ABSTRACT
Limnoperna fortunei (L. fortunei) is one of the most abundant freshwater bivalves in southeast Asia, with wide-ranging direct and indirect impacts on ecosystems. To estimate material flows in the habitats of L. fortunei, a combination of stable-isotope and fatty-acid analyses were applied to assess the feeding spectrum of L. fortunei in southern China. Using the isotope-mixing model, the contribution proportions to the diet of L. fortunei were estimated as 19.8%–28.2% for plankton, 57.6%–65.2% for particulate organic matter (POM) and 10.2%–21.1% for sediment organic matter. We conclude that POM is the principal food source of L. fortunei. The δ13C enrichment of fixed carbon from POM to L. fortunei was 0.67%–2.41%. Based on the fatty acid data, it was estimated that L. fortunei consumed or selectively accumulated Chlorophyceae, Cryptophyceae, Dinophyceae, bacteria and terrestrial organic matter. The feeding spectrum of L. fortunei is similar to that of Dreissena polymorpha. We suggest that L. fortunei is able to differentiate suitable food items using chemical cues and the surface properties of particles.
Introduction
The bivalve mussel, Limnoperna fortune (L.fortunei), is a benthic suspension feeder with a widespread distribution in fresh waters of Southeast Asia and South America (Paolucci et al. Citation2010; Zhang et al. Citation2014; Zhang et al. Citation2015). Adults of L.fortunei are able to firmly attach to hard substrata using the byssus (Nishino Citation2012). The density of L.fortunei is extremely high, reaching 10,000 individuals m−2 near the bank of the Xizhijiang River, southern China (Xu et al. Citation2009). With individual filtration rates up to 350 mL h−1, L.fortunei has a marked effect on suspended and sediment organic matter (SSOM), which modified the nutrient supply (Boltovskoy et al. Citation2009; Di Fiori et al. Citation2012). Therefore, L.fortunei provides an important link between SSOM and consumers in fresh water. Consequently, to understand the energy and material flows in the habitats of L.fortunei, it is necessary to clarify the composition of diet of this species.
Physical methods to determine the diet of bivalves include analysis of stomach contents and faecal pellets (Lehane and Davenport Citation2004). Stomach-contents analysis of L.fortunei living in the Middle Paraná River indicated that the main food ingested comprised plankton, particularly Euglenophyta, Rotifera, Chydoridae and Bosminidae (Molina et al. Citation2010). However, this method did not identify which dietary items were assimilated and can provide only rough estimates of recent feeding activity (seconds to hours) (Kang et al. Citation1999). More recently, biochemical methods, such as the analysis of stable isotopes or fatty acids, have been used with considerable success in determining the diet of bivalves (Kürten et al. Citation2013; Najdek et al. Citation2013; Braeckman et al. Citation2015). Stable-isotope analysis is a useful approach for identifying the food sources assimilated by bivalve mussels (Vaughn and Hakenkamp Citation2001). Consumers typically contain more heavy isotopes than is present in their diets, with stepwise heavy-isotope enrichment occurring at each trophic level (Peterson and Fry Citation1987). The ratio of 13C/12C (δ13C) is preferentially used as a dietary tracer because there is little fractionation (ca. 1.0%) between the consumer and its food sources (Asante et al. Citation2010; Sun et al. Citation2012). In contrast, the method of using fatty acids as dietary tracers in aquatic ecosystems relies on the observation that different primary producers (e.g. diatoms, dinoflagellates, bacteria) generally have specific fatty-acid profiles in their biomass. Furthermore, these fatty acid profiles tend to be transferred conservatively and thus are present in consumers’ tissues in an unchanged form (Shin et al. Citation2008). Therefore, it is possible to distinguish dietary and non-dietary compounds in the body of a consumer via fatty-acid analysis. These new approaches are therefore able to improve the accuracy of information on the diet of L.fortunei. Such information is essential for understanding energy and material flows in the habitats in which it occurs.
Bivalves are considered to be herbivores and it is assumed that plankton, particulate organic matter (POM) and sediment organic matter (SOM) are the main components of their diet (Molina et al. Citation2010; Zhao et al. Citation2013). In this work, we used a combination of stable-isotope and fatty-acid analyses to evaluate the contribution of plankton, POM and SOM to the diet of L.fortunei. Using these data, we assessed the feeding spectrum of L.fortunei in the Xijiang River, southern China.
