E, Claquin P, Lagadeuc Y. 2016. Production of exopolymers (EPS) by cyanobacteria: impact on the carbon-to-nutrient ratio of the particulate organic matter. Aquat Ecol. 50:29–44.
- Passow U. 2002. Transparent exopolymer particles (TEP) in aquatic environments. Prog Oceanogr. 55:287–333.
- Passow U, Alldredge AL. 1995. Aggregation of a diatom bloom in a mesocosm: the role of transparent exopolymer particles (TEP). Deep Sea Res Part II. 42:99–109.
- De Philippis RD, Colica G, Micheletti E. 2011. Exopolysaccharide-producing cyanobacteria in heavy metal removal from water: molecular basis and practical applicability of the biosorption process. Appl Microbiol Biotechnol. 92:697–708.
- Qin B, Yang G, Ma R, Deng J, Li W, Wu T, Liu L, Gao G, Zhu G, Zhang Y. 2016. [Dynamics of variability and mechanism of harmful cyanobacteria bloom in Lake Taihu, China [J]]. Sci China Press. 61:759–770. Chinese.
- Qu F, Liang H, He J, Ma J, Wang Z, Yu H, Li G. 2012. Characterization of dissolved extracellular organic matter (dEOM) and bound extracellular organic matter (bEOM) of Microcystis aeruginosa and their impacts on UF membrane fouling. Water Res. 46:2881–2890.
- Saqrane S, Oudra B. 2011. Cyanobacterial toxins: a short review on phytotoxic effect in an aquatic environment. Afr J Environ Sci Technol. 5:1146–1151.
- Sheng GP, Yu HQ, Li XY. 2010. Extracellular polymeric substances (EPS) of microbial aggregates in biological wastewater treatment systems: a review. Biotechnol Adv. 28:882–894.
- Shifrin NS, Chisholm SW. 1980. Phytoplankton lipids: environmental influences on production and possible commercial applications. In: Shelef, G., Soeder, C.J., editors. Algae biomass. Amsterdam: Elsevier/North Holland Biomedical Press; p. 625–645.
- Shnyukova EI, Zolotareva EK. 2002. Effect of different sources of nitrogen on production of exopolysaccharides from Nostoc linckia (Roth) Born. et Flah. (Cyanophyta). Inter J Algae. 4:86–98.
- Sorrels CM, Proteau PJ, Gerwick, H W. 2009. Organization, evolution, and expression analysis of the biosynthetic gene cluster for scytonemin, a cyanobacterial UV-absorbing pigment. Appl Environ Microbiol. 75:4861–4869.
- Takahashi E, Goux D, Orvain F. 2009. Optimising extraction of extracellular polymeric substances (EPS) from benthic diatoms: comparison of efficiency of six EPS extraction methods. Mar Freshwater Res. 60:1201–1210.
- Thornton D. 2002. Diatom aggregation in the sea: mechanisms and ecological implications. Euro J Phycol. 37:149–161.
- Tonietto AE, Lombardi AT, Vieira AAH, Parrish CC, Choueri RB. 2014. Cylindrospermopsis raciborskii (Cyanobacteria) exudates: chemical characterization and complexation capacity for Cu, Zn, Cd and Pb. Water Res. 49:381–390.
- Verspagen JMH, Visser PM, Huisman J. 2006. Aggregation with clay causes sedimentation of the buoyant cyanobacteria Microcystis spp. Aquat Microb Ecol. 44:165–174.
- Villacorte LO, Ekowati Y, Neu TR, Kleijn JM, Winters H, Amy G, Schippers JC, Kennedy MD. 2015. Characterisation of algal organic matter produced by bloom-forming marine and freshwater algae. Water Res. 73:216–230.
- Wang W, Liu Y, Geng L, Yang Z, Chen Y. 2011. The effect of nitrogen concentration and its interaction with light intensity on population dynamics and polysaccharide content of Microcystis aeruginosa. J Freshwater Ecol. 26:241–248.
- Wang LC, Zi JM, Xu RB, Hilt S, Hou XL, Chang XX. 2017. Allelopathic effects of Microcystis aeruginosa on green algae and a diatom: evidence from exudates addition and co-culturing. Harmful Algae. 61:56–62.
- Wu ZX, Gan NQ, Huang Q, Song LR. 2007. Response of microcystis to copper stress – do phenotypes of Microcystis make a difference in stress tolerance? Environ Pollut. 147:324–330.
- Xavier Mari UP, Christophe M, Adrian B. Burd LL. 2017. Transparent exopolymer particles effects on carbon cycling in the ocean. Prog Oceanogr. 151:13–37.
- Xu H, Cai H, Yu G, Jiang H. 2013. Insights into extracellular polymeric substances of cyanobacterium Microcystis aeruginosa using fractionation procedure and parallel factor analysis. Water Res. 47:2005–2014.
- Xu H, Jiang H, Yu G, Yang L. 2014. Towards understanding the role of extracellular polymeric substances in cyanobacterial Microcystis aggregation and mucilaginous bloom formation. Chemosphere. 117:815–822.
- Yang H, Cai Y, Xia M, Wang X, Shi L, Li P, Kong F. 2011. Role of cell hydrophobicity on colony formation in Microcystis (Cyanobacteria). Int Rev Hydrobiol. 96:141–148.
- Yang Z. 2010. Study on the driving factors of colony formation in Microcystis [dissertation]. Nanjing city (China): University of Chinese Academy of Sciences Publishers.
- Ye, LL, Shi, XL, Zhang, M, Xiao-Dong, WU, Kong, FX. 2012. [Distribution of carbohydrates species during summer bloom in Lake Chaohu]. China Environ Sci. 32:318–323. Chinese.
- Żak A, Kosakowska A. 2014. Allelopathic influence of cyanobacteria microcystis aeruginosa on green Algae Chlorella vulgaris. Geoplanet Earth Planet Sci. 14:141–150.
- Zhai C, Song S, Zou S, Liu C, Xue Y. 2013. The mechanism of competition between two bloom-forming Microcystis species. Freshw Biol. 58:1831–1839.
- Zhang M, Kong F, Tan X, Yang Z, Cao H, Xing P. 2007. Biochemical, morphological, and genetic variations in Microcystis aeruginosa due to colony disaggregation. World J Microbiol Biotechnol. 23:663–670.
- Zheng G, Xu R, Chang X, Hilt S, Wu C. 2013. Cyanobacteria can allelopathically inhibit submerged macrophytes: effects of Microcystis aeruginosa extracts and exudates on Potamogeton malaianus. Aquat Bot. 109:1–7.