Abstract
Stream fish rely on a mix of terrestrial and aquatic prey sources. While the importance of terrestrial invertebrates as a food source for stream fish is well documented, the role of aquatic insects that emerge from the stream as winged adult insects (aquatic winged adults) and return to the stream as prey is less understood. In this study we determined the proportion of total diet for stream-rearing juvenile Coho Salmon (Oncorhynchus kisutch) that is derived from terrestrial and aquatic winged adult invertebrates which enter the stream from riparian habitats and consider how those cross-ecosystem prey contributions vary based on riparian habitat type. Study reaches were identified in three streams within the Kenai River watershed of Alaska that were representative of habitats found throughout the region and riparian vegetation was classified into grass/sedge, shrub and tree types using LiDAR. Juvenile Coho Salmon stomach contents were sampled seasonally in study reaches over a two-year period and ingested invertebrates were identified by taxa, life stage and origin. Our results showed that aquatic winged adult prey contributions to juvenile salmon diet were significantly lower in the grass/sedge study reach, and cross-ecosystem invertebrate prey represented a significantly higher proportion of juvenile salmon diet in the tree study reach. Invertebrate prey in the grass/sedge reach were composed primarily of the larval life stage of aquatic winged adults. These results suggest that change in riparian vegetation from tree/shrub to grass/sedge along Kenai streams as projected by regional climate change models, or that results from anthropogenic modification, will likely lead to lower availability of cross-ecosystem prey for stream fish. Management of riparian buffers along streams to preserve or increase occurrence of trees and shrubs is likely to help mitigate impacts of those possible changes.
Introduction
Streams are connected to adjacent terrestrial habitats by the movement of prey and other resources between ecosystems (Polis et al. Citation1997; Baxter et al. Citation2005; Richardson et al. Citation2010). Terrestrial invertebrates that enter streams supplement in-situ prey sources for stream-rearing salmonids, and often provide half or more of their annual energy intake (Hunt and Krokhin Citation1975; Wipfli Citation1997; Kawaguchi and Nakano Citation2001). Terrestrial prey represented 50% of the seasonal biomass ingested by juvenile salmonids in several southeast and southcentral Alaska streams (Wipfli Citation1997; Roon et al. Citation2018). Kawaguchi and Nakano (Citation2001) found terrestrial invertebrates supported 49% of annual prey consumption by salmonids in forested stream reaches and 53% in grassland reaches of a northern Japanese stream. Over the course of the summer, terrestrial invertebrate prey generally become more numerous and increase in availability while larval aquatic invertebrate densities generally decrease due to predation and emergence from streams as adults (Merritt and Cummins Citation1996; Kawaguchi and Nakano Citation2001; Sweka and Hartman Citation2008). Terrestrial prey can comprise 50–90% of fish diet during summer months (Garman Citation1991; Wipfli Citation1997; Baxter et al. Citation2005).
Riparian vegetation type plays a major role in determining the quantity of terrestrial invertebrates that enter streams. Riparian vegetation overhanging streams promotes in-fall of terrestrial invertebrates to streams (Cadwallder et al. Citation1980), and different riparian tree species contribute different quantities of terrestrial invertebrate biomass to streams (Mason and Macdonald Citation1982). In Alaska, dense shrub understory associated with riparian alder increased terrestrial invertebrate subsidies to streams and deciduous trees supported more foliar invertebrate mass than conifers (Wipfli Citation1997; Allan et al. Citation2003). Edwards and Huryn (Citation1995) found that terrestrial invertebrates made only a small contribution to trout diet in a New Zealand pasture stream, and Kawaguchi and Nakano (Citation2001) found that the annual input of terrestrial invertebrates was 1.7 times greater for forested than grassland stream reaches in a low-order Japanese stream.
