ABSTRACT
Nearly 20,000 adult caddisfly specimens were collected during May through September 2010–2014 from approximately weekly ultraviolet light samples of Fairbanks Creek in northern Lower Michigan. Detrended correspondence analysis determined four distinct seasonal assemblages corresponding to spring (May to late June), summer (late June to late July), late summer (late July to early September), and autumn (early to late September). Of the 109 total species caught, 5 indicated the spring assemblage, 13 the summer assemblage, 1 the late summer assemblage, and 4 the autumn assemblage. Species richness, specimen abundance, and number of unique species were highest during the summer. Most summer samples contained ∼50% of all species caught during the year; whereas the spring and autumn assemblage contained a smaller but unique fauna, and the late summer assemblage was characterized by a decreasing richness of summer species. Pollution tolerance and shredder to filtering collector ratio metrics varied by factors of 5–30x; both were highly dependent on the abundance of particular species. Overall mean values of all metrics varied seasonally but not annually. The specific date of peak species richness varied based on annual weather, but occurred at a similar degree-day value for all 5 years. These results suggest the importance of sampling within a season when comparing streams for biological monitoring purposes. Moreover, they confirm the comparability of samples taken from different years, provided such samples are collected during the same season.
Introduction
Due to the ecological importance of caddisflies in nearly all types of aquatic ecosystems, as well as their utility in biological monitoring protocols (Barbour et al. Citation1999), it is imperative to sample their assemblages representatively. A common device for sampling the positively phototaxic winged adult stage is the ultraviolet light trap (Wright et al. Citation2013; Boda et al. Citation2014; Brakel et al. Citation2015). This device consists of a battery-powered 8-watt ultraviolet light placed over a white pan filled with a killing agent such as 70% EtOH. The traps are placed near aquatic habitats at dusk and typically left out for 1–2 hours.
Adult sampling has several advantages over larval collecting. First, ultraviolet lights are unmanned techniques, allowing for several collections at once. Second, because winged adults have left the natal habitat, the difficulty of sampling all stream microhabitats is removed (Houghton, Berry et al. Citation2011). Third, adult specimens – assuming taxonomic keys and expertise are available – can usually be identified to the species level, whereas most larvae cannot. Species identification yields more information for biological monitoring studies and is necessary for faunistic studies (Hawkins et al. Citation2000). Recent studies have utilized adult caddisfly assemblages to assess differences in seasonal flight (Kimura et al. Citation2008; Houghton Citation2015b), ecosystem type (Kimura et al. Citation2006; Blinn and Ruiter Citation2013; Houghton Citation2015a), changes in water flow (Waringer Citation2003), land use disturbance (Collier et al. Citation1997; Houghton Citation2006, Citation2007), water quality (Sommerhäuser et al. Citation1999; Dohet Citation2002), and changes in riparian habitat (Houghton and Wasson Citation2013).
Unfortunately, several features of adult caddisflies render their use in assessing aquatic ecosystems problematic. Weather variables such as temperature, wind speed, precipitation, and humidity have been observed to affect the abundance and composition of light trap samples (Waringer Citation1989; Anderson and Vondracek Citation1999). The specific length of sampling period can also affect captured assemblages since different species are active during different periods throughout a night (Wright et al. Citation2013; Brakel et al. Citation2015). Assemblages change seasonally as different species mature throughout the year (Houghton Citation2015b). Thus, an early summer light trap sample may not be comparable to a late summer sample. Likewise, light traps from different years may or may not be comparable to each other.
While many studies have looked at the seasonal flight periodicity of individual caddisfly species (Huryn and Wallace Citation2000), fewer have assessed the flight periodicity of an entire assemblage. And, since nearly all assemblage studies have occurred over a single year (Swegman et al. Citation1981; Krueger and Cook Citation1984; Singh et al. Citation1984; Dobrin and Giberson Citation2003), annual changes in assemblages have not been well studied. If caddisfly adults are going to be properly used to compare conditions between ecosystems, then it is important to assess how their assemblages change between seasons and between years.