Materials and methods
Sample collection and preparation
Triplicate samples of the plankton, POM and SOM were collected monthly between March and November 2013. The sampling site (23°08′12″ N, 112°48′7″ E) () is locate at the Xijiang River (south China), and its riverbed is rock based. The water quality of sampling site belong to Class I of the Surface water Environmental Quality Standard (Citation2002). Samples of plankton were collected with a net of 60 µm mesh by repeated multiple horizontal short duration tows (5–10 min) carried out between 2–8 m depth. About 30 L of fresh water were collected and stored in acid-cleaned polyethylene bottles to obtain samples of POM. SOM samples were collected at depths of 8–10 m via the sediment corer (300 mm in diameter, PC-300, Mooring Systems Inc., Cataumet, MA). Adult L.fortunei (≥22 mm) were collected from the riverbed at same site using SCUBA at depths of 2–10 m. All samples were immediately stored at 4 °C before being transported to the laboratory.
Figure 1. Sampling site (23°08′12″ N, 112°48′7″ E) locating at the Xijiang River is about 140 kilometres from seaport. The Xijiang River cover a full distance of over 2214 kilometres.
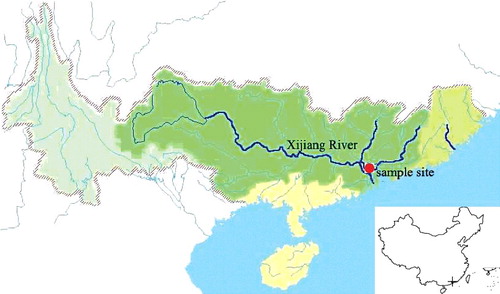
In the laboratory, samples of plankton were freeze-dried, ground into powder using a pestle and mortar, and stored in acid-cleaned polyethylene bags at −80 °C. For samples of POM, water samples were pre-sieved through a 200 µm mesh net to remove large particles and then filtered using Whatman GF/F glass fibre (pre-combusted at 550 °C for 5 h). The filter papers were rinsed with ultrapure water, freeze-dried and stored in acid-cleaned polyethylene bags at −80 °C. Samples of SOM were freeze-dried, sieved through a 300 µm stainless steel screen, homogenised and stored in acid-cleaned polyethylene bags at −80 °C. After depuration in filtered water for 24 h, samples of L.fortunei tissues were dissected with a plastic knife and rinsed with ultrapure water.
For each analysis, 10 individuals of L.fortunei were pooled, freeze-dried, homogenised, and stored in acid-cleaned polyethylene bags at −80 °C.
Stable-isotope analyses
For stable-isotope measurements, plankton, POM and SOM samples were acidified with 10% HCl, rinsed with distilled water and oven-dried at 40 °C for 24 h, to remove carbonates (Deniro and Epstein Citation1978). When production of CO2 bubbles ceased, the samples were dried and stored in acid-cleaned polyethylene bags. For analysis, about 1 mg of the powdered sample was packed into a 4 × 6-mm tin capsule.
Samples were combusted in an elemental analyser (Vario MICRO cube, Elementar Analysensysteme GmbH, Lagensebold, Germany) attached to an isotope-ratio mass spectrometer (MAT 253, Thermo Fisher Scientific, Waltham, MA) to determine 13C /12C. The value of δ13C was expressed as the deviation from a standard in parts per thousand (‰) according to the following equation: δ13C = [(Rsample/Rstandard)−1] × 1000 where R is the corresponding ratio of 13C /12C. Carbon values were referenced to standard Pee Dee Belemnite (PDB). Measurements were made with a precision of approximately 0.2‰.
Fatty acid analyses
Lipids were extracted from plankton, POM, SOM and L.fortunei samples following the method of Folch et al. (Citation1957) and Zhao et al. (Citation2013). Lipids were extracted ultrasonically for 10 min using a solvent mixture (two parts chloroform to one part methanol). The lower chloroform phase containing lipids was collected and separated further by centrifugation. The lipid extracts were saponified, transmethylated, separated and purified to transform fatty acids to fatty-acid methyl esters (FAMEs). FAMEs were analysed using a gas chromatograph (GC-9A; Shimadzu, Tokyo, Japan) on a DB-FFAP capillary column (30 m × 0.32 mm internal diameter, 0.25 µm film). Hydrogen was used as the carrier gas. The injector temperature was 250 °C. FAMEs were identified by comparing their retention times with those of standards.
Data analyses
Statistical analyses were performed using SPSS software (Version 19.0; SPSS, Chicago, IL). Significant differences (P < 0.05) in δ13C were tested using Student's t-test and one-way analysis of variance (ANOVA). For fatty acid data, means, standard errors (SE) and Fisher's least significant difference (LSD) post-hoc tests were calculated conventionally.