The larval life stages of aquatic winged adult taxa such as Plecoptera, Trichoptera, Ephemeroptera and Diptera are abundant in many lotic environments and represent an important prey resource for stream fishes, especially during seasons when terrestrial inputs are low. These taxa emerge as winged adults from streams during hatching events and enter riparian habitats (Collier and Smith Citation1997; Petersen et al. Citation1999; Briers et al. Citation2005). The return of aquatic winged adult insects from riparian habitat to the stream represents a potentially important source of prey for stream fishes, however, this trophic pathway is not well understood. This study is intended to address this knowledge gap and more generally consider the implications of riparian habitat change on invertebrate prey contributions to fish diet.
After aquatic winged adult insects emerge from the stream, swarming, mating, foraging and sheltering occur in riparian habitats (). Lateral movement of those insects away from the stream is influenced by streamside vegetation (Jackson and Fisher Citation1986; Bohonak and Jenkins Citation2003; Winterbourn et al. Citation2007), and dispersal behavior appears selective based on habitat preferences for food and shelter (Kuusela and Huusko Citation1996; Petersen et al. Citation1999; Delettre and Morvan Citation2000), as well as weather conditions and insect flying ability (Briers et al. Citation2003; Parkyn and Smith Citation2011; Greenwood Citation2014). Riparian shrubs reduced lateral dispersal of aquatic winged adult insects (Ephemeroptera, Plecoptera, Trichoptera and Megaloptera) when compared to grasslands in New Zealand (Greenwood Citation2014). Delettre and Morvan (Citation2000) found that in open agricultural landscapes of Brittany, adult chironomids moved away from their natal stream, with extent of lateral dispersal related to landscape openness. Movement by aquatic winged adults away from their natal stream reduces their chances of returning to the stream and becoming potential prey for stream fishes (Jackson and Fisher Citation1986; Briers and Gee Citation2004).
In this study, we measured contributions by terrestrial, aquatic and aquatic winged adult invertebrates to the diet of stream-rearing juvenile Coho Salmon Oncorhynchus kisutch within the Kenai River watershed of southcentral Alaska and calculated the total invertebrate contribution derived from invertebrates that enter the stream from riparian habitats (cross-ecosystem). Invertebrate contributions to juvenile salmon diet were compared among three study reaches representative of common riparian vegetation types found within the Kenai watershed. We hypothesized that terrestrial and aquatic winged adult invertebrates were an important component of juvenile Coho Salmon diet that is moderated by vegetation type. Our prediction was that the fractional contribution to juvenile salmon diet of aquatic winged adult and cross-ecosystem invertebrates would be lower in streams with more open riparian habitats (grass/sedge) than those in shrub or tree riparian habitats.
Materials and methods
Study area
This study was conducted within the Kenai River watershed of southcentral Alaska which covers approximately 5,600 sq km and includes over 2,600 km of mapped tributary streams and rivers (). The Kenai River watershed supports Pink Salmon O. gorbusha, Chum Salmon O. keta, Sockeye Salmon O. nerka, Chinook Salmon O. tshawytscha, Coho Salmon, and Rainbow Trout and Steelhead O. mykiss.
Figure 2. Study watersheds within the Kenai River watershed of Southcentral Alaska. Fish sampling sites on each study reach are indicated by numbers 1 and 2.
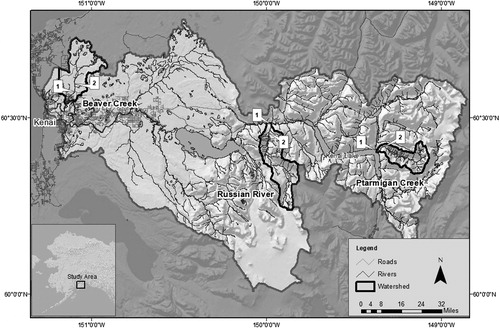
Extended, homogeneous study reaches within three salmon-rearing tributaries (Beaver Creek, Ptarmigan Creek, Russian River) of the Kenai River were chosen for study that were representative of the most common vegetation types (grass/sedge, shrub, tree) occuring along streams in the Kenai watershed (). These extended study reaches were selected to avoid mixing of invertebrate input from diverse vegetation types or the movement of juvenile salmon among riparian habitats (Wipfli Citation1997; Allan et al. Citation2003; Roon et al. Citation2016). Sampling replicates of similar extended, homogeneous reaches was not practical, consequently we could not statistically assess effects of riparian type. This study therefore provides a statistical comparison of study reaches and considers how observed differences in prey contributions could be attributed to variation in riparian type.