The objective of this study was to monitor the changes in a single assemblage of adult caddisflies over a 5-year period. By sampling with the same protocol and under similar weather conditions during each of the sampling nights throughout the 5 years, differences between seasons and years can be assessed. Moreover, due to the longer study period, any potential determined patterns can be viewed with greater confidence.
Materials and methods
Caddisfly assemblages were studied from a single site of Fairbanks Creek (N 44.046°, W 85.674°), located in northwestern Lower Michigan, USA. Fairbanks Creek is a first-order stream with a protected watershed and intact riparian canopy throughout its continuum to the study site (Houghton and Wasson Citation2013). The study site was <1 km downstream from a 20-ha mesotrophic lake, had predominantly sand and gravel substrate, and was covered by a dense riparian canopy composed primarily of Thuja occidentalis (L.), Quercus rubra (L.), Betula papyrifera Marshall, and Acer rubrum (L.)
Adult caddisflies were sampled approximately weekly from mid-May to late September or early October 2010–2014 using the ultraviolet light traps described above. Each trap was placed ∼1 m from the stream bank at dusk and retrieved 1 hour after dusk. To eliminate weather as a capture-success variable, samples were collected from May to September only if the peak daytime temperature was >25 °C, dusk temperature was >18 °C, and there was no noticeable wind or precipitation at dusk (Houghton Citation2004). During the cooler months of September and October, samples were collected if peak daytime temperature was >21 °C and dusk temperature was >13 °C. Since caddisflies collected within 15 m of a habitat accurately reflect the assemblage of that habitat (Petersen et al. Citation1999; Brakel et al. Citation2015), occasional dispersals of adults from other streams, while certainly possible, were considered unimportant. All specimens were identified to the species level using Houghton (Citation2012) and were stored in the Hillsdale College Insect Collection.
Samples were examined for patterns in their caddisfly assemblages with detrended correspondence analysis (DCA) using the program PC-ORD for Windows® (McCune and Medford Citation2006) with the default settings. The DCA was performed on a 2-dimensional data matrix of each sample by species presence (‘1’) or absence (‘0’). All species were weighted equally in the analysis.
Once distinct assemblages were determined by DCA, individual species were tested for their value to indicate such assemblages with Dufrêne and Legendre's (Citation1997) indicator species technique also using PC-ORD with the default settings. For both the DCA and indicator species analysis, a Monte Carlo test was added to assess the significance of determined values. Indicator species analysis determines a species’ indicator value based on a combination of the percentage of samples within a seasonal assemblage that contain a particular species, and the average abundance of that species within each assemblage divided by the average abundance of that species in all assemblages. Combining these frequency and abundance values yields an overall indicator value, which is expressed as a percentage of perfect indication. Thus, in order to be a significant indicator, a species needed to be common and abundant in some seasonal assemblages but not in others.
Five different metrics were calculated from adult caddisfly data to assess their annual and seasonal trends (). Specimen abundance, species richness, and number of species caught on only a single date per year were determined. Hillsenhoff's tolerance index (Hilsenhoff Citation1987; Bode et al. Citation1991; Barbour et al. Citation1999) was calculated at the species level when species-level values were available and at the genus level when they were not. The abundance of specimens within the filtering collector and shredder functional groups (Merritt et al. Citation2008) were determined, and the ratio of shredder to filtering collector specimens was calculated. Because samples were not collected on the same day every year, in order to calculate a mean value for each metric during the 5-year period, each year was divided into 52 weeks. Samples from each of the five years collected during the same week were considered as occurring on the same date and were averaged to determine an overall mean for that week. A 2-way analysis of variance was calculated for the 10 weeks during the year in which all 5 years had samples to determine the significance of seasonal and annual changes on the five metrics. Mean species–sample curves were also constructed from the 5 years of data.
Table 1. Summary table for 2-way analysis of variance performed on the five metrics, based on adult caddisfly data over 10 weeks in each of the 5 years of the study.
The water temperature of this site on every Friday afternoon during 2010–2014 was determined and reported in a previous study (Houghton Citation2015b). The current study utilized the same data. To determine the effects of stream temperature variation on species richness, degree-days were calculated for each year of this study by starting at the first Friday of each year and adding the temperature for each Friday to the accumulated temperature values from the previous Fridays. In order to compare recent historical weather to that of the years of the study, mean annual temperatures were also determined from the Wexford Airport weather station (N44.2753, W85.4189), the station nearest (∼40 km) to the study site, for the last 20 years (www.wunderground.com).