To evaluate the relative contributions of plankton, POM and SOM to the diet of L.fortunei, the isotope-mixing model (Philips Citation2001) was used with slight modifications. The fractionation of δ13C values for bivalves was set at 0.8 in the model (Fukumori et al. Citation2008), defined as follows: δ13CLf = fpl(δ13Cpl+0.8)+fp(δ13Cp+0.8)+fs(δ13Cs+0.8) where the subscripts Lf, pl, P and S refer to L.fortunei, plankton, POM and SOM, respectively, and fpl, fp and fs are the fractional contributions of plankton, POM and SOM, respectively.
Fatty acids that are commonly used as biochemical markers for particular taxonomic groups that occurred in our study are shown in .
Table 1. Fatty acids as biochemical markers of certain taxonomic groups that occur in the Xijiang River, southern China. SFAs, MUFAs, PUFAs and BrFAs refer to saturated fatty acids, monounsaturated fatty acids, polyunsaturated fatty acids and branched fatty acids, respectively. DHA refers tor C22:6(n-3) and EPA refers to C20:5(n-3).
Results
Characteristics of stable carbon isotopes in plankton, POM, SOM and L.fortunei
Mean δ13C values for plankton, POM, SOM and L.fortunei tissue sample are shown in . Significant differences in δ13C values were observed among plankton, POM, SOM and L.fortunei samples (one-way ANOVA, P < 0.05). The δ13C values varied between −23.38‰ and −20.68‰ for plankton, between −23.52‰ and −20.99‰ for POM, and between −26.72‰ and −19.30‰ for SOM. The δ13C values for L.fortunei ranged from −21.62‰ to −19.91‰.
Table 2. δ13C values (‰) for the plankton, POM, SOM and L.fortunei tissues in the Xijiang River between March and November in 2014. POM, particulate organic matter; SOM, sediment organic matter. δ13C values (‰) are means ± SD (n = 3).
Contribution of plankton, POM and SOM to the dietary regime of L.fortunei
The relative contributions of plankton, POM and SOM in the diet of L.fortunei, calculated using the isotope-mixing model, are presented in . The contribution of POM to the carbon content of L.fortunei ranged between 57.6% and 65.2%, which was significantly higher than that of plankton (19.8%–28.2%) and SOM (10.2%–21.1%) (t-tests, P < 0.05).
Fatty acid profiles of plankton, POM, SOM and L.fortunei
The proportions of 45 prominent FAs in the samples of plankton, POM, SOM and L.fortunei are shown in . The level of saturated fatty acids (SFAs) was highest in SOM (49.73%), followed by POM, plankton and L.fortunei. Among SFAs, C14:0, C16:0 and C18:0 dominated in all samples, although their ratios significantly differed among the samples (one-way ANOVA, P < 0.05). Monoenoic acids (MUFA) ranged between 21.85% and 29.99% in the samples and were primarily represented by C16:1(n-7 + n-9), C18:1(n-7), C18:1(n-9), C20:1(n-9) and C22:1(n-9). However, MUFA profiles differed among L.fortunei and their food items. L.fortunei had highest level of C18:1(n-7) and C20:1(n-9), while plankton, POM and SOM showed significantly higher levels of C16:1(n-7 + n-9) and C22:1(n-9). The levels of polyunsaturated fatty acids (PUFA) varied from 11.23% to 33.93% of the samples. The lowest value for PUFA was found in SOM, while the highest value was in L.fortunei tissue. Dominant PUFAs, shown in bold in , were particularly represented in some taxonomic groups. Branched fatty acids (BrFAs) comprised mostly 15-iso, 16-iso, 16-anteiso and 17-iso and their levels varied from 2.45% to 13.64% of the samples. The levels of 15-iso and 17-iso were significantly higher in SOM than in the other samples ().
Table 3. Fatty acid profiles (%) of plankton, POM, SOM and L.fortunei in the Xijiang River (mean ± SD, n = 3). Values indicate concentration (%), while ‘n.d’ and ‘tr.’ mean non-detected and trace, respectively. Fisher's LSD test was used to compare the fatty acids indicated in bold among samples; values labelled with the same letter are not significantly different (P < 0.05). POM, particulate organic matter; SOM, sediment organic matter. SFAs, saturated fatty acids; MUFAs, monounsaturated fatty acids; PUFAs, polyunsaturated fatty acids; BrFAs, branched fatty acids.
lists the fatty acid biomarkers that were used to identify the food sources of L.fortunei. Compared to its food items, the L.fortunei samples contained higher levels of biomarkers for Cryptophyceae (C18:4(n-3), C20:5(n-3) and C22:6(n-3); (b)) and Dinophyceae (C18:4(n-3), C22:6(n-3), C16:1(n-7)/C16:0 = 0.19, DHA/EPA = 1.28; (c)). In contrast, higher level of biomarkers for Chlorophyceae (C18:3(n-3) and C16:4(n-3); (a)), Bacillariophyceae (C16:2(n-4), C16:3(n-4), C16:1(n-7)/C16:0 = 0.19, DHA/EPA = 1.28; (d)), cyanobacteria (C18:3(n-3) in (e)), decomposed material (C18:0; ) and plant detritus (C20:0 and C22:0; (g)) were found in the food items of L.fortunei.
