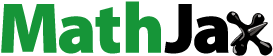
Abstract
Additions of large wood (LW) have become a go-to technique for recovering altered river ecosystems. However, successful applications of this technique are generally limited to unchannelized rivers and headwater streams. Channelization of rivers, that is, engineering river channels to reduce recruitment and retention of in-channel structure, may, by definition, limit success of this restoration technique. Moreover, sufficient time has passed (a century or more) since initial channelization of many large rivers that portions of the fish community associated with LW may have become extirpated. Thus, the maxim that LW leads to a positive fish community response may not hold true. We examined fish community associations in habitats with and without LW in the channelized Missouri River to gain an understanding of the role of LW in large, channelized rivers. There were some differences between habitats with wood present compared to those without, but the differences were not evident once year, season and channel modifications intended to create aquatic habitat were taken into account. We assert that careful planning is necessary to ensure that additions of LW in channelized rivers are made to appropriate locations such that it will be retained in-channel for use as fish habitat and that LW-associated species are found in the system.
Keywords:
Introduction
Societal use of large rivers for navigation, flood control and hydropower has led to declines in native biota (Dudgeon et al. Citation2006; Vörösmarty et al. Citation2010; Pracheil et al. Citation2013). The resulting declines in aquatic organism abundances are due in no small part to river channel alterations including dams and channelization that have fundamentally changed hydrology, storage and export of sediment and nutrients, and channel form and function. Moreover, impoundment and channelization have reduced habitat availability for some fish and other aquatic biodiversity (Wolter and Arlinghaus Citation2003; Dudgeon et al. Citation2006; Vörösmarty et al. Citation2010). Restoration solutions in large rivers, however, are not simple because channel alterations are necessary pieces of human infrastructure and therefore cannot be removed. We must now focus our efforts on gaining a more mechanistic understanding of the patterns, processes and rates of these altered riverscapes to balance the needs of humans and biodiversity.
Engineering large-river channels to support navigation has proved especially inhospitable to riverine biota (Wolter and Arlinghaus Citation2003). Transforming a river into a navigation highway requires creating channels of sufficient depth, clearance and permanence that barges can safely pass. River transformation takes place via removal of obstructions such as large wood (LW) and channelization: that is, armouring banks with rocks or other hard materials to stabilize banks to hydrologically push debris from the navigation channel and quickly shunt water and in-channel debris downstream. Channelization thus has ecosystem-level effects because it changes the rate of recruitment, retention and export of resources in riverine habitats (Guyette et al. Citation2002) which directly affects biota (Kennedy and Turner Citation2011). For example, unchannelized reaches of the Rio Grande River maintain greater energetic connectivity between aquatic and riparian areas as demonstrated by higher abundance of terrestrial carbon (C) composition in macroinvertebrates (Kennedy and Turner Citation2011).
Transforming the lowest 1183 km of the Missouri River to better suit commercial navigation has resulted in changes to river hydrology, geomorphology, productivity and biodiversity. For instance, the removal of in-channel trees beginning in 1838 and channelization beginning in 1912 to facilitate steamship travel has dramatically altered the fish community and contributed to declines of several native species including the federally endangered Pallid Sturgeon Scaphirhynchus albus (Hesse and Sheets Citation1993). The response of fish communities to these channel alterations is not unique to the Missouri River Basin. Similar channel alterations on the lower Rhone River in Europe have led to marked changes in biological communities in that riverine ecosystem such that entire fish assemblages have disappeared (Fruget Citation1992; Jongman Citation2002).
While LW is most certainly important fish habitat in intact large rivers, snag removal and habitat alteration have catalyzed steep declines in fish populations where physical modifications have occurred. As a result, it is not necessarily clear that the remaining native fish communities still respond to LW. Sufficient time has passed since the initial channelization and snag removal activities in the Missouri River that characteristic fish assemblages affiliated with LW may no longer exist. Thus, our general understanding of the fish community response to LW derived in other systems may not apply to anthropogenically altered systems like the Missouri River. Specifically, the role of LW in smaller lotic systems has been well documented (e.g. Angermeier and Karr Citation1984; Harmon et al. Citation1986; Dolloff and Warren Citation2003), yet this information may not be scalable to the Missouri River due to the long legacy of alterations and non-linear, discharge-dependent controls on riverine biota (Oberdorff et al. Citation2011; Pracheil et al. Citation2013). Our study works to fill this knowledge gap by characterizing fish communities in sites with and without LW present along a channelized section of the Missouri River.