The effect of lunar phase on species richness, specimen abundance, and number of unique species was estimated using QuickPhase Pro for Windows® Software (www.moonconnection.com/quickphase/). The percentage of full (100%) moon was determined for each sampling date based on coordinates N44°and W85°. Simple linear regression models of the number of specimens or species caught (dependent variable) based on percentage of full moon (independent variable) were calculated for each of the three metrics for each of the 5 years.
Results
A total of 19,642 specimens representing 109 species were collected during the 5 years of this study. Eighty-five species were collected in 2014, 82 in 2012, 79 in 2013, 77 in 2010, and 74 in 2011. A total of 16 samples were collected in 2011; all other years had 18 samples, for a grand total of 88 samples. Ordination of these 88 samples by DCA produced two significant axes of ecological interest (). Axis 1 had an eigenvalue of 0.473 (P = 0.001) and axis 2 of 0.269 (P = 0.001). A third produced axis was not significant. Although there were some outlier samples, DCA suggested four seasonal assemblages, corresponding to the spring (May to late June), summer (late June to late July), late summer (late July to early September), and autumn (early to late September).
Figure 1. Detrended correspondence analysis ordination of the 88 samples from the 5 years of this study, suggesting four seasonal caddisfly assemblages.
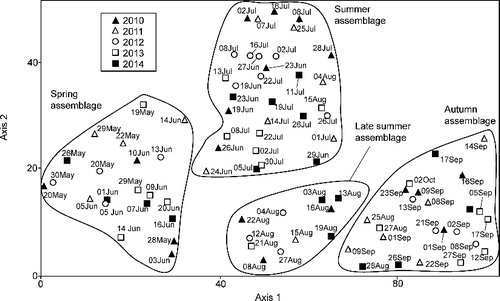
Mean species richness was low in the early spring, increased into mid-June, and dipped slightly in late June (). This dip approximated the boundary between the spring and summer assemblages (). Species richness then increased again to a peak in early to mid-July before decreasing into late August. This decrease approximated the boundary between summer and late summer assemblages, although it was less distinct than the spring/summer boundary. A small increase then occurred in early September, approximating the beginning of the autumn assemblage, before a gradual decrease to the end of the collecting season. The number of species caught on only one single date and the total number of specimens caught both followed a similar seasonal pattern (). All three metrics were different between weeks, but not between years (). Peak emergence was 1 week earlier in 2010 and 2012 then it was in 2011 and 2013, and 2 weeks earlier than in 2014 (). The mean species–sample curve exhibited a sharp increase from May until the end of the July, with the greatest variability between years occurring in June (). Few additional species were caught during August, and a few additional species were caught during September.
Figure 2. Total number of adult caddisfly species caught per sampling date (A), number of species caught only on a single date per year (B), and total number of specimens (C). Markers indicate actual date of sample. Means were calculated per week.
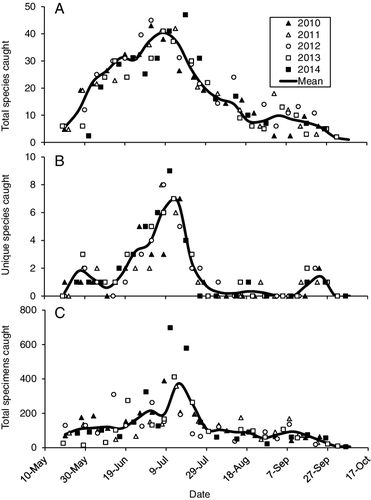
Figure 3. Mean (±SE) cumulative percentage of caddisfly species caught per collecting date for all 5 years of the study.
