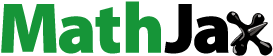
Abstract
The effects of food quality and food quantity on crustacean zooplankton’s feeding rate have been analyzed in the present study. In two experiments, we studied the clearance rates (CRs) of Daphnia pulex on different algal diets (Scenedesmus obliquus, nontoxic Microcystis sp. PCC7806 and Anabeana flos-aquae) varying in carbon to phosphorus (C:P) ratios and quantities (concentrations). In order to investigate the effects of different algal diets on the feeding of cladocerans, the mixture of S. obliquus, Microcystis sp. PCC7806 and A. flos-aquae was also fed to D. pulex during a 72-h period. Our results showed: (1) D. pulex had different clearance rates from the three mono-specific P-rich algal diets, while no significant difference among clearance rates was observed among the three mono-specific P-poor algal diets; (2) D. pulex increased its clearance rates when it fed on mono-specific P-poor S. obliquus and A. flos-aquae, while a P-poor mixture of M. aeruginosa and A. flos-aquae reduced the clearance rate; (3) A U-shaped quadratic function provided a good fit to the clearance rate and C:P ratios because the clearance rate of D. pulex in both low and high C:P categories were significantly higher than in the moderate C:P category (C:P value = 219). Our results demonstrated that filaments and C:P ratios of algae strongly influence the feeding of crustacean zooplankton. ‘Compensatory feeding’ occurred in the mono-specific, P-poor algal diets but not in the mixed diets. Moreover, the clearance rate of zooplankton was the lowest in the moderate P stoichiometric category. Our experiments suggest that the morphology and C:P ratios of algal diets have important effects on the feeding of Daphnia, and the P stoichiometric characteristics in mixed diets may play an important role in the feeding of large-sized cladocerans.
Introduction
The feeding of crustacean zooplankton species on phytoplankton is an important index for evaluating the biomass of phytoplankton and the ‘top-down’ force of the aquatic food chain (Sahuquillo et al. Citation2007; Rollwagen-Bollens et al. Citation2013). In eutrophic lakes and rivers cyanobacteria may dominate the aquatic ecosystem because they have the ability to resist ingestion by crustacean zooplankton. In general, cyanobacteria may directly decrease the fitness of zooplankton through (i) the toxicity of their secondary metabolites, such as microcystins (MCs), (ii) mechanical interference with food collection, referred to here as poor manageability, and (iii) their low nutritional value (i.e. they lack or contain insufficient amounts of essential components like polyunsaturated fatty acids, sterols and other as yet unidentified substances (Kirk and Gilbert Citation1992; Sarnelle and Wilson Citation2005; Bednarska et al. Citation2014; Fabre et al. Citation2017). Filaments of cyanobacteria usually result in a feeding inhibition of daphnids and the proportion of filamentous cyanobacteria relative to suitable food may be a key factor in the outcome of feeding performance of Daphnia (Gliwicz and Lampert Citation1990; Panosso and Lürling Citation2010).
Meanwhile, some studies suggested that toxic cyanobacteria and non-toxic cyanobacteria also lead to a feeding inhibition of Daphnia (Costa et al. Citation2013; Zhu et al. Citation2013), although feeding inhibition in the presence of cyanobacteria could be attributable to the defense mechanisms of daphnids rather than a survival strategy of cyanobacteria (Jiang et al. Citation2013; Zhu et al. Citation2013). Gall et al. (Citation2017) suggested that in a low temperature and low physiological treatment, the lack of food had a more important effect on the fitness of Daphnia than food quality. An increase in the feeding by herbivores, caused by the low nutrient content of food, is usually called ‘compensatory feeding’ (Darchambeau et al. Citation2003). However, it is not yet clear how food quantity and food quality lead to compensatory feeding of Daphnia (Elser et al. Citation2016; Wagner et al. Citation2017). The consequences of these shifts of feeding rate were major changes in trophic conversion efficiency and the internal state of homeostasis. So, more insight is needed on the negative effects of cyanobacteria on zooplankton feeding and it is necessary to analyze the effects of food quantity and food quality on the feeding of Daphnia.