Beaver Creek (BC) is a lowland stream with an overall 2% average gradient and 0% direct glacial influence. Vegetation adjacent to the BC study reach was predominately grass and sedge (Calamagrostis spp., Deschmapsia spp., Arctogrostis spp., and Carex spp.). Ptarmigan Creek (PC) is a higher elevation glacially fed stream with an overall 14% average gradient and 7% glacial watershed coverage. Vegetation adjacent to the PC study reach was predominantly shrubs (Alnus spp. and Salix spp.) as well as deciduous (Betula spp. and Populus spp.) and coniferous trees (Picea spp.). Russian River (RR) is a montane tributary with an overall 9% average gradient and has minor (< 1%) glacial coverage within its watershed. Vegetation adjacent to the RR study reach is dominated by mixed deciduous and coniferous trees (Betula spp., Populus spp. and Picea spp.) with deciduous shrub (Alnus spp. and Salix spp.) understory. While areas within the lower Kenai River watershed have experienced substantial human development, the study reaches were generally undeveloped, and as a result riparian habitats were relatively intact and undisturbed.
Characterizing vegetation type
Remote sensing data are useful for mapping ecological patterns (Dauwalter et al. Citation2017), and in this study we used LiDAR data to classify vegetation type adjacent to the three study reaches according to height. The LiDAR data were collected at a horizontal resolution of 1.2 m with an expected vertical accuracy of 0.15 m. Vegetation height categories were chosen based on vegetation classes defined for Alaska vegetation (Viereck et al. Citation1992): grass/sedge 0.0–0.6 m shrub >0.6–6.0 m and tree > 6.0 m. Vegetation was classified 150 m upstream/downstream and 20 m inland from the stream bank at fish sampling sites and along the study reaches.
Juvenile salmon sampling
Fish sampling sites of 150 m were identified at the upper and lower end of each of the three study reaches. Repeat sampling of juvenile Coho Salmon occurred at each sample site during Spring (June), Summer (July) and Fall (August–September) for 2015 and 2016 (). At each sampling event, six to 12 Gee® minnow traps with 6.4 mm mesh were randomly placed. Traps were baited with sterilized salmon roe in perforated Whirl-Pak® bags, and submerged 15–45 cm. A total of 1302 traps were set for an average period of 2.5 ± 1.4 hours (mean ± SD).
Captured juvenile salmon were anesthetized by submersion in a bath of AQUI-S 20ETM administered at 20 mg/L for 2 to 3 minutes or until fish exhibited total loss of equilibrium. Fork length was measured to the nearest millimeter, and weight was measured to the nearest tenth of a gram with an electronic balance. Gastric lavage sampling of a random subset of captured fish with fork length greater than 50 mm was conducted to determine diet. Stomach contents were preserved in 70% ethanol. All fish were released near the point of capture when sampling was complete at the end of each day. Juvenile Coho Salmon with no stomach contents or with salmon eggs as stomach contents were not included in the analysis ().
Table 1. Fish sampling site, dates and number of fish sampled within each study reach.
Identifiable invertebrates from stomach samples were classified to Family, or the next highest reliable taxa and life stage (Merritt and Cummins Citation1996). Length of partially digested prey was estimated based on intact individuals of the same taxon that appeared similar in size (Wipfli Citation1997). Estimation of prey dry mass was based on measured invertebrate body length with the allometric formula W = aLb, where W is total dry body mass, L is total body length, and a and b are constants of the regression between W and L (Ricker Citation1973). Length–mass regression constants a and b were derived from a database of allometric juvenile salmon prey length–weight relationships (B.E. Meyer, unpublished data).