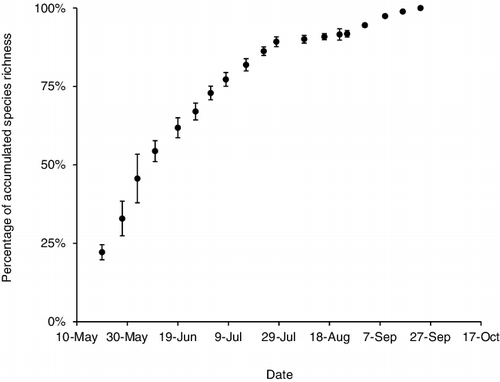
Mean tolerance value for the site was <1 during spring, increased to ∼3 for much of the summer, decreased to ∼2 in August, and then increased to ∼4 in autumn (). Mean shredder to filtering collector ratio fluctuated between 2–10 from May through July, before increasing to ∼75 during September. Both metrics were different between weeks, but not between years ().
Figure 4. Mean pollution tolerance value based on adult caddisflies per sampling date (A), and the Log10 shredder to filtering collector ratio for each sampling date (B). Markers indicate actual date of sample. Means were calculated per week.
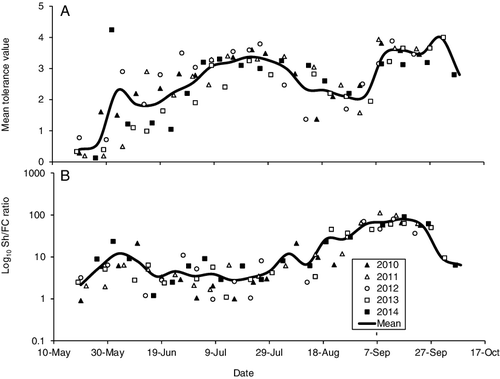
Of the 109 total species caught, 23 had significant indicator values for a particular assemblage. Five species indicated the spring assemblage, 13 the summer assemblage, 1 the late summer assemblage, and 4 the autumn assemblage (). Three species indicated >1 assemblage.
Table 2. Overall indicator values (IV) and associated P values for the 23 significant indicator species of the four seasonal assemblages of caddisflies in Fairbanks Creek. Numbers below seasonal assemblages denote the percentage of perfect indication based on a combination of species relative abundance and relative frequency in a particular assemblage. Species are arranged in approximate descending IV value for each assemblage. TFG: trophic functional group. GC: gathering collectors, FC: filtering collectors, Pr: predators, Sc: scrapers, Sh: shredders. Tol: Hillsenhoff pollution tolerance values (Hilsenhoff Citation1987).
Based on mean daily air temperature for the year measured at the Wexford Airport weather station, 2014 (5.1 °C) was the coldest year of the last 20; whereas, 2012 (8.3 °C) was the warmest since 1999. The other years were within a standard deviation (1.1) of the mean (6.9 °C) of the last 20 years (www.wunderground.com). The year 2012 was the warmest, followed by 2010, 2011, 2013, and 2014. Overall caddisfly species richness patterns relative to degree-day were similar for all 5 years, with the flight period beginning at ∼100 accumulated degree-days, peak species richness occurring at ∼200, and the flight period ending at 350–450 every year (). All regression models calculated using lunar phase as a predictor of specimen abundance, species richness, or number of unique species had R2 values <0.20.
Figure 5. The number of species caught per year based on accumulated degree-days. Markers indicate actual number of species caught, while binomial fit lines show seasonal trends. Temperature data from Houghton (Citation2015b).
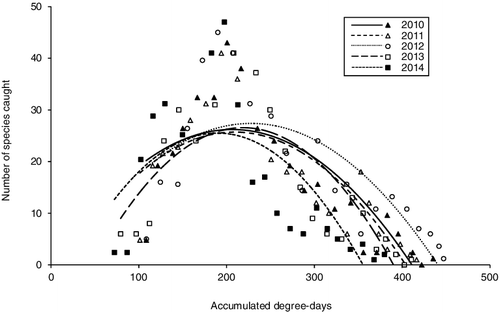
Discussion
It appears, based on adult caddisfly flight periodicity, four distinct seasonal assemblages exist in Fairbanks Creek. This pattern persisted for all 5 years of the study. Each assemblage was indicated by particular species.