In most freshwater systems, phosphorus (P) is not only the limiting nutrient for phytoplankton growth (Sterner and Elser Citation2002; Prater et al. Citation2017), but also is a limiting factor for Daphnia populations in many situations (DeMott Citation2003; Hessen et al. Citation2013). The concentration of P can have an important effect on the species and community structure of crustacean zooplankton through the food chain (Sterner and Elser Citation2002). The effects of high nitrogen:phosphorus (N:P) and carbon:phosphorus (C:P) food on Daphnia populations are well established due to extensive prior work (Sterner and Elser Citation2002; Hessen et al. Citation2013). A large number of studies suggest that a high N:P ratio in algal diets is usually considered to limit growth of invertebrates and a high C:P ratio in algal diets is usually considered as poor food quality (Lukas et al. Citation2011; Hood and Sterner Citation2014). When the C:P ratio in algal diets exceeds a threshold (element proportion threshold), the growth and reproduction of zooplankton will decrease with consequences for population dynamics, community structure, the trophic cascade and evolution (Urabe et al. Citation2002; Meng et al. Citation2014; Elser et al. Citation2016). Plath and Boersma (Citation2001) argued that Daphnia adjust their feeding rate to food P content, and Suzuki-Ohno et al. (Citation2012) reported that the feeding rate of Daphnia would decrease as the content of phosphorus in algal diets decreased. Elser et al. (Citation2016) showed that the high C:P ratio will increase the respiratory rate of Daphnia, and respiration rates were significantly higher in both low and high C:P food treatments. A U-shaped quadratic function provided a good fit to the feeding data, which is consistent with the stoichiometric knife-edge hypothesis supported by Plath and Boersma (Citation2001). However, previous studies usually involved physiologically uniform cells of a mono-specific species of green algae. Whether or not similar responses were observed for zooplankton processing mixtures of various phytoplankton species requires further investigation. Also, whether the compensatory feeding by Daphnia would occur in the mixture of algal diets requires further testing. The clearance rates of Daphnia were investigated as a function of stoichiometric imbalance, the study of phosphorus stoichiometric characteristics and feeding traits would improve our understanding of energy intake and the elemental composition of herbivores.
In the present study, three algae species with different P content were selected for the feeding experiments. These algae species were cultured in P-sufficient and P-deficient media. A large crustacean zooplankter (Daphnia pulex) was also used in the feeding experiment. The effects of different algal species, poor manageability and C:P ratios on the clearance rates of Daphnia were analyzed in the feeding experiments. The phosphorus content of algal diets and clearance rates of D. pulex was also assessed in different algal diets. The relationship between C:P ratios and Daphnia’s clearance rates was also analyzed. Our experiments elucidate the imbalance mechanism of the phosphorus biogeochemical cycle through the low nutritional value of algae.
Methods
Experimental organisms
Single-celled green algae (Scenedesmus obliquus, biovolume 50.00 µm3.ind−1, FACHB-417), non-toxic cyanobacterium (Microcystis sp. PCC7806, biovolume 31.28 µm3.ind−1, FACHB-915) and non-toxic filamentous cyanobacterium (Anabeana flos-aquae, biovolume 64.53 µm3.ind−1, filament length ranging from 225 to 427 µm, FACHB-1496; strain originating from the Institute of Hydrobiology, Chinese Academy of Science, China) were used as food for Daphnia in all experiments. They were incubated in the exponential growth phase with frequent transfers into BG-11 medium (Rippka et al. Citation1979). All algae were cultured on P-sufficient (230 μmol P L−1) or P-deficient (10 μmol P L−1) BG11 medium. The cultures were grown at 25 °C under fluorescent lights at 50 μmol photons m−2 s−1, on a 12:12 h light:dark cycle (). No MCs were detected in the non-toxic strain of S. obliquus, Microcystis sp. PCC7806 and A. flos-aquae. Clones of the large-bodied cladoceran D. pulex (Adults, 2.17 ± 0.12 mm), which were isolated from Chaohu lake located at Hefei, Anhui Province, China. Cladocerans were provided with nutritious S. obliquus daily at a concentration of 5 × 105cells mL−1. Animals were incubated in tanks containing 5 L of M7 medium (Samel et al. Citation1999; Guo and Xie Citation2006) in the laboratory at least for one month prior to the experiments. The D. pulex individuals were taken from a non-monoclonal laboratory culture.
Table 1. Mean (standard error) food quality expressed in molar N:P, C:N and C:P ratios for P- rich and P-poor algae treatments used in the two experiments.
Mono-specific algal diets
Newly born (8–24 h), lively, healthy D. pulex (average body length of 0.735 ± 0.032 mm) were selected for the feeding experiments on mono-specific algal diets. Ten D. pulex individuals were put into a plastic vessel with 10 mL M7 medium to ensure the consistency of the offspring with D. pulex, for the same maternal experiment. The culture temperature was 25 °C and the plastic vessel was covered by a black plastic bag to provide shade. The P-rich (C:P 80) and P-poor (C:P 388) S. obliquus, the P-rich (C:P 149) and P-poor (C:P 175) Microcystis sp. PCC7806 and the P-rich (C:P 17) and P-poor (C:P 49) A. flos-aquae diets were feed to D. pulex, respectively. The initial concentration of algal bait was 4 × 104, 1 × 105, 2 × 105, 4 × 105, 1 × 106, 2 × 106 cells mL−1. For each treatment, 30 neonates were distributed in three replicated vessels (10 mL) and the feeding experiments lasted for 72h.