Invertebrate taxa were grouped into three primary prey categories based on origin and life stage: aquatic (larval and adult life stages of invertebrates that originate and reside in-stream), terrestrial (invertebrates that originate and reside in the terrestrial environment) and aquatic winged adult (aquatic-born invertebrates that emerge from the stream to the terrestrial environment as winged adults). The origin of some adult Diptera prey taxa were unknown, and were categorized as Diptera of unknown origin. The cross-ecosystem contribution to juvenile salmon diet that resulted from the movement of invertebrates from the terrestrial environment to the stream was calculated as the sum of terrestrial, aquatic winged adult and adult Diptera of unknown origin categories.
The percentage of total invertebrate prey consumed (dry mass) was calculated for each prey category in each study reach to allow comparison of invertebrate prey consumption among study reaches. Dry mass values of fish stomach contents were standardized for fish length to minimize fish-size bias. Arcsine-square-root transformation of percentages was used to standardize variance and improve normality of the data for statistical analysis. One-way analysis of variance (ANOVA-SPSS v.24) of transformed values was used to evaluate the difference between study reaches for invertebrate prey categories using a robust test of equality of means (Welch) and post-hoc Games-Howell tests where equal variances are not assumed (SPSS v.24) were used to conduct pairwise comparison of means between study reaches. Values were considered significant at α < 0.05. Lack of replication in treatment categories limits inference to the three study reaches.
Results
Vegetation type
The composition of vegetation types adjacent to sample sites differed substantially between study reaches (). Grass/sedge represented 89%, 12% and 8%, shrubs 8%, 55% and 43%, and trees represented 3%, 33% and 49% at sample sites on BC, PC and RR, respectively. Riparian vegetation types along study reaches were similar to vegetation type found at sampling sites ().
Invertebrate contributions to juvenile Salmon diet
Stomach contents of 405 juvenile Coho Salmon were analyzed during the 2015 (n = 210) and 2016 (n = 195) field seasons. In 2015, 76 distinct combinations of taxa and life stage were identified among 2,481 total diet items while in 2016, 96 distinct combinations of taxa and life stage were identified among 6,822 total diet items.
The dominant aquatic taxa ingested by juvenile salmon were similar for all study reaches and consisted primarily of larval life forms of aquatic winged adults including: fly larvae (Diptera), caddisfly larvae (Trichoptera), mayfly larvae (Ephemeroptera) and stonefly larvae (Plecoptera)) (). These taxa represented 92%, 88% and 95% of the aquatic biomass in juvenile salmon diet for BC, PC and RR. Wasps and ants (Hymenoptera) were the largest contributors of terrestrial invertebrate biomass for each of the study reaches providing 39%, 39% and 31% of the total terrestrial contribution for BC, PC and RR. Butterfly/moth larvae (Lepidoptera) represented a larger percentage by weight of total invertebrates consumed by salmonids within PC (20%) and RR (9%) as compared to BC (0%), however, they were infrequent in stomach contents (n = 17) during the 2015-2016 sampling periods. The combined aquatic winged adult contributions to juvenile salmon diet by adult fly (Diptera), adult mayfly (Ephemeroptera) and adult caddisfly (Trichoptera) represented 100% and 96% within BC and PC respectively for those study reaches. Those taxa represented 74% of aquatic winged adult invertebrates consumed by juvenile salmon in the RR, and adult stonefly (Plecoptera) represented 26%.
Table 2. Percent of total dry mass consumed by juvenile salmon by taxa for invertebrate prey categories within each study reach.