The spring assemblage occurred during May and into late June, and was indicated by Parapsyche apicalis (Banks), Goera stylata Ross, Nemotaulis hostilis (Hagen), Hydatophylax argus (Harris), and Lepidostoma vernale (Banks). All of these species have been shown previously to emerge early in the season (Singh et al Citation1984; Williams and Hogg Citation1988; Dobrin and Giberson Citation2003; Houghton Citation2015b). The precise beginning of this assemblage was not clear due to the cool weather precluding effective blacklighting early in the season. Moreover, the precise beginning is probably affected by annual differences in stream temperatures. Such differences are known to alter the adult flight periods of G. stylata, P. apicalis, L. vernale, and probably other spring-emerging species (Houghton Citation2015b).
The summer assemblage lasted from late June until late July. Samples of this assemblage contained the most specimens, the most species, the most unique species, and the most indicator species. Most sampling dates within this period contained ∼50% of the total species richness for the entire year. This result is consistent with previous studies showing peak richness during July in temperate woodland streams (Singh et al. Citation1984; Dobrin and Giberson Citation2003; Houghton, Brandin et al. Citation2011).
The late summer assemblage lasted from late July until early September. It contained the fewest species, and was only indicated by a single species: Lepidostoma griseum (Banks). In fact, L. griseum was the only species caught exclusively during late summer. Richness decreased throughout the late summer interval as summer emergent species disappeared from the assemblage.
The autumn assemblage lasted from early September until late September or early October. It was indicated by Onocosmoecus unicolor (Banks), Neophylax oligius Ross, and two species of Pycnopsyche. All of these species are known to emerge in the autumn (Cummins Citation1964; Moulton and Stewart Citation1996; Houghton Citation2015b). Pycnopsyche guttifera (Walker) was, by far, the most abundant species of the autumn, sometimes composing >90% of the sample. Similar to the spring assemblage, a definite determination of the assemblage ending point was not possible due to cool weather precluding effective blacklighting. Other common woodland species, such as Frenesia missa (Milne) or Glyphosyche irrorata (F.), that emerge even later in the season (Houghton Citation2012), may have been missed.
Indicator species are typically used to designate environments with similar climate, community composition, or disturbance status (Bakker Citation2008). Since the capture of this small number of species indicates a particular ecosystem or set of conditions, it provides a relatively easy method for ecosystem assessment. Although using indicator species to indicate seasonal assemblages is not commonly done (Carignan and Villard Citation2002), the principle is similar: confirming a particular seasonal assemblage with the capture of its particular indicator species.
Overall, this study confirmed differences among caddisfly assemblages throughout the collecting season. While it is well known that assemblages change as individual species complete their larval life cycle and emerge as adults (Dobrin and Giberson Citation2003; Houghton Citation2015b), this study demonstrates how and when such assemblages change throughout the season. Assuming that the patterns established in Fairbanks Creek are generally representative of streams throughout the northcentral US, then aquatic entomologists and water quality managers will need to choose a single season within which to compare assemblages between streams. Inadvertently sampling different seasonal assemblages, even by just a couple of weeks, would yield fundamentally different species assemblages.
Moreover, even if a taxonomic comparison is not the objective of the study, seasonal consistency will still need to be maintained, since the two studied water quality metrics also changed seasonally. Differences in tolerance values among assemblages in this study were due to the specific species emerging at particular times. All of the indicator species of the spring assemblage, particularly Goera stylata and Parapsyche apicalis, had low (0–2) tolerance values, whereas the abundant species of the autumn, P. guttifera, had a higher value (4) (Barbour et al. Citation1999). Differences in the shredder to filtering collector ratio between assemblages were, likewise, strongly influenced by the specific species found at a particular time. The spring emergent indicator species constituted a mixture of feeding groups, whereas the abundant shredder P. guttifera dominated the overall autumn assemblage and increased the overall ratio. Moreover, since specimen abundance was relatively low in the spring and autumn, any abundant species had a strong influence on the overall result. The summer assemblage had higher species richness and specimen abundance to mitigate the effects of a single abundant species.