Mixed diets experiments
Different mixtures of algae were fed to D. pulex the same as the mono-specific algae experiment: Ten D. pulex larvae (<24 h) were put into the plastic vessels with M7 medium, the P-rich (C:P 115) mixture of S. and Microcystis sp. PCC7806, the P-rich (C:P 49) mixture of S. obliquus and A. flos-aquae, the P-rich (C:P 83) mixture of Microcystis sp. PCC7806 and A. flos-aquae were feed to D. pulex, respectively. The P-poor (C:P 282) mixture of S. obliquus and Microcystis sp. PCC7806, the P-poor (C:P 218) mixture of S. obliquus and A. flos-aquae, the P-poor (C:P 112) mixture of Microcystis sp. PCC7806 and A. flos-aquae were also feed to D. pulex. The percentage of each algae species in mixture diets was 50%. The initial concentration of algal diets was 4 × 104, 1 × 105, 2 × 105, 4 × 105, 1 × 106, 2 × 106 cells mL−1, The algal density was estimated using a cell counter and analyzer system at 4, 8, 12, 24, 48 and 72 h.
Chemical analysis
For the C and N analysis of algal samples, the suspension was filtered through pre-combusted (at 500 °C) glass fiber (GF/F, Whatman) filters and dried at 60 °C for >24 h and exposed to concentrated HCl fumes for carbonate removal. The dried filters were digested in a K2S2O8 solution at 125 °C for 30 min. It has been documented that burning reduces the variability and makes P more soluble. Blank filters were carried through the same procedure. Standard curves were prepared for each batch of samples based on a stock phosphate solution (Bukovinszky et al. Citation2012). C- and N-content in algae and Daphnia was determined using a FLASH 2000 organic elemental analyzer (Brechbueler Inc., Interscience B.V., Breda, the Netherlands). The content of P is determined by the conventional ammonium molybdate spectrophotometric method.
Data analysis
The total and respective clearance rates (CR) of the animals on all the algae or the mixture of algae were calculated using the following equation (Peters Citation1984):
Among them, CR is the clearance rate (ml−1 ind−1· h−1); V is the testing water volume (mL); N is the total number of the experimental animals (individual); Ct is the concentration at time t in the controls (cells mL−1); Ctf is the phytoplankton concentration at time t in the treatments (cells mL−1); t is the incubation period (h). For each sampling time, differences of clearance rates among the treatments were analyzed via one-way analyses of variance (ANOVA) followed by a post hoc multiple comparisons (Dunnett test). Results are presented as mean SD. Differences were considered at the significance level of 0.05. The analysis was undertaken using SPSS for Windows Release 19.0.
Results
The feeding effects on mono-specific algal diets
In the first experiment, D. pulex showed considerable variation in the clearance rates to three algal diets overall total time. Among the mono-specific algal diets experiments: (1) There was a significant difference between the clearance rates among the three P-rich algal diets. Non-toxic Microcystis sp. PCC7806 had the highest clearance rate, followed by S. obliquus and A. flos-aquae (F2,15 = 11.28, p < 0.05). D. pulex showed no difference in clearance rates in the three P-poor algal diets (F2,15 = 0.20, p > 0.05; ). (2) D. pulex had a higher clearance rate when it was fed P-poor S. obliquus compared with P-rich S. obliquus (F1,10 = 5.01, p < 0.05). A higher clearance rate of D. pulex was also observed in P-poor A. flos-aquae diets compared with P-rich A. flos-aquae diets (F1,10 = 6.62, p < 0.05); there was no significant difference among D. pulex’s clearance rates when it was fed P-poor Microcystis sp. PCC7806 compared with P-rich Microcystis sp. PCC7806 (F1,10 = 0.29, p > 0.05) ( and , ). (3) There is a significant effect of food quantity on the clearance rate of D. pulex: the clearance rate of D. pulex was the highest in the moderate food quantity (1 × 106 cells mL−1) in the P-rich S. obliquus treatments (C:P ratio = 80.26, F5,30 = 15.67, p < 0.001) and the P-poor S. obliquus treatments (C:P ratio = 349.75, F5,30 = 480.62, p < 0.001). The clearance rates of D. pulex increased as the concentration of food increased significantly in the P-rich Microcystis sp. PCC7806 treatments (C:P ratio = 148.97, F5,30 = 53.87, p < 0.001) and the P-poor Microcystis sp. PCC7806 treatments (C:P ratio = 174.62, F5,30 = 1311.51, p < 0.001). The clearance rate of D. pulex was the lowest in the moderate food quantity (1 × 106 cells mL−1) in the P-rich A. flos-aquae treatments (C:P ratio = 17.22, F5,30 = 15.67, p < 0.001) and in the P-poor Anabaena treatments (C:P ratio = 48.75, F5,30=54.96, p < 0.001) ().
Figure 1. Specific clearance rates of D. pulex on mono-specific (A–B) and mixed (C–D) algal diets. S + M: S. obliquus and Microcystis sp. PCC7806 treatment. S + A: S. obliquus and A. flos-aquae treatment. M + A: Microcystis sp. PCC7806 and A. flos-aquae treatment.