Methods
Study area
We sampled 10 sites (eight river bends and two chutes) on the channelized Missouri River between Sioux City, IA (mean daily discharge 848 m3s−1) and the confluence of the Kansas River (mean daily discharge 1,475 m3s−1; ) between May 2007 and September 2008. We used a stratified random sampling design to select study sites representative of habitat modifications that have been used to mitigate effects of channelization through increasing shallow water habitat availability. As such, we selected (1) four bends where wing-dike channelization structures have been lowered and chevrons installed (v-shaped underwater rock structures constructed to collect sediment and create shallow water habitat), hereafter called modified sites, (2) two unregulated historically present chutes that were re-established by cutting trenches into the floodplain and reconnecting to the river at two points and (3) four bends consisting of unaltered wing-dike channelization structures, hereafter called unmodified channelization structure sites.
Figure 1. Map of study area showing locations of sites. Letters on map correspond to sites as follows: (A) Lower Peterson Cut-off Bend, (B) California Chute, (C) DeSoto Cut-off Bend, (D) Lower Calhoun Bend, (E) Boyer Bend, (F) Tobacco Bend, (G) Lower Copeland Bend, (H) Hamburg Chute, (I) Upper Hamburg Bend and (J) Upper Barney Bend. Names of sites represent geographic names and are not necessarily indicative of current habitat conditions (i.e. cut-offs are not currently cut-off habitats). Sites marked with * represent sites modified to mitigate the effects of channelization and sites marked with ** represent chutes.
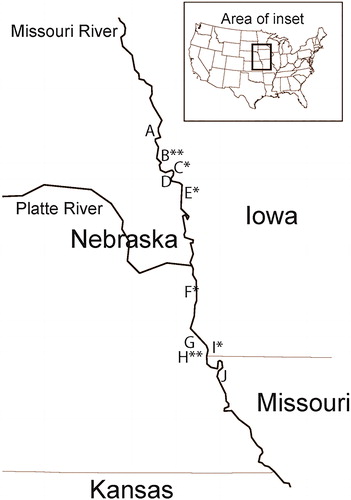
Biota abundance
All sites were sampled seasonally: April–May, June–July and August–September during 2007 and 2008 to determine fish community attributes at sites with and without LW. Sampling occurred at five randomly selected stations containing LW (one accumulation of LW per site) and five randomly selected stations containing open water (OW) per site. All available stations were sampled if study bends contained five or fewer LW stations.
Fish collection methods closely followed those of Lehtinen et al. (Citation1997) and consisted of 5 min electrofishing runs (boat-mounted electrofisher, Smith-Root GPP 5.0, pulsed-DC, 60 Hz, ∼8 amps, with two dip netters) that began approximately 10 m downstream of the snag and continued in an upstream direction ending approximately 10 m upstream of the LW. OW stations were sampled in a similar manner and covered approximately the same amount of surface area (e.g. 20–30 m). Fish species abundances were recorded in the field and returned to the water near the point of capture; however, fish that we could not easily identify in the field were preserved in 10% formalin and identified in the laboratory. Habitat classifications were recorded in accordance with the Missouri River Standard Operating Procedures for Sampling and Data Collection (Drobish Citation2006) and included recording GPS coordinates, LW dimensions (diameter estimated at water surface and length using sonar) and condition, maximum water depth, surface water temperature, surface water velocity (m*s−1), conductivity (µS/cm) and dominant substrate type (sand, silt, gravel) at each fish sampling station. We also used a snag rating index devised by Lehtinen et al. (Citation1997) to describe size and complexity of snags at each station (). This index requires assigning scaled values to three parameters that characterize LW, ranges from 0 to 12, and was calculated as
where Ni = the number of major branches of the LW snag i (ranging from 0 to 4), Ci = the circumference in mm of the LWi snag (ranging from 0 to 4) and Ri = roughness of the LW snag i (ranging from 0 to 4). Sites without LW snags were assigned a snag rating index of 0.