This study also confirmed the general consistency of assemblages between years. None of the five tested metrics varied significantly from year to year, despite the dates of this study encompassing both the warmest and the coldest years so far of the 2000s. Likewise, lunar phase had no discernable impact on adult flight. These observations suggest that aquatic scientists can compare assemblages and water quality metrics with confidence between years within the same season.
Despite the overall assemblage similarity between years, researchers should be careful when comparing samples from different years due to slight deviations in the timing of seasonal assemblages. Patterns of species richness did appear affected by warming water temperatures, and peaked at a consistent accumulated degree-day value (∼200) on slightly different dates. This observation suggests that, while assemblage patterns are generally consistent between years, they are also affected by annual weather differences. Annual weather differences are known to affect the life cycles of many aquatic insect species; relationship between temperature and development rate is typically linear up to a species’ thermal optimum (Trudgill et al Citation2005). Species that emerge in the spring or autumn are particularly affected by small annual changes in temperature (Huryn and Wallace Citation2000; Li et al. Citation2011; Houghton Citation2015b), so it makes sense that such differences would also affect the collective assemblage.
While over extrapolation from a single stream site can be risky, the patterns found during the 5 years of this study do suggest some general recommendations. The best time period to sample adult caddisflies will depend on the overall objective of the study. For example, if exhaustive sampling of Fairbanks Creek was the goal, then sampling would have been necessary during all four seasons in order to collect all species. Sampling only during spring, summer, and autumn would have collected all but one species. Sampling on a single date during late June to mid-July would have collected ≥50% of all species caught for the year. If representative sampling of Fairbanks Creek for biological monitoring was the goal, then this same late June to mid-July time frame would probably be optimal since its overall high specimen abundance mitigates against particularly abundant species, and its high species richness likely yields a more representative sample of stream conditions (Cao and Hawkins Citation2011). Any time period could be theoretically effective for general comparison purposes between streams as long as researchers were confident that the same seasonal assemblage was being sampled.
Further research will be necessary to clarify the limits of the observations found in this study. In particular, lower latitude streams may have different trends in flight patterns due to more consistent weather in different seasons. Larger rivers will be dominated by different species and trophic functional groups, and will have a different annual thermal profile than Fairbanks Creek. Increasing our understanding of caddisfly flight periodicity in various types of habitats will greatly improve their efficacy as aquatic biological monitoring organisms.
Acknowledgments
I thank Chris Bowyer, Kelsey Brakel, Kiralyn Brakel, Erin Furmaga, Hannah (Russell) Goble, Connie (Brandin) Loruss, Bilyana (Petkova) McLeod, Bridget O'Leary, Angie Pytel, Sarah (Rogers) Salow, Logan Shoup, Peter Thistelton, and Lydia Wassink for assistance with sampling and rough-sorting the nearly 20,000 caddisfly specimens examined in this study. The valuable comments of two anonymous reviewers improved earlier versions of the manuscript. This is paper #20 of the G.H. Gordon BioStation Research Series.
Disclosure statement
No potential conflict of interest was reported by the author.
Additional information
Funding
Notes on contributors
David C. Houghton
David Houghton is a professor of biology at Hillsdale College and the director of the G.H. Gordon Biological Station. His research involves the biological diversity of aquatic organisms, especially caddisflies, and the effects of natural and anthropogenic impacts on their assemblages.
References
- Anderson DJ, Vondracek B. 1999. Insects as indicators of land use in three ecoregions in the Prairie Pothole region. Wetlands. 19:648–664.
- Bakker JD. 2008. Increasing the utility of indicator species analysis. J Appl Ecol. 45:1829–1835.
- Barbour MT, Gerritsen J, Snyder BD, Stribling JB. 1999. Rapid bioassessment protocols for use in streams and rivers: Periphyton, benthic macroinvertebrates, and fish. 2nd ed. EPA 841-B-99-002. Washington, DC: Office of Water, U.S. Environmental Protection Agency.
- Blinn DW, Ruiter DE. 2013. Tolerance values and effects of selected environmental determinants on caddisfly (Trichoptera) distribution in northwest and north central Washington, USA. West N Am Nat. 73:270–294.