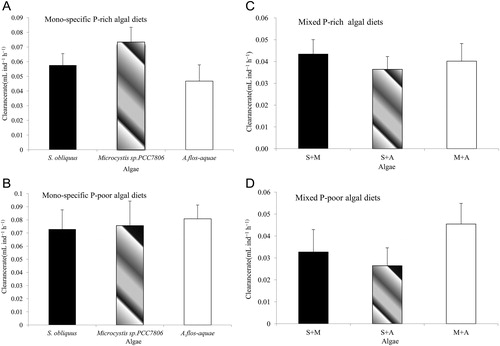
Figure 2. Effects of the P-rich and P-poor S. obliquus, Microcystis sp. PCC7806, A. flos-aquae (A–C); mixture of S. obliquus and Microcystis sp. PCC7806 (S + M), mixture of S. obliquus and A. flos-aquae (S + A), mixture of Microcystis sp. PCC7806 and Microcystis sp. PCC7806 (M + A) (D–F) on the CRs of D. pulex over time (4, 8, 12, 24, 48 and 72 h).
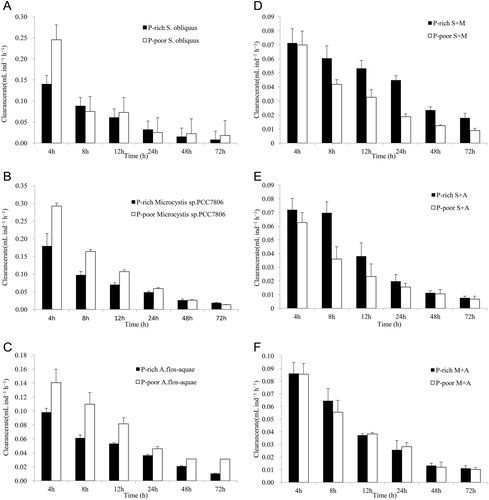
Figure 3. The effect of food concentration on CRs of D. pulex, cultured on P-poor (C:P 388, open symbols) and P-rich (C:P 80, closed symbols) S. obliquus, P-poor (C:P 175, open symbols) and P-rich (C:P 149, closed symbols) Microcystis sp. PCC 7806, P-poor (C:P 49, open symbols) and P-rich (C:P 17, closed symbols) A. flos-aquae (A–C); P-poor (C:P 282, open symbols) and P-rich (C:P 115, closed symbols) mixture of Microcystis sp. PCC 7806 and S. obliquus (S + M), P-poor (C:P 219, open symbols) and P-rich (C:P 49, closed symbols) mixture of S. obliquus and A. flos-aquae (S + A), P-poor (C:P 112, open symbols) and P-rich (C:P 83, closed symbols) mixture of Microcystis sp. PCC 7806 and A. flos-aquae (M + A) diets (D–F) at six food concentrations (4, 10, 20, 40, 100 and 200 × 104 cells mL−1).
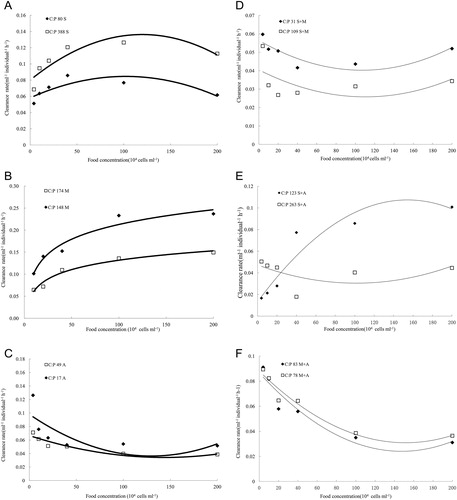
Table 2. Effect of the P-rich mixture diets on the total CRs of D. pulex.
Table 3. Effect of the P-poor mixture diets on the total CRs of D. pulex.
Feeding effects on mixed diets
In the second experiment, there was a significant difference in the feeding rates of D. pulex fed with different mixture of algal diets, and Daphnia had a lower clearance rate on mixture diets compared with mono-specific species of algae. Among the mixed diets experiments: (1) D. pulex had a lower clearance rate in mixture of algal diets than mono-specific algal diets, while there was no significant difference in clearance rate among the three mixtures of algal diets (F2,15 = 0.825, p >0.05; ). It showed that D. pulex had different clearance rates when it fed with P-poor mixture diets compared with P-rich mixture diets (F2,15 = 6.49, p < 0.05) (). (2) D. pulex had a lower clearance rate when it fed with a P-poor mixture than a P-rich mixture of A. flos-aquae and Microcystis sp. PCC7806 diet (F1,10 = 8.53, p < 0.05); while no difference of clearance rates was observed in the mixture of S. obliquus and Microcystis sp. PCC7806 (F1,10 = 0.584, p > 0.05) or mixture of S. obliquus and A. flos-aquae (F1,10 = 3.43, p > 0.05) ( and , ). (3) The clearance rates of D. pulex were significantly affected by food quantity. The clearance rate of D. pulex was the highest in the moderate food quantity (1 × 106 cells/ml) when it fed with the P-rich (F5,30 = 54.96, p < 0.001) and P-poor (F5,30 = 14.45, p < 0.001) mixture of Microcystis sp. PCC7806 and S. obliquus. The clearance rate of D. pulex was the highest in the moderate food quantity (1 × 106 cells mL−1) when it was fed the P-rich mixture of S. obliquus and A. flos-aquae (F5,30 = 8.52, p < 0.001), while it was the lowest in the moderate food quantity (1 × 106 cells mL−1) when it fed with the P-poor mixture of S. obliquus and A. flos-aquae (F5,30 = 22.13, p < 0.001). The clearance rate of D. pulex increased significantly with increasing food concentration when it fed on the P-rich (C:P ratio = 148.97, F5,25 = 117.05, p < 0.001) and P-poor (C:P ratio = 174.62, F5,30 = 9.31, p < 0.001) mixture of Anabaena and Microcystis sp. PCC7806 ().