Figure 3. Fatty acid biomarkers in plankton, POM, SOM and in L.fortunei tissues in the Xijiang River. a, Chlorophyceae; b, Cryptophyceae; c, Dinophyceae; d, Bacillariophyceae; e, Cyanobacteria; f, heterotrophic bacteria; g, plant detritus particle; h, Copepoda. Column shading: white, plankton; grey, POM; diagonal, SOM; cross-hatch, L.fortunei, tissues.
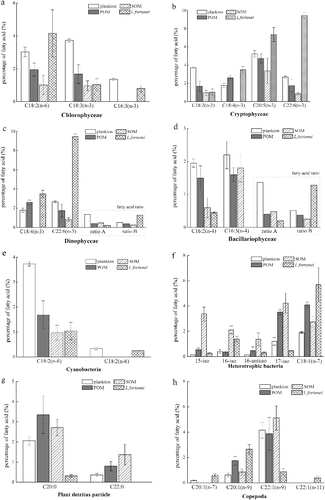
Discussion
In the present study, the δ13C signatures of plankton, POM and SOM were significantly different. These distinct signatures made it possible to calculate the relative contributions of plankton, POM and SOM to the diet of L.fortunei using a mixing model. These calculations indicated that L.fortunei primarily feeds on POM, followed by plankton and SOM (). Previous studies on the feeding ecology of bivalves indicated that Pinctada fucata martensii obtained 78% (Kanaya et al. Citation2005) and Ruditapes philippinarum 61.0% (Fukumori et al. Citation2008) of their carbon from POM in their natural habitats. Our results are consistent with their conclusion that POM is the principal food source of bivalves. The stable-isotope approach assumes a fixed isotopic enrichment between the bivalve and its food items. Ruditapes philippinarum, Mactra veneriformis and Nihonotrypaea japonica were reported to be enriched by 0.6%–2.0% for δ13C relative to POM (Yokoyama et al. Citation2005). Therefore, our observation of the δ13C enrichment of L.fortunei relative to POM (0.67%–2.41%) () is consistent with those findings.
However, plankton, POM and SOM are heterogeneous mixtures of phytoplankton, bacteria, benthic microalgae and other OM (Dalsgaard et al. Citation2003). The isotopic signatures of these sources often overlap in natural conditions, making it difficult to separate specific components (Phillips and Gregg Citation2003). Primary producers, such as diatoms, dinoflagellates and bacteria, are characterised by distinct fatty-acid profiles (Kharlamenko et al. Citation2001). Therefore, these profiles can be used to identify the relative contribution of each component to the plankton, POM and SOM mixtures.
According to the fatty acid profiles of L.fortunei food items (), plankton, POM and SOM differ in the percentages of several fatty acid markers, indicating high levels Chlorophyceae, Cryptophyceae, Bacillariophyceae and cyanobacteria in plankton, high levels of plant detritus in POM, and high levels of heterotrophic bacteria and Copepoda in SOM. Although the dominant taxa in the phytoplankton were Chlorophyceae, Cryptophyceae, Dinophyceae and Bacillariophyceae, fatty acid markers of these algae were not abundant in plankton, and were particularly scarce in POM. The relatively low proportion of algal biomass in the water column is a possible explanation of this result. The higher percentages of SFAs (C18:0, C20:0 and C22:0) and BrFAs in POM and SOM suggest that they contain high proportions of detrital particles derived from plant debris, pseudofaeces, faeces and other molluscan excreta (Makhutova et al. Citation2011).
The fatty acid compositions of various bivalve taxa are highly variable. As in other freshwater bivalve, e.g. D. polymorpha and D. bugensis (Makhutova et al. Citation2011), L.fortunei possesses high levels of C20:5(n-3) and C22:6(n-3), which are considered physiologically crucial and probably are conservatively retained in tissues relative to other compounds (Gladyshev et al. Citation2011; Kelly and Scheibling Citation2012). In addition, many researchers emphasised the importance of the ratio C22:6(n-3)/C20:4(n-6) for the growth and reproduction of the zoobenthos (Ahlfren et al. Citation2009). Data collected from the literature (Makhutova et al. Citation2011) indicated values for the C22:6(n-3)/C20:4(n-6) ratio in D. polymorpha and D. bugensis of 1.44 and 1.49, respectively, and in Potamocorbula amurensis the ratio was ca. 2 (Canuel et al. Citation1995). In the present study, the ratio in L. fortunei was somewhat higher (2.02; ).