Table 1. Snag rating index from Lehtinen et al. (Citation1997).
Data analysis
We used an analysis of variance (ANOVA) to determine if there were differences in habitat characteristics between LW and OW habitats such as water depth, velocity, conductivity, dissolved oxygen and turbidity as well as the Shannon diversity index of the fish community while controlling for year, season, bend and modification type (α < 0.05). We summarized the relative abundance of fish species irrespective of year, season or habitat modification using catch per unit effort (CPUE; fish/min). Not all components of the fish community may respond similarly to LW, so we examined the biotic response of the (1) entire fish community, (2) large-river specialist fish community and (3) small-bodied fish community separately. We chose to specifically examine the response of small-bodied and large-river specialist fish because these groups are of conservation concern in the Missouri River (Pracheil et al. Citation2013). Additionally, efforts to mitigate biodiversity declines caused, in part, by channelization have led to two general types of experimental channel modifications (constructed chutes and wing-dike modification) in the Missouri River. We therefore controlled for modification type in our analysis to determine if these modifications influence fish community response to LW.
We used a non-metric multidimensional scaling (NMDS) ordination of sites, stratified by site modification (R Core Team Citation2013; metaMDS, Vegan package; Oksanen et al. Citation2016) to visually display fish community composition and structure in LW or OW sites. An NMDS is an unconstrained ordination technique that can be used to visualize differences between sites, where the distance between sites is directly relational to differences in communities based on rank orders of a dissimilarity matrix (in our case, a Bray–Curtis matrix). Fish abundance data were Wisconsin double standardized prior to analysis. We coupled the NMDS ordination with an analysis of similarity (ANOSIM) to test whether or not LW and OW fish communities were different (P ≤ 0.05; R < 0.20). The ANOSIM is allied with NMDS, in that it also uses rank orders of the dissimilarity matrix to statistically differentiate between groups. Initial analyses indicated that there were no differences in fish community structure either seasonally or annually within a given bend type (e.g. unmodified channelization structure, modified, chute; P > 0.05; R > 0.20). Therefore, we were able to combine sample data within a bend for a given habitat type. Where the ANOSIM identified differences in fish communities between LW and OW habitats, we used a similarity percentage (SIMPER) analysis to determine which fish species contributed to that difference (Vegan package; Oksanen et al. Citation2016).
We calculated Shannon–Weiner diversity values for the entire fish community at all LW and OW stations for each site to compare community diversity. We then tested for the effect of site modification (i.e. unmodified channelization structure, modified, chute) on the relationship between community diversity and snag complexity using an ANCOVA (α < 0.05). We excluded stations with a snag index rating of 0 because we were interested in a gradient of snag complexity in this analysis.
Results
We sampled a total of 2733 fish at 588 stations during 2007 and 2008. Our sampling included 252 snags with a mean ± SD snag rating index of 7.4 ± 2.59 and ranging in snag index value from 1 to 12 (). There were differences in physical properties of LW and OW habitats (); stations with LW were associated with higher velocity, turbidity and depth. However, the differences appeared to be minor and may reflect variability encompassed in measurement precision rather than meaningful differences.
Figure 2. Frequency of snag rating index scores of 252 stations where the large wood-associated fish community was sampled.
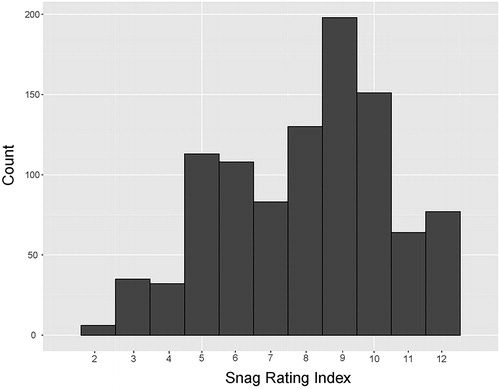
Table 2. Results of ANOVA of effect of year, season (spring, summer, fall), modification (modified/unmodified channelization structure, chute) and treatment (open water or large wood) on habitat charactics, including water depth, dissolved oxygen, turbidity (µS/cm) and water velocity (m/s) from sample sites.