The effect of P content on the feeding rate of D. pulex
During the experiment, the C:N ratio of algal diets was about 5.99–9.52, the C:N ratio of algal diets had no significant effect on clearance rate of D. pulex (F1,30 = 1.67, p > 0.05); the N:P ratio of algae was about 2.89–43.46, the N:P ratios had no significant impact on the feeding rate of D. pulex (F1,30 = 5.67, p > 0.05); the C:P ratio of algae was about 17.22–388.40, feeding rate responded significantly (p < 0.05) to food C:P ratio in D. pulex, with somewhat elevated feeding rate in both the lowest C:P and the highest C:P ratio treatment (, ). Our results suggested: (1) There was no significant difference of C:N ratio in all algal diets, and significant difference was found in the N:P ratio of algal diets and significant difference was found in C:P ratio of algal diets (). When algae were cultured on P-sufficient (230 μmol P L−1) BG11 medium, filamentous A. flos-aquae (17.22 ± 0.36) had a lower C:P ratio than single-celled S. obliquus (80.26 ± 3.42) and Microcystis sp. PCC7806 (148.97 ± 5.64). The C:N and N:P ratio of algal diets had no significant effect on the clearance rate of D. pulex, while C:P ratio of algal diets had a significant effect on the clearance rate of D. pulex. (2) The present study suggested that a U-shaped quadratic function provided a good fit to the clearance rate and C:P ratios, and the clearance rate of D. pulex ∼2.9 and 3.0 times higher in the lowest (17:1) and highest (388:1) C:P ratio treatments, respectively, relative to feeding rate with food of intermediate C:P ratio (219:1) (). It showed that not only P-poor (high C:P ratio) food, but also P-rich (low C:P ratio) foods will have a positive impact on the feeding of herbivorous consumers, D. pulex may be a positive response to this effect on the feeding efficiency of P-poor food and P-rich food.
Discussion
Feeding experiments on mono-specific algal diets
In aquatic systems, the feeding of crustacean zooplankton is affected by many factors such as light, temperature, pH and so on (Elser et al. Citation2016). The feeding behavior of cladocerans is strongly influenced by the quantity and quality of food to which the animals have been acclimated (Zhu et al. Citation2013; Rangel et al. Citation2016). In the present study, there is a significant difference of D. pulex’s clearance rate in different P-rich algal diets (good quality of food), while there was no significant difference of D. pulex’s clearance rate in different P-poor algal diets (bad quality of food). According to Brooks and Dodson’s (Citation1965) seminal Size Efficiency Hypothesis, feeding responses to algal diets with different algal size are variable in Daphnia. As well known, most cladocerans are relatively nonselective filter feeders (Zhu et al. Citation2013) and the size of algae play an important role in the clearance rates of water fleas which result in the low clearance rates on filamentous A. flos-aquae. While in P-poor algal diets (bad quality of food), the inferior quality of algal diets would reduce the effect of Size Efficiency, which would result no significant difference in the clearance rates of Daphnia (Suzuki-Ohno et al. Citation2012). A ‘compensatory feeding’ was observed in the mono-specific Scenedesmus and Anabaena diets, Daphnia increased their feeding effort when fed mono-specific algae with low P content (Plath and Boersma Citation2001). While the ‘compensatory feeding’ was not observed in the Microcystis diet, which may attribute to the low P content and some inhibitory compounds of non-toxic Microcystis sp. PCC7806 (Darchambeau et al. Citation2003; Zhu et al. Citation2013; Freitas et al. Citation2014). From these results, we can infer that the feeding behavior of cladoceran is strongly influenced by the quantity and quality of food, especially for filamentous cyanobacteria, when its concentrations beyond the above-mentioned critical level, feeding inhibition by filamentous cyanobacteria is most probably due to entirely mechanical interference (Sahuquillo et al. Citation2007; Panosso and Lürling Citation2010; Sikora and Dawidowicz Citation2017).
Our results suggested that compensatory feeding varies with changes in diet, including food abundance, digestibility and the severity of nutritional element shortages within food. The inferior quality and the concentration of filaments both strongly influence the compensatory feeding. A good quality of algal diets may compensate the effects of filaments while a bad quality of algal diets not. It may indicate that the predation of Daphnia on cyanobacteria occurred with a low concentration of cyanobacterial filaments and a high food quality (P-rich algal diets). A high density of filamentous cyanobacteria would result in a feeding inhibition of large-sized cladocerans, but ‘compensatory feeding’ in mono-specific algal diets would increase the feeding rate of Daphnia.