Algal fatty acids are used as energetic resources and are catabolised in animal tissues (Brett and Goldman Citation2006; Gladyshev et al. Citation2011). The percentage of C18:2(n-6) (a), an essential fatty acid synthesised by algae (Maria-José et al. Citation2010), was higher in L.fortunei than in its food items. Likewise, percentages of the essential fatty acid, C18:4(n-3), which was abundant in Cryptophyceae and Dinophyceae (Gugger et al. Citation2002; Maria-José et al. Citation2010), was also higher in L.fortunei (). Apparently, L.fortunei consumed or selectively accumulated C18:2(n-6) and C18:4(n-3) from certain algae species, i.e. Chlorophyceae, Cryptophyceae and Dinophyceae. In contrast, Bacillariophyceae are abundant in plankton, POM and SOM, but are deficient in L.fortunei ((d)). Nevertheless, we could not estimate the consumption of Bacillariophyceae by L.fortunei by using fatty-acid markers because bivalves are reported to preferentially store fatty acids from Bacillariophyceae as triacylglycerols and to use them for catabolism (Gladyshev et al. Citation2011). Heterotrophic bacterial fatty acids are reported to be a significant source of organic carbon and nitrogen for some bivalve molluscs (Nichols and Garling Citation2000). Bacterial taxa differ markedly in fatty markers (Napolitano Citation1999), e.g. bacterial i17:0 and 17:0 for sulfate-reducing bacteria, and C18:1(n-7) for cyanobacteria, sulphur-oxidising and aerobic bacteria. Thus, L.fortunei in the present paper apparently consumed the latter bacteria, which usually dwell in the water column. Decomposed material, particles of plant detritus and copepods can also be potential food sources for L.fortunei but the high percentage of their fatty acid markers in plankton, POM and SOM, and low levels in L.fortunei indicated that L.fortunei did not prefer these food items. The high level of the biomarkers of terrestrial OM in L.fortunei () suggest the indirect assimilation of terrestrial OM via heterotrophic bacteria, but could also result from the direct assimilation of terrestrial OM using cellulase and hemicellulase in this species (Mcleod and Wing Citation2009).
According to the fatty-acid marker analyses, L.fortunei preferred planktonic algae and bacteria. This feeding spectrum is similar to that of D. polymorpha (Cole and Solomon Citation2012; Makhutova et al. Citation2013) and could be explained in terms of active selection, more efficient assimilation of the selected diet from POM and/or preferential ingestion. Although some bivalve species appear to capture their diet indiscriminately (Ward et al. Citation1997), L.fortunei is able to differentiate between suitable and unsuitable particles by gill sorting mechanisms. This selection mechanism appears not to be based on particle size but on chemical cues and their surface properties (Wong and Cheung Citation1999).
Conclusions
The feeding spectrum of L.fortunei in the Xijiang River in summer was described. Using the isotope-mixing model, the relative contributions to the diet of L.fortunei were estimated as 19.8%–28.2% for plankton, 57.6%–65.2% for POM and 10.2%–21.1% for SOM. Fatty acid biomarkers specific to Chlorophyceae, Cryptophyceae, Dinophyceae, heterotrophic bacteria and terrestrial OM were identified in the tissues of L.fortunei, indicating that there were substantial algal, bacterial and terrestrial inputs into the diet of L.fortunei. However, the present study did not include the winter months. The possibility of seasonal variation in the levels of plankton, POM and SOM in the water column should be addressed in future research.
Acknowledgments
The authors would like to acknowledge the financial support for this work provided as follows:
First, National Natural Science Foundation of China (Grants No. 51608140, Grants No. 51778164 and Grants No. 41401355); second, the Academician Workstation for urban environment governance in Guangzhou Municipal Engineering Design & Research Institute, (Grants No.2013B090400006); third, the Guangdong Provincial Department of Education Project of production, teaching, research cooperation (Grants No. 2012B091000055).
Disclosure statement
The authors declare that they have no conflict of interest.
Additional information
Funding
Notes on contributors
Ruijian Zhang
Ruijian Zhang received doctoral degree from South China University of Technology. His research focuses on L.fortunei control.
Bin Cui
Bin Cui is an associate professor of the school of civil engineering, Guangzhou University. She received doctoral degree from South China University of Technology. Her research focuses on L.fortunei control and water pollution control.