There were no differences in overall fish community with respect to year, season, habitat modification or presence/absence of LW. Stress values for the two-dimensional NMDS plots shown in ranged from 0.2 to 0.5 (). For all species assemblages, four-dimensional NMDS provided stress values below 0.1. We chose to display dimensions one and two because they provided the greatest marginal reduction in stress values, and the results were consistent with information from our ANOSIM analysis. Presence of LW had a significant effect on the overall fish community (ANOSIM: R = 0.15, P = 0.04; ), although there were no differences in components of the fish community on the Missouri River that are of conservation concern (ANOSIM: large-river specialist: R = 0.05, P = 0.18; small-bodied: R = 0.06, P = 0.15; ). Gizzard shad Dorosoma cepedianum was the most abundant species sampled and was primarily associated with OW, while common carp Cyprinus carpio was the most abundant species associated with LW for the entire fish community analysis (). Gizzard shad, emerald shiner Notropis athernoides, common carp, red shiner Cyprinella lutrensis, river carpsucker Carpiodes carpio and flathead catfish Pylodictus olivarus relative abundances accounted for 58% of the difference in LW and OW communities for the SIMPER analysis (). Only flathead catfish is a large-river specialist species, whereas emerald shiner and red shiner are small-bodied fishes. Furthermore, LW and OW fish communities in the Missouri River are different, but these differences do not lead to separation in overall fish community diversity when bend modification was considered (ANCOVA: F3,16= 0.55; P = 0.66).
Figure 3. Non-metric multidimensional scaling plot of fish species (hollow dots) and bends (letters; N = 10) containing no large wood (black letters) and large wood (gray letters) for (a) all species (N = 44 species; N = 2,732 fish), (b) large-river specialist species (N = 20 species; N = 766 fish) and (c) small-bodied species (N = 17 species; N = 614 fish). Polygons were constructed by joining the centroids from sites with Open Water (OW – polygon connecting black letters) and those containing Large Wood (LW – polygon connecting gray letters). Overlapping dots and letters are hidden for clarity.
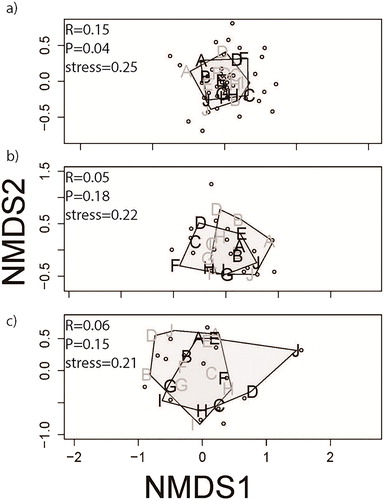
Figure 4. Catch per unit effort (CPUE; fish/min) of fish associated with Open Water (OW – black bars) and Large Wood (LW – gray bars). Error bars represent ± 1 SE.
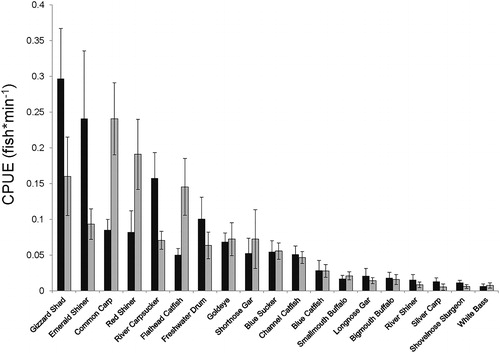
Figure 5. Cumulative proportion (SIMPER) of upper 90th percentile of species contributing to fish community differences between Open Water (OW) and Large Wood (LW) habitats. A * by a species name denotes non-overlapping CPUE SE bars () between LW and OW treatments. Species in gray text were significantly associated with LW (as determined by non-overlapping CPUE SE bars). Species in black text with a * represent species that were associated with OW (as determined by non-overlapping CPUE SE bars). Species in black without * showed no preference for either LW or OW habitats.