The feeding experiments on mixture diets
In the field, natural seston communities are usually composed of various phytoplankton species and zooplankton usually grazed on mixture diets. Zhu et al. (Citation2013) reported that the mixture of non-toxic M. aeruginosa and S. obliquus would decrease the ingestion rate of D. carinata, and the feeding rates of D. carinata declined when it fed with a mixture diet. In the present study, the lower clearance rates on mixture diets may exhibit that the feeding rate of large-sized cladocerans was usually overestimated as the feeding rate was usually evaluated by the data of mono-specific algal diets (Rollwagen-Bollens et al. Citation2013). From the results of feeding experiments, significant differences were observed in the clearance rates of D. pulex on three P-poor mixture diets, while no difference was observed on the P-rich mixtures diets. Feeding activity of D. pulex decreased with increasing in C:P ratio of the mixture diet, especially in the mixture of non-toxic Microcystis sp. PCC7806 and Anabaena treatment. Filaments and inhibitory compounds from cyanobacteria were both strongly influence the clearance rate, so the P-poor mixture of Microcystis sp. PCC7806 and Anabaena reduce D. pulex’s CRs significantly (Boersma and Stelzer Citation2000; Gulati et al. Citation2001; Plath and Boersma Citation2001). Contrast with mono-specific algae, we did not find a significant increase in CRs when Daphnia were fed mixed algae. ‘Compensatory feeding’ in the P-poor mixture diets was not observed as suggested by Urabe et al. (Citation2018). It may exhibit Daphnids may be easily satiated when fed mono-specific food, but their appetite may be stimulated when a variety of food is supplied (Urabe et al. Citation2018). Many researchers observed that high food C:P ratios would negatively influence the feeding rate and growth rate of Daphnia (Van Donk et al. Citation1997; He and Wang Citation2007; He and Wang Citation2008; Currier and Elser Citation2017). The behaviorally mediated changes in feeding activity may serve as mechanisms adjusting the C:P ratio of ingested food and thus offer a possible mechanistic explanation for homeostasis in Daphnia (Van Donk et al. Citation1997). The P stoichiometric characteristics in mixture diets may play an important role in the feeding of large-sized cladocerans. Moreover, filaments and colonies of cyanobacteria were usually observed in field and were expected to have a greater inhibitory effect on clearance rates than single-celled cyanobacteria (Wilson et al. Citation2006). The effects of filaments and colony on the feeding processes of zooplankton should not be ignored in eutrophic lakes.
The effect of phosphorus content on the feeding rate
D. pulex was the most commonly used species in the researches of the relationship between cyanobacteria and cladocerans, earlier studies mainly focus on the effect of C:P ratios in algal diets on the growth rate of crustacean zooplankton(Wilson et al. Citation2006; Jiang et al. Citation2013). In the present study, all algae were cultured on P-sufficient (230 μmol P L−1) and P-deficient (10 μmol P L−1) BG11 medium, respectively. The concentration of phosphorus in the medium is much higher than that in field (about 4 umol P L−1 in Chaohu lake). The C:P ratios in algal diets range from 17.22 to 349.75, and in large lakes seston C:P ratios observations <120 (atomic) contributed 31% overall to compiled data. Plath and Boersma (Citation2001) achieved food C:P ratio <40 and Elser et al. (Citation2016) achieved food C:P ratios were ∼60. In the present study, a lower C:P ratio was found in A. flos-aquae (C:P 17) than S. obliquus (C:P 80). It may suggest that the P content of filamentous cyanobacteria is higher than green algae, while the low clearance rate on filamentous cyanobacteria would reduce the assimilation of P (Sikora and Dawidowicz Citation2017). Our feeding experiments also supported the existence of a ‘stoichiometric knife-edge’ for D. pulex. A U-shaped quadratic function provided a good fit to the clearance rate and C:P ratios. The maximum feeding rate in our experiments (∼0.5–0.6) is similar to those reported elsewhere (Sikora and Dawidowicz Citation2017), and the clearance rates of D. pulex in both low and high C:P categories were significantly higher than in the moderate C:P category(C:P value = 219). It was also found that the respiration rates were lowest for Daphnia on foods in the moderate C:P category, elevated respiration rates for consuming high C:P food were generally interpreted as a means of disposing of ‘excess C’ (Darchambeau et al. Citation2003; Hessen et al. Citation2013; Elser et al. Citation2016). Currier and Elser (Citation2017) reported that growth rate reductions for Daphnia were strongly in response to high C:P food, most likely as a result of decreased feeding rate. The reduction of the feeding rate may attribute to the preservation of energy (He and Wang Citation2008) or to convert the body P content into the SP (somatic phosphorus) (Sterner and Elser Citation2002). Acharya et al. (Citation2004) showed that the P to dry weight ratio of Daphnia fed algae with an atomic C:P ratio of 995 was lower than that of Daphnia fed algae with a C:P ratio of 104. Although the variation in the body P content of Daphnia is much smaller than in algal species, different C:P food ratios would result in variations of the SP and fitness among Daphnia (Sterner and Elser Citation2002). The variations of the SP and fitness among Daphnia may highly influence the growth and reproduction of Daphnia. For the high correlation of feeding rate and growth rate of Daphnia, the dietary diversity and quality is indeed a concern in nature. Moreover, the influence of MCs and some unidentified substances on the relationship between different C:P foods and the clearance rates of Daphnia were also needed to research in future.