- Boda P, Horváth G, Kriska G, Blahó M, Csabai Z. 2014. Phototaxis and polarotaxis hand in hand: night dispersal flight of aquatic insects distracted synergistically by light intensity and reflection polarization. Naturwisswenchaften. 101:385–395.
- Bode RW, Novak MA, Abele LA. 1991. Methods for rapid biological assessment of streams. Albany, NY: New York Department of Environmental Conservation.
- Brakel K, Wassink LR, Houghton DC. 2015. Nocturnal flight periodicity of the caddisflies (Trichoptera) in forest and meadow habitats of a first order Michigan stream. Great Lakes Entomol. 48:34–44.
- Cao Y, Hawkins CP. 2011. The comparability of bioassessments: a review of conceptual and methodological issues. J N Am Benthol Soc. 30:680–701.
- Carignan V, Villard MA. 2002. Selecting indicator species to monitor ecological integrity: a review. Environ Monit Assess. 78:45–61.
- Collier K, Smith B, Ballie B. 1997. Summer light-trap catches of adult Trichoptera in hill-country catchments of contrasting land use. New Zealand J Mar Freshw Res. 31:623–634.
- Cummins, KW. 1964. Factors limiting the microdistribution of larvae of the caddisflies Pycnopsyche lepida (Hagen) and Pycnopsyche guttifer (Walker) in a Michigan stream (Trichoptera: Limnephilidae). Ecol Monogr. 34:271–295.
- Dobrin M, Giberson DJ. 2003. Life history and production of mayflies, stoneflies, and caddisflies (Ephemeroptera, Plecoptera, and Trichoptera) in a spring-fed stream in Prince Edward Island, Canada: evidence for population asynchrony in spring habitats? Can J Zool. 81:1083–1095.
- Dohet A. 2002. Are caddisflies an ideal group for the assessment of water quality in streams? In: Mey W, editor. Proceedings of the 10th International Symposium on Trichoptera; 30 Jul–05 Aug; Potsdam, Germany. p. 507–520. Nova Suppl. Entomol., Keltern Germany.
- Dufrêne M, Legendre P. 1997. Species assemblages and indicator species: the need for a flexible asymmetrical approach. Ecol. Mon. 67:345–366.
- Hawkins CP, Norris RH, Gerritsen J, Hughes RM, Jackson SK, Johnson RK, Stevenson RJ. 2000. Evaluations of the use of landscape classifications for the prediction of freshwater biota: synthesis and recommendations. J North Am Benthol Soc. 19:541–556.
- Hilsenhoff WL. 1987. An improved biotic index of organic stream pollution. Great Lakes Entomol. 20:31–39.
- Houghton DC. 2004. Minnesota caddisfly biodiversity (Insecta: Trichoptera): delineation and characterization of regions. Environ Mon Assess. 95:153–181.
- Houghton DC. 2006. The ability of common water quality metrics to predict habitat disturbance with biomonitoring with adult caddisflies (Insectas: Trichoptera). J Freshw Ecol. 21:705–716.
- Houghton DC. 2007. The effects of landscape-level disturbance on the composition of caddisfly (Insecta: Trichoptera) trophic functional groups: evidence for ecosystem homogenization. Env Mon Assess. 135:253–264.
- Houghton DC. 2012. Biological diversity of Minnesota caddisflies. ZooKeys Special Issues. 189:1–389.
- Houghton DC. 2015a. Delineation and characterization of Michigan caddisfly biological diversity (Insecta: Trichoptera), and comparison with Minnesota. J Freshw Ecol. 30:525–542.
- Houghton DC. 2015b. A 5-year study of the adult flight periodicity of 27 caddisfly (Trichoptera) species in forest and meadow habitats of a first-order Lower Michigan (USA) Stream. Env Entomol. 44:1472–1487.
- Houghton DC, Berry EA, Gilchrist A, Thompson J, Nussbaum MA. 2011. Biological changes along the continuum of an agricultural stream: influence of a small terrestrial preserve and the use of adult caddisflies in biomonitoring. J Freshw Ecol. 26:381–397.
- Houghton DC, Brandin CM, Brakel KA. 2011. Analysis of the caddisflies (Trichoptera) of the Manistee river watershed, Michigan. Great Lakes Entomol. 44:1–15.