The contribution of aquatic winged adult invertebrate prey to juvenile salmon diet was 5%, 9% and 15% for BC, PC and RR, respectively ( and and ) which was statistically different among study reaches (F2, 234.7= 4.431, P = 0.013). Pair-wise comparison of BC and RR showed significant differences for aquatic winged adult prey contributions (P = 0.013). Adult Diptera of unknown origin contributed 17%, 12% and 26%, respectively, to total invertebrate prey consumed by juvenile salmon in BC, PC and RR which was statistically significant among study reaches (F2, 260.8= 6.079, P = 0.003). Pair-wise comparison between PC and RR showed significant difference for adult Diptera of unknown origin (P = 0.002). The proportion of juvenile salmon diet represented by aquatic invertebrates was significantly different among study reaches (F2, 260.4= 13.707, P < 0.001) representing 62%, 55% and 37%, respectively, for BC, PC and the RR. Post-hoc comparison showed significant differences between RR and BC (P < 0.001) and PC (P = 0.001). Terrestrial invertebrate prey contributed 15%, 22% and 21% for BC, PC and RR, respectively; however, these differences were not significantly different (F2, 252.6 =1.548, P = 0.215). Contributions by all cross-ecosystem sources of invertebrate prey were 38%, 45% and 63%, respectively, for BC, PC, and RR () which differed significantly among study reaches (F2, 260.5=12.453, P < 0.001). Pair-wise comparison showed significant difference in cross-ecosystem invertebrate prey consumed by juvenile salmon in RR as compared to PC (P = 0.003) and BC (P < 0.001).
Figure 5. Percent of total prey dry mass (all years/dates) ingested by juvenile Coho Salmon in each study reach for each invertebrate prey category (mean ± std dev). Study reaches are: Beaver Creek (BC), Ptarmigan Creek (PC) and Russian River (RR). Note: Axis differ on plots.
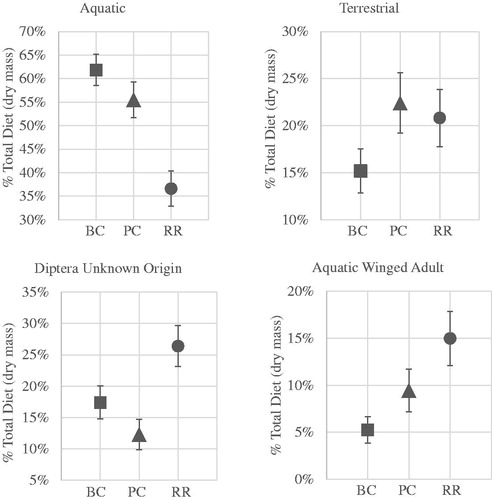
Figure 6. Percent of total prey dry mass ingested by juvenile Coho Salmon for cross-ecosystem and aquatic invertebrate prey categories within each study reach.
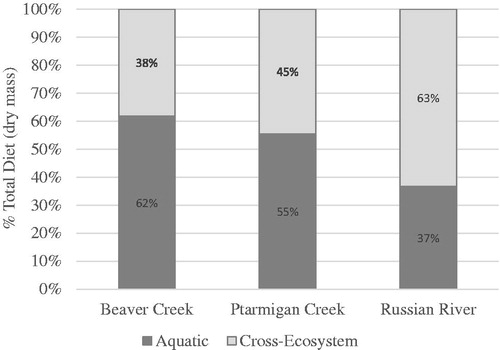
Table 3. ANOVA results for comparison among study reaches of the percent total invertebrate prey consumed (dry mass) by each invertebrate prey category using a robust test of equality of means (Welch). Degrees of freedom, F and P statistics provided. Values were considered significant at α < 0.05.
Table 4. Significance of post-hoc pair-wise comparisons (Games-Howell) of percent total invertebrate prey dry mass consumed by invertebrate prey category for study reaches. Values were considered significant at α < 0.05. Study reaches are: Beaver Creek (BC), Ptarmigan Creek (PC) and Russian River (RR).
Seasonal contribution of invertebrate prey to juvenile salmon diet varied among study reaches (). Aquatic invertebrates provided the majority (>50%) of prey resources to juvenile salmon in BC and PC throughout the year. In RR, aquatic invertebrate contributions decreased below 50% in Spring which was coincident with increased fractional consumption by juvenile salmon of Diptera of unknown origin and aquatic winged adult invertebrate prey and again in Fall when terrestrial and Diptera of unknown origin prey provided increased fractional contributions to diet. During Spring in BC, a reduction in the proportion of aquatic prey consumption was coincident with an increase in the proportion of Diptera of unknown origin consumed by juvenile salmon.