Notes on contributors
Nichun Guo, Male, PH.D, Associate Professor, Major in freshwater ecology.
Mingyuan Li, Female, undergraduate, Major in ecology.
Haotong Tian, Male, undergraduate, Major in ecology.
Youhua Ma, Male, PH.D, Professor, Major in Agricultural Resources and Environment.
Disclosure statement
No potential conflict of interest was reported by the authors.
Additional information
Funding
References
- Acharya K, Kyle M, Elser JJ. 2004. Biological stoichiometry of Daphnia growth: an ecophysiological test of the growth rate hypothesis. Limnol Oceanogr. 49(3):656–665.
- Bednarska A, Pietrzak B, Pijanowska J. 2014. Effect of poor manageability and low nutritional value of cyanobacteria on Daphnia magna life history performance. J Plankton Res. 36(3):838–847.
- Boersma M, Stelzer CP. 2000. Response of a zooplankton community to the addition of unsaturated fatty acids: an enclosure study. Freshwater Biol. 45(2):179–188.
- Brooks JL, Dodson SI. 1965. Predation, body size, and composition of plankton. Science. 150(3692):28–35.
- Bukovinszky T, Verschoor AM, Helmsing NR, Bezemer TM, Bakker ES, Vos M, Domis LNDS. 2012. The good, the bad and the plenty: interactive effects of food quality and quantity on the growth of different Daphnia species. PLoS One. 7(9):1–8.
- Costa SMD, Ferrão-Filho AS, Azevedo SMFO. 2013. Effects of saxitoxin- and non-saxitosin-producing strains of the cyanobacterium Cylindrospermopsis raciborskii on the fitness of temperate and tropical cladocerans. Harmful Algae. 28:55–63.
- Currier CM, Elser JJ. 2017. Beyond monoculture stoichiometry studies: assessing growth, respiration, and feeding responses of three Daphnia species to P-enriched, low C:P lake seston. Inland Waters. 7(3):348–357.
- Darchambeau F, Faerøvig PJ, Hessen DO. 2003. How Daphnia copes with excess carbon in its food. Oecologia. 136(3):336–346.
- DeMott WR. 2003. Implications of element deficits for zooplankton growth. Hydrobiologia. 491(1–3):177–184.
- Elser JJ, Kyle M, Learned J, McCrackin ML, Peace A, Steger L. 2016. Life on the stoichiometric knife-edge: effects of high and low food C:P ratio on growth, feeding, and respiration in three Daphnia species. Inland Waters. 6(2):36–146.
- Fabre A, Lacerot G, Rodriguez R, Carolina DPM, Soares S, Magalhães VFD, Bonilla S. 2017. South American PSP toxin-producing Cylindrospermopsis raciborskii (Cyanobacteria) decreases clearance rates of cladocerans more than copepods. Hydrobiologia. 785(1):61–69.
- Freitas EC, Pinheiro C, Rocha O, Loureiro S. 2014. Can mixtures of cyanotoxins represent a risk to the zooplankton? The case study of Daphnia magna Straus exposed to hepatotoxic and neurotoxic cyanobacterial extracts. Harmful Algae. 31:143–152.
- Gall A, Kainz MJ, Rasconi S. 2017. Daphnia magna fitness during low food supply under different water temperature and brownification scenarios. J Limnol. 76(1):161–169.
- Gliwicz ZM, Lampert W. 1990. Food thresholds in Daphnia species in the absence and presence of blue-green filaments. Ecology. 71(2):691–702.
- Gulati RD, Bronkhorst M, Donk EV. 2001. Feeding in Daphnia galeata on Oscillatoria limnetica and on detritus derived from it. J Plankton Res. 23(7):705–718.
- Guo NC, Xie P. 2006. Development of tolerance against toxic Microcystis aeruginosa in three cladocerans and the ecological implications. Environ Pollut. 143(3):513–518.
- He XJ, Wang WX. 2007. Kinetics of phosphorus in Daphnia at different food concentrations and carbon: phosphorus ratios. Limnol Oceanogr. 52(1):395–406.
- He XJ, Wang WX. 2008. Stoichiometric regulation of carbon and phosphorus in P deficient Daphnia magna. Limnol Oceanogr. 53(1):244–254.
- Hessen DO, Elser JJ, Sterner RW, Urabe J. 2013. Ecological stoichiometry-an elementary approach using basic principles. Limnol Oceanogr. 58(6):2219–2236.
- Hood JM, Sterner RW. 2014. Carbon and phosphorus linkages in Daphnia growth are determined by growth rate, not species or diet. Funct Ecol. 28(5):1156–1165.