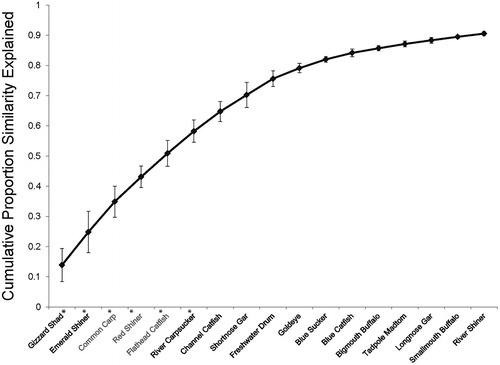
Discussion
Our study found that there were some limited species-specific differences in abundance between LW and OW sites, but these differences did not translate to clear separation in fish communities between habitat types in the Missouri River. Our findings posit the ideas that (1) LW may not be as important as generally assumed in large rivers that have been altered extensively and for an extended period of time and/or (2) that the sector of the fish community that historically relied more heavily on LW no longer exists. The lack of detectable association between fish species abundance and diversity to the presence of LW in the channelized Missouri River compared to results from other systems may be due to several reasons. It may be that historically, LW has not been important to the native fish community. However, this explanation is unlikely due to the historic abundance of LW in the Missouri River (Hesse and Sheets Citation1993) and the large number of studies reporting the importance of LW to fish communities and populations across scales of lotic ecosystems (e.g. Angermeier and Karr Citation1984; Lehtinen et al. Citation1997; Johnson et al. Citation2005). Perhaps, more likely is the long legacy of snag removal and channelization on the Missouri River coupled with low availability, recruitment and retention of LW into the current system (Archer Citation2010) that may have led to extirpation of species reliant on LW from this portion of river. For example, we show that the bulk of the contemporary fish community either does not respond to LW differently from OW or responds positively to OW habitats, a potential outcome of selection against species that rely solely on LW.
The spatial scale of alterations that have occurred on the Missouri River may have been greater than the spatial scale at which many native species complete their life history. Recent work has shown that for habitat heterogeneity to benefit species and overcome negative effects of alterations, improvements must be made at a scale relevant to the life history of target organisms. Lepori et al. (Citation2005) showed, for instance, that fish and macroinvertebrate communities on the Ume River in Sweden did not respond to habitat improvements aimed at increasing habitat complexity because the spatial scale of the alterations was large relative to both the spatial scale of habitat improvement and the organisms in the biotic community. Our study did not focus on the addition of LW as a means of restoration; however, the same principle – that heterogeneity (in this case, LW) must be present at a scale at which organisms can detect such – may still apply. Fish communities in the channelized Missouri River may not be detecting LW to use as structure because it is too sparse as a result of the low retention of LW in the system (Archer Citation2010).
We can gain further understanding of the interactions between LW and channelization by comparing our study with Lehtinen et al. (Citation1997) who surveyed LW and OW sites in channel border and backwater habitats in Pool 6 of the Mississippi River – an unchannelized system similar in size (mean daily discharge 838 m3s−1) to the Missouri River – and found LW to be important for many native fish species. Similar to our study, Lehtinen et al. (Citation1997) found common carp to be more abundant in LW locations, but several other species they found were absent or rare in our study despite our study area being within many of these same species’ ranges. Specifically, species of fish commonly associated with LW in the Lehtinen et al. (Citation1997) study such as bluegill Lepomis macrochirus, bigmouth buffalo Ictiobus cyprinellus and piscivorous smallmouth bass Micropterus dolomieu, largemouth bass M. salmoides, black crappie Pomoxis nigromaculatus, walleye Sander vitreus and sauger S. canadense were not common in our study. Interestingly, Lehtinen et al. (Citation1997) also found emerald shiner to be associated with LW where we found this species associated with OW. Species found in the Lehtinen et al. (Citation1997) study associated with LW that were rare or absent in our study are generally associated with slower velocity currents (Pegg and Pierce Citation2002): a rare environmental condition in the channelized Missouri River. In fact, stations containing LW had higher current velocities than stations with OW (), suggesting that the multiple benefits for fish to be associated with LW such as increased cover and food availability are not realized. However, we were unable to measure velocities directly within snags where there was a high diversity of current velocities, including areas of lower velocity. Because we do not have these measurements, we are unable to directly compare within snag velocities with OW current velocities, although we assume that some areas of within snag velocity were lower than those reported here.