- Houghton DC, Wasson JL. 2013. Abrupt biological discontinuity in a small Michigan stream due to historical riparian canopy loss. J Freshw Ecol. 28:293–306.
- Huryn AD, Wallace JB. 2000. Life history and production of aquatic insects. Ann Rev Entomol. 45:83–110.
- Kimura G, Hirabayashi K, Hanazato T. 2006. Abundance and distribution of adult caddisflies (Trichoptera) caught by light traps in Lake Suwa. In: Nakano S, Hwang S-J, Tanida K, Hirotani H, editors. Proceedings of the Second Japan-Korea Joint Symposium on Limnology. Osaka: The Japanese Society of Limnology. p. 1–10.
- Kimura G, Inoue E, Hirabayashi, K. 2008. Seasonal abundance of adult caddisfly (Trichoptera) in the middle reaches of the Shinano River in Central Japan. In: Robinson WH, Bajomi D, editors. Proceedings of the 6th International Conference on Urban Pests. Budapest: OOK-Press Korlátolt felelősségű társaság. p 259–266.
- Krueger CC, Cook EF. 1984. Life cycles and drift of Trichoptera from a woodland stream in Minnesota. Can J Zool. 62:1479–1484.
- Li JL, Johnson SL, Sobota JB. 2011. Three responses to small changes in stream temperature by autumn-emerging aquatic insects. J NA Benthol Soc. 30:474–484.
- McCune B, Medford MJ. 2006. PC-ORD. Multivariate analysis of ecological data. Version 5. Gleneden Beach, OR: MjM Software.
- Merritt RW, Cummins KW, Berg MB. 2008. An introduction to the aquatic insects of North America. 4th ed. Dubuque, IA: Kendall/Hunt.
- Moulton SR, Stewart KW. 1996. Caddisflies (Trichoptera) of the interior highlands of North America. Mem Amer Entomol Inst. 56:1–313.
- Petersen I, Winterbottom JH, Orton S, Friberg N, Hildrew AG, Spiers DC, Gurney WSC. 1999. Emergence and lateral dispersal of adult Plecoptera and Trichoptera from Broadstone Stream, U.K. Freshw Biol. 42:401–416.
- Singh MP, Smith SM, Harrison AD. 1984. Emergence of some caddisflies (Trichoptera) from a wooded stream in southern Ontario. Hydrobiologia. 112:223–232.
- Sommerhäuser M, Koch P, Robert B, Schumacher H. 1999. Caddisflies as indicators for the classification of riparian systems along lowland streams. In: Malicky H, Chantaramongkol P, editors. Proceedings of the 9th International Symposium on Trichoptera; Jan 5–10; Chiang Mai, Thailand: Faculty of Science, Chiang Mai University. p. 337–348.
- Swegman BG, Walker W, Sykora JL. 1981. The adult Trichoptera of Linesville Creek, crawford county, Pennsylvania with notes on their alight activity. Trans Am Entomol Soc. 107:125–147.
- Trudgill DL, Honek A, Li D, Van Straalen NM. 2005. Thermal time – concepts and utility. Ann Appl Biol. 146:1–14.
- Waringer JA. 1989. The abundance and temporal distribution of caddisflies (Insecta: Trichoptera) caught by light traps on the Austrian Danube from 1986 to 1987. Freshw Biol. 21:387–399.
- Waringer JA. 1991. Phenology and the influence of meteorological parameters on the catching success of light-trapping for Trichoptera. Freshw Biol. 25:307–319.
- Waringer JA. 2003. Light-trapping of caddisflies at the Thaya (Lower Austria), a river influenced by pulsating hypolimnetic water release. Inter Rev Hydrobiol. 88:139–153.
- Williams DD, Hogg ID. 1988. Ecology and production of invertebrates in a Canadian coldwater spring-springbrook system. Ecography. 11:41–54.
- Wright DR, Pytel AJ, Houghton DC. 2013. Nocturnal flight periodicity of the caddisflies (Insecta: Trichoptera) in a large Michigan river. J Freshw Ecol. 28:463–476.