Zhimin Sun
Zhimin Sun is a professor of the Guangzhou Municipal Engineering Design & Research Institute. He received doctoral degree from the Harbin Institute of Technolog. His research focuses on water pollution control.
Wuyang Zhou
Wuyang Zhou received his MSc degree from the school of civil engineering, Guangzhou University. His research focuses on water pollution control.
Pengfei Ren
Pengfei Ren received doctoral degree from the Harbin Institute of Technolog. His research focuses on water pollution control.
Zhili Du
Zhili Du received his MSc degree from the Illinois Institute of Technology (iit). His research focuses on water pollution control.
Nanhao He
Nanhao He is currently an MSc candidate in School of Municipal and Environmental Engineering, Shenyang Jianzhu University, under the direction of professor Baiyi Jiang. His research focuses on water pollution control.
Jing Lu
Jing Lu is an associate professor of School of Environment and Safety Engineering, North University of China. She received doctoral degree from South China University of Technology. Her research focuses on water pollution control.
References
- Ahlgren G, Vrede T, Goedkoop W. 2009. Fatty acid ratios in freshwater fish, zooplankton and zoobenthos - are there specific optima? Lipids Aquat Ecosyst. 147–178.
- Asante KA, Agusa T, Kubota R, Mochizuki H, Ramu K, Nishida S, Ohta S, Yeh H-M, Subramanian A, Tanabe S. 2010. Trace elements and stable isotope ratios (δ;13C and δ;15N) in fish from deep-waters of the Sulu Sea and the Celebes Sea. Mar Pollut Bull. 60(9):1560–1570.
- Bergé JP, Barnathan G. 2005. Fatty acids from lipids of marine organisms: molecular biodiversity, roles as biomarkers, biologically active compounds, and economical aspects. Adv Biochem Eng Biotechnol. 96(10):49.
- Boltovskoy D, Sylvester F, Otaegui A, Leites V, Cataldo DH. 2009. Environmental modulation of reproductive activity of the invasive mussel Limnoperna fortunei: implications for antifouling strategies. Austral Ecol. 34(7):719–730.
- Braeckman U, Provoost P, Sabbe K, Soetaert K, Middelburg JJ, Vincx M, Vanaverbeke J. 2015. Temporal dynamics in a shallow coastal benthic food web: Insights from fatty acid biomarkers and their stable isotopes. Mar Environ Res. 108:55–68.
- Brett MT, Goldman CR. 2006. Daphnia fatty acid composition reflects that of their diet. Limnol Oceanogr. 51(5):2428–2437.
- Brett MT, Müllernavarra DC, Persson J. 2009. Crustacean zooplankton fatty acid composition. Lipids Aquat Ecosyst. 115–146.
- Canuel EA, Cloern JE, Ringelberg DB, Guckert JB, Rau GH. 1995. Molecular and isotopic tracers used to examine sources of organic matter and its incorporation into the food webs of San Francisco bay. Limnol Oceanogr. 40(1):67–81.
- Cole JJ, Solomon CT. 2012. Terrestrial support of zebra mussels and the Hudson River food web: A multi-isotope, Bayesian analysis. Limnol Oceanogr. 57(6):1802–1815.
- Dalsgaard J, St JM, Kattner G, Müller-Navarra D, Hagen W. 2003. Fatty acid trophic markers in the pelagic marine environment. Adv Mar Biol. 46(6):225.
- Deniro MJ, Epstein S. 1978. Influence of diet on the distribution of carbon isotopes in animals. Geochim Cosmochim Acta. 42(5):495–506.
- Desvilettes C, Bourdier G, Amblard C, Barth B. 1997. Use of fatty acids for the assessment of zooplankton grazing on bacteria, protozoans and microalgae. Freshwater Biol. 38(3):629–637.
- Di FE, Pizarro H, Dos SAM, Cataldo D. 2012. Impact of the invasive mussel Limnoperna fortunei on glyphosate concentration in water. Ecotoxicol Environ Saf. 81(6):106–113.
- Dijkman NA, Kromkamp JC. 2006. Phospholipid-derived fatty acids as chemotaxonomic markers for phytoplankton: application for inferring phytoplankton composition. Marine Ecol Progr. 324(1):113–125.
- Folch J, Lees M, Stanley GHS. 1957. A simple method for the isolation and purification of total lipids from animal tissues. J Biol Chem. 226(1):497–509.
- Fukumori K, Oi M, Doi H, Okuda N, Yamaguchi H, Kuwae M, Miyasaka H, Yoshino K, Koizumi Y, Omori K, et al. 2008. Food sources of the pearl oyster in coastal ecosystems of Japan: evidence from diet and stable isotope analysis. 76(3):704–709.