The Missouri River now has substantially less in-channel LW than the pre-alteration river; however, because fish communities have changed as a result of these channel alterations (Pegg and Pierce Citation2002), additions of LW to this and other channelized rivers are not likely to engender a broad, positive response from the native fish community. As such, the results of our study further demonstrate the need for appropriate process-based restoration approaches (Beechie et al. Citation2010) to recover highly altered river ecosystems. Additions of LW in river ecosystems have proven a very effective technique for restoring some riverine habitats (e.g. Dolloff and Warren Citation2003; Acuña et al. Citation2013), particularly where the recruitment of LW to a river ecosystem has only recently been lost or reduced due to human activities like tree harvest. In these ecosystems, the lack of LW is the primary problem, and in adding LW, the geomorphic and biologic functions provided by these additions can quickly aid fishes. For instance, additions of LW to Tenmile Creek, OR, were responsible for an immediate increase in young-of-year steelhead Onchorhynchus mykiss survival (Johnson et al. Citation2005). However, channelized rivers like the Missouri River have endured over a century of ecosystem alterations – namely in-channel snag removal, loss of floodplain-channel connectivity and reduced channel complexity – that have likely resulted in changes to the overall fish community structure due to required use of human-made habitats by native fishes (Ridenour et al. Citation2009). We show in this study that the native fish community, especially portions of the native fish community of concern, may not respond to a simple addition of LW if restoration attempts are implemented because the baseline species set may no longer be present to benefit from habitat improvements. Rather, careful planning will be needed to ensure not only that LW habitat is available in appropriate locations but also the species that have or will use such habitat are also found in the system. Moreover, the effects of channelization not only reduce the recruitment and retention of LW (Archer Citation2010) but potentially trump the geomorphic and hydrologic influence of in-channel wood. Given LW has been shown to reside in-channel for as much as 800 years (Guyette et al. Citation2002), it may well be that recovering geomorphic and biologic processes lost from massive snag removal and subsequent channel engineering activities may take decades to centuries.
Notes on contributors
Michael W. Archer is the state lakes coordinator for the Nebraska Department of Environmental Quality, Surface Water Unit.
Brenda M. Pracheil is currently an aquatic ecologist and research and development staff at Oak Ridge National Laboratory.
Alexandrea E. Otto is currently an undergraduate student completing her BS degree in Fisheries and Wildlife at the University of Nebraska-Lincoln.
Mark A. Pegg is a fish ecologist and professor at the University of Nebraska-Lincoln.
Acknowledgements
We thank G.E. Mestl, A. Barada, M. Hamel and A. Blank for sampling assistance.
Disclosure statement
No potential conflict of interest.
Additional information
Funding
References
- Acuña V, Díez JR, Flores L, Meleason M, Elosegi A. 2013. Does it make economic sense to restore rivers for their ecosystem services?. J Appl Ecol . 50(4):988–997.
- Angermeier PL, Karr JR. 1984. Relationships between woody debris and fish habitat in a small warmwater stream. Trans Amer Fisher Soc. 113(6):716–726.
- Archer MW. 2010. Retention, movement, and the biotic response to large woody debris in the channelized Missouri River [master’s thesis]. Lincoln (NE): University of Nebraska.
- Beechie TJ, Sear DA, Olden JD, Pess GR, Buffington JM, Moir H, Roni P, Pollock MM. 2010. Process-based principles for restoring river ecosystems. BioScience. 60(3):209–222.
- Dolloff CA, Warren ML. Jr, 2003. Fish relationships with large wood in small streams. In: Gregory, S, Boyer, KL, Gurnell, AM, editors. The Ecology and Management of Wood in World Rivers American Fisheries Society, Symposium, Vol. 37, Bethesda, Maryland, p. 179–193.