- Jiang XD, Zhang LH, Liang HS, Li QM, Zhao YL, Chen LQ, Yang W, Zhao SY. 2013. Resistance variation within a Daphnia pulex population against toxic cyanobacteria. J Plankton Res. 35(5):1177–1181.
- Kirk KL, Gilbert JJ. 1992. Variation in herbivore response to chemical defenses: zooplankton foraging on toxic cyanobacteria. Ecology. 73(6):2208–2217.
- Lukas M, Sperfeld E, Wacker A. 2011. Growth Rate Hypothesis does not apply across colimiting treatments: cholesterol limitation affects phosphorus homoeostasis of an aquatic herbivore. Funct Ecol. 25(6):1206–1214.
- Meng M, Deng DG, Zhang XL, Ge Q, Zhang K. 2014. The influence of phosphorus concentration on the population dynamics and resting egg formation of two cladocerans. J Freshwater Ecol. 3:38–396.
- Panosso R, Lürling M. 2010. Daphnia magna feeding on Cylindrospermopsis raciborskii: the role of food composition, filament length and body size. J Plankton Res. 32(10):1393–1404.
- Peters RH. 1984. Methods for the study of feeding, grazing and assimilation by zooplankton. In: Downing JA, Rigler FH, editors. A manual on methods for the assessment of secondary productivity in fresh waters: IBP Handbook 17, 2nd ed. Blackwell: Oxford, p. 336–412.
- Plath K, Boersma M. 2001. Mineral limitation of zooplankton: stoichiometric constraints and optimal foraging. Ecology. 82:1260–1269.
- Prater C, Wagner ND, Frost PC. 2017. Interactive effects of genotype and food quality on consumer growth rate and elemental content. Ecology. 98:1399–1408.
- Rangel LM, Ger KA, Silva LHS, Soares MCS, Faassen EJ, Lürling M. 2016. Toxicity overrides morphology on Cylindrospermopsis raciborskii grazing resistance to the calanoid copepod Eudiaptomus gracilis. Microb Ecol. 71(4):835–844.
- Rippka RJ, Deruelles JB, Waterbury M, Herdman RY, Stanier RY. 1979. Generic assignments, strain histories and properties of pure cultures of cyanobacteria. Microbiology. 111(1):1–61.
- Rollwagen-Bollens G, Bollens SM, Gonzalez A, Zimmerman J, Lee T, Emerson J. 2013. Feeding dynamics of the copepod Diacyclops thomasi before, during and following filamentous cyanobacteria blooms in a large, shallow temperate lake. Hydrobiologia. 705(1):101–118.
- Sahuquillo M, Melão MGG, Miracle MR. 2007. Low filtering rates of Daphnia magna in a hypertrophic lake: laboratory and in situ experiments using synthetic microspheres. Hydrobiologia. 594(1):141–152.
- Samel A, Ziegenfuss M, Goulden CE, Banks S, Baer KN. 1999. Culturing and bioassay testing of Daphnia magna using Elendt M4, Elendt M7, and COMBO media. Ecotox Environ Safe. 43(1):103–110.
- Sarnelle O, Wilson AE. 2005. Local adaptation of Daphnia pulicaria to toxic cyanobacteria. Limnol Oceanogr. 50(5):1565–1570.
- Sikora A, Dawidowicz P. 2017. Breakage of cyanobacterial filaments by small- and large sized Daphnia: are there any temperature-dependent differences? Hydrobiologia. 798(1):119–126.
- Sterner RW, Elser JJ. 2002. Ecological stoichiometry-the biology of elements from molecules to the biosphere. Princeton (NJ): Princeton University Press.
- Suzuki-Ohno Y, Kawata M, Urabe J. 2012. Optimal feeding under stoichiometric constraints: a model of compensatory feeding with functional response. Oikos. 121(4):569–578.
- Urabe J, Elser JJ, Kyle M, Yoshida T, Sekino T, Kawabata Z. 2002. Herbivorous animals can mitigate unfavorable ratios of energy and material supplies by enhancing nutrient recycling. Ecol Letters. 5(2):177–185.
- Urabe J, Shimizu Y, Yamaguchi T. 2018. Understanding the stoichiometric limitation of herbivore growth: the importance of feeding and assimilation flexibilities. Ecol Lett. 21(2):197–206.
- Van Donk E, Lurling M, Hessen DO, Lokhorst GM. 1997. Altered cell wall morphology in nutrient-deficient phytoplankton and its impact on grazers. Limnol Oceanogr. 42(2):357–364.
- Wagner ND, Yang Z, Scott AB, Frost PC. 2017. Effects of algal food quality on free amino acid metabolism of Daphnia. Aquat Sci. 79(1):127–137.
- Wilson AE, Sarnelle O, Tillmanns AR. 2006. Effects of cyanobacterial toxicity and morphology on the population growth of freshwater zooplankton: meta-analyses of laboratory experiments. Limnol Oceanogr. 51(4):1915–1924.
- Zhu JY, Lu KH, Sun SZ, Zhang KX. 2013. Foraging inhibition in two c ladocerans feeding on Microcystis aeruginosa. Clean Soil Air Water. 41(7):645–650.