- Gladyshev MI, Sushchik NN, Anishchenko OV, Makhutova ON, Kolmakov VI, Kalachova GS, Kolmakova AA, Dubovskaya OP. 2011. Efficiency of transfer of essential polyunsaturated fatty acids versus organic carbon from producers to consumers in a eutrophic reservoir. Oecologia. 165(2):521–531.
- Green CT, Scow KM. 2000. Analysis of phospholipid fatty acids (PLFA) to characterize microbial communities in aquifers. Hydrogeol J. 8(1):126–141.
- Guevara M, Bastardo L, Cortez R, Arredondo-Vega B, Romero L, Gómez P. 2011. Rhodomonas salina (Cryptophyta) pastes as feed for Brachionus plicatilis (Rotifera). Rev Biol Trop. 59(4):1503–1515.
- Gugger M, Lyra C, Suominen I, Tsitko I, Humbert JF, Salkinoja-Salonen MS, Sivonen K. 2002. Cellular fatty acids as chemotaxonomic markers of the genera Anabaena, Aphanizomenon, Microcystis, Nostoc and Planktothrix (cyanobacteria). Int J Syst Evol Microbiol. 52(3):1007–1015.
- Hagen W. 1993. Calanoides acutus and Calanus propinquus, Antarctic copepods with different lipid storage modes via wax esters or triacylglycerols. Marine Ecol Progr. 97(2):135–142.
- Hama T. 1999. Fatty acid composition of particulate matter and photosynthetic products in subarctic and subtropical Pacific. J Plankton Res. 21(7):1355–1372.
- Kanaya G, Nobata E, Toya T, Kikuchi E. 2005. Effects of different feeding habits of three bivalve species on sediment characteristics and benthic diatom abundance. Marine Ecol Progr. 299(1):67–78.
- Kang CK, Sauriau PG, Richard P, Blanchard GF. 1999. Food sources of the infaunal suspension-feeding bivalve Cerastoderma edule in a muddy sandflat of Marennes-Oléron Bay, as determined by analyses of carbon and nitrogen stable isotopes. Marine Ecol Progr. 187(1):147–158.
- Kattner G, Hagen W, Lee RF, Campbell R, Deibel D, Falk-Petersen S, Graeve M, Hansen BW, Hirche HJ, Jónasdóttir SH, et al. 2007. Perspectives on marine zooplankton lipids. Can J Fish AquatSci. 64(11):1628–1639.
- Kelly JR, Scheibling RE. 2012. Fatty acids as dietary tracers in benthic food webs. Marine Ecol Progr. 446(1):1–22.
- Kharlamenko VI, Kiyashko SI, Imbs AB, Vyshkvartzev DI. 2001. Identification of food sources of invertebrates from the seagrass Zostera marina community using carbon and sulfur stable isotope ratio and fatty acid analyses. Marine Ecol Progr. 220(1):103–117.
- Kürten B, Frutos I, Struck U, Painting SJ, Polunin NVC, Middelburg JJ. 2013. Trophodynamics and functional feeding groups of North Sea fauna: a combined stable isotope and fatty acid approach. Biogeochemistry. 113(1):189–212.
- Lehane C, Davenport J. 2004. Ingestion of bivalve larvae by Mytilus edulis: experimental and field demonstrations of larviphagy in farmed blue mussels. Mar Biol. 145(1):101–107.
- Makhutova ON, Protasov AA, Gladyshev MI. 2013. Feeding spectra of bivalve mollusks Unio and Dreissena from Kanevskoe Reservoir, Ukraine: are they food competitors or not? Zool Stud. 52(1):1–10.
- Makhutova ON, Sushchik NN, Gladyshev MI, Ageev AV, Pryanichnikova EG, Kalachova GS. 2011. Is the fatty acid composition of freshwater zoobenthic invertebrates controlled by phylogenetic or trophic factors? Lipids. 46(8):709–721.
- Maria-José C, Boschker HTS, Wim A. 2010. Fatty acid profiles of algae mark the development and composition of harpacticoid copepods[J]. Freshwater Biol. 53(1):77–90.
- Mcleod RJ, Wing SR. 2009. Strong pathways for incorporation of terrestrially derived organic matter into benthic communities. Estuar Coast Shelf Sci. 82(4):645–653.
- Molina FR, Paggi JC, Devercelli M. 2010. Zooplanktophagy in the natural diet and selectivity of the invasive mollusk Limnoperna fortunei. Biol Invasions. 12(6):1647–1659.