- Drobish M. editor. 2006. Missouri River standard operating procedures for sampling and data collection, Vol. 1.1. SD: U.S. Army Corps of Engineers; p. 162.
- Dudgeon D, Arthington AH, Gessner MO, Kawabata ZI, Knowler DJ, Lévêque C, Naiman RJ, Prieur-Richard A, Soto D, Stiassny ML, Sullivan CA. 2006. Freshwater biodiversity: importance, threats, status and conservation challenges. Biol Rev. 81(02):163–182.
- Fruget JF. 1992. Ecology of the lower Rhône after 200 years of human influence: a review. Regul Riv: Res Mgmt. 7(3):233–246.
- Guyette RP, Cole WG, Dey DC, Muzika RM. 2002. Perspectives on the age and distribution of large wood in riparian carbon pools. Can J Fish Aquat Sci. 59(3):578–585.
- Harmon ME, Franklin JF, Swanson FJ, Sollins P, Gregory SV, Lattin JD, Anderson NH, Cline SP, Aumen NG, Sedell JR, et al. 1986. Ecology of coarse woody debris in temperate ecosystems. Adv Ecol Res. 15:133–302.
- Hesse LW, Sheets W. 1993. The Missouri River hydrosystem. Fisheries. 18(5):5–14.
- Johnson SL, Rodgers JD, Solazzi MF, Nickelson TE. 2005. Effects of an increase in large wood on abundance and survival of juvenile salmonids (Oncorhynchus spp.) in an Oregon coastal stream. Can J Fish Aquat Sci. 62(2):412–424.
- Jongman R. 2002. Homogenisation and fragmentation of the European landscape: ecological consequences and solutions. Lands Urb Plan. 58(2-4):211–221.
- Kennedy TL, Turner TF. 2011. River channelization reduces nutrient flow and macroinvertebrate diversity at the aquatic terrestrial transition zone. Ecosphere. 2:1–13.
- Lehtinen RM, Mundahl ND, Madejczyk JC. 1997. Autumn use of woody snags by fishes in backwater and channel border habitats of a large river. Environ Biol Fish. 49:7–19.
- Lepori F, Palm D, Brännäs E, Malmqvist B. 2005. Does restoration of structural heterogeneity in streams enhance fish and macroinvertebrate diversity? Ecol Appl. 15(6):2060–2071.
- Oberdorff T, Tedesco PA, Hugueny B, Leprieur F, Beauchard O, Brosse S, Dürr HH. 2011. Global and regional patterns in riverine fish species richness: a review. Int J Ecol. 2011:12.
- Oksanen O, Blanchet FG, Kindt R, et al. 2016. Vegan: community ecology package. R Package Version 2.3-5. accessed [3 October 2018]. http://CRAN.R-project.org/package=vegan
- Pegg MA, Pierce CL. 2002. Fish community structure in the Missouri and lower Yellowstone rivers in relation to flow characteristics. Hydrobiologia. 479(1/3):155–167.
- Pracheil BM, McIntyre PB, Lyons JD. 2013. Enhancing conservation of large-river biodiversity by accounting for tributaries. Front Ecol Environ. 11(3):124–128.
- R Core Team. 2013. R: A language and environment for statistical computing. R Foundation for Statistical Computing, Vienna, Austria. http://www.R-project.org/.
- Ridenour CJ, Starostka AB, Doyle WJ, Hill TD. 2009. Habitat used by Macrhybopsis chubs associated with channel modifying structures in a large regulated river: implications for river modification. River Res Appl. 25(4):472–485.
- Vörösmarty CJ, McIntyre PB, Gessner MO, Dudgeon D, Prusevich A, Green P, Glidden S, Bunn SE, Sullivan CA, Reidy Liermann C, et al. 2010. Global threats to human water security and river biodiversity. Nature. 467(7315):555–561.
- Wolter C, Arlinghaus R. 2003. Navigation impacts on freshwater fish assemblages: the ecological relevance of swimming performance. Rev Fish Biol Fish. 13(1):63–89.