- Najdek M, Blažina M, Ezgeta-Balić D, Peharda M. 2013. Diets of fan shells (Pinna nobilis) of different sizes: fatty acid profiling of digestive gland and adductor muscle. Mar Biol. 160(4):921–930.
- Napolitano GE. 1999. Fatty acids as trophic and chemical markers in freshwater ecosystems. Lipids Freshwater Ecosyst. 21–44.
- Nichols SJ, Garling D. 2000. Food-web dynamics and trophic-level interactions in a multispecies community of freshwater unionids. Can J Zool. 78(5):871–882.
- Nishino M. 2012. Ecological Changes in Lake Biwa: Interactions between Nature and People. 155–238.
- Paolucci EM, Thuesen EV, Cataldo DH, Boltovskoy D. 2010. Veligers of an introduced bivalve, Limnoperna fortunei, are a new food resource that enhances growth of larval fish in the Paraná River (South America). Freshwater Biol. 55(9):1831–1844.
- Peterson BJ, Fry B. 1987. Stable isotopes in ecosystem studies. Annu Rev Ecol Systemat. 18(1):293–320.
- Petkov G, Garcia G. 2007. Which are fatty acids of the green alga Chlorella? Biochem Syst Ecol. 35(5):281–285.
- Phillips DL. 2001. Mixing models in analyses of diet using multiple stable isotopes: a critique. Oecologia. 127(2):166–170.
- Phillips DL, Gregg JW. 2003. Source partitioning using stable isotopes: coping with too many sources. Oecologia. 136(2):261–269.
- Prato E, Danieli A, Maffia M, Biandolino F. 2012. Lipid contents and fatty acid compositions of idotea baltica and Sphaeroma serratum (Crustacea: Isopoda) as indicators of food sources. Zool Stud. 51(1):38–50.
- Shin KH, Hama T, Yoshie N, Noriki S, Tsunogai S. 2000. Dynamics of fatty acids in newly biosynthesized phytoplankton cells and seston during a spring bloom off the west coast of Hokkaido Island, Japan. Mar Chem. 70(4):243–256.
- Shin PKS, Yip KM, Xu WZ, Wong WH, Cheung SG. 2008. Fatty acid as markers to demonstrating trophic relationships among diatoms, rotifers and green-lipped mussels. J Exp Mar Biol Ecol. 357(1):75–84.
- Shorland FB. 1963. The distribution of fatty acids in plant lipids - chemical plant taxonomy - CHAPTER 10. Chem Plant Taxon. 253–311.
- Sun Z, Gao Q, Dong S, Shin PKS, Wang F. 2012. Estimates of carbon turnover rates in the sea cucumber Apostichopus japonicus (Selenka) using stable isotope analysis: the role of metabolism and growth. Marine Ecol Progr. 457(405):101–112.
- The surface water environmental quality standard (GB3838-2002). Beijing: China Environmental Science Press; 2002. (in Chinese)
- Vaughn CC, Hakenkamp CC. 2001. The functional role of burrowing bivalves in freshwater ecosystems. Freshwater Biol. 46(11):1431–1446.
- Viso AC, Marty JC. 1993. Fatty acids from 28 marine microalgae. Phytochemistry. 34(6):1521–1533.
- Ward JE, Levinton JS, Shumway SE, Cucci T. 1997. Site of particle selection in a bivalve mollusc. Nature. 390(6656):131–132.
- Wong WH, Cheung SG. 1999. Feeding behaviour of the green mussel, Perna viridis, (L.): responses to variation in seston quantity and quality. J Exp Mar Biol Ecol. 236(2):191–207.
- Xu M, Wang Z, Duan X, Zhuang M, Souza FTD. 2009. Ecological measures of controlling invasion of golden mussel (Limnoperna fortunei) in water transfer systemsn water transfer systems. Water Engineering for A Sustainable Environment.
- Yokoyama H, Tamaki A, Harada K, Shimoda K, Koyama K, Ishihi Y. 2005. Variability of diet-tissue isotopic fractionation in estuarine macrobenthos. Marine Ecol Progr. 296(1):115–128.
- Zhang R, Cui B, Huang S. 2014. Algae consumption and nitrate removal in a raw water transport system by Limnoperna fortunei and its associated microorganisms. Water Environ Res. 86(12):2301–2308.
- Zhang R, Cui B, Huang S. 2015. Degradation of forchlorfenuron by nitrification and denitrification reactions in the gut and shell biofilm of Limnoperna fortunei. Ecotoxicology. 24(2):381–90.
- Zhao L, Yang F, Yan X. 2013. Stable isotopes and fatty acids as dietary tracers of intertidal bivalves. Fish Sci. 79(5):749–756.