Discussion
Our results support the prediction that the fractional contribution to juvenile salmon diet of aquatic winged adult and cross-ecosystem invertebrates would be lower in streams with more open grass/sedge riparian habitats than those in shrub or tree riparian habitats. We found the proportion of juvenile Coho Salmon diet provided by cross-ecosystem invertebrate prey (terrestrial, aquatic winged adult and Diptera of unknown origin) was significantly lower in grass/sedge (BC) and shrub (PC) study reaches than was observed in the tree (RR) study reach (38%, 45% and 63%, respectively). Observed differences in cross-ecosystem prey contributions among study reaches resulted in part from variation in the proportion of aquatic winged adults and Diptera of unknown origin in juvenile salmon diet. Aquatic winged adult and Diptera of unknown origin together contributed 22%, 21% and 41%, respectively, to juvenile salmon diet in grass/sedge, shrub and tree study reaches. The immature form of these taxa represented the dominant aquatic resident taxa consumed by juvenile salmon in each of the study reaches; however, the proportion of aquatic winged adult invertebrates that returned to the stream, and were consumed by juvenile salmon was significantly lower in the grass/sedge (5%) vs. tree (15%) reaches and Diptera of unknown origin provided a greater proportion of prey contributions to diet for juvenile salmon in the tree study reach (26%) as compared to the grass/sedge (17%) and shrub reaches (12%).
The observed differences between study reaches for terrestrial invertebrate contributions to juvenile salmon diet were not statistically significant. High variability in the size and frequency of terrestrial invertebrate prey observed in juvenile salmon diet contributed to the lack of significance in comparisons between study reaches. Understory vegetation within the tree reach was similar to the shrub reach and similar terrestrial invertebrate contributions were observed (21% and 22% respectively). The percent contribution of terrestrial invertebrates in the grass/sedge study reach was generally lower (15%). Prior studies have found greater terrestrial invertebrate in-fall to streams in areas of deciduous riparian shrub and tree habitat as compared to coniferous forest (Wipfli Citation1997; Allan et al. Citation2003; Inoue et al. Citation2013), pasture (Edwards and Huryn Citation1995) and grasslands (Kawaguchi and Nakano Citation2001), consequently greater observed terrestrial prey contributions from tree and shrub vegetation types as compared to grass/sedge would be expected.
Observed consumption of aquatic winged adult prey varied seasonally and aquatic winged adults represented approximately 30% of spring invertebrate consumption in the tree study reach. Seasonal consumption of Diptera of unknown origin (∼30%) was observed during spring (grass/sedge and tree study reaches) and fall (tree study reach). Increased consumption of terrestrial, aquatic winged adult and Diptera of unknown origin was generally associated with decreases in aquatic invertebrate consumption in grass/sedge and tree study reaches and most likely were the result of emergence or hatching events. Opportunities to measure invertebrate consumption associated with emergence or hatching events were constrained by the gastric evacuation rates of juvenile salmon (Allan et al. Citation2003; Sweka et al. Citation2004; Armstrong et al. Citation2013) and frequency of sampling.
Our results suggest that increased dispersal of aquatic winged adult insects and Diptera of unknown origin may reduce in-fall of those taxa as prey in open riparian habitats. Abundance of aerial insects has been found to be high in areas of open riparian habitats such as grass or clear-cut (Hetrick et al. Citation1998; Albertson et al. Citation2018); however, there is no strong correlation between aerial abundance and in-fall to streams (Hetrick et al. Citation1998; Inoue et al. Citation2013). Open areas expose insects to greater wind velocities that facilitate downwind drift and dispersal of weak-flying insects while taller riparian vegetation such as shrubs and trees can serve as habitat (Kuusela and Huusko Citation1996; Petersen et al. Citation1999; Delettre and Morvan Citation2000) that collects flying insects at stream margins (Helle and Muona Citation1985; Whitaker et al. Citation2000), and increases opportunities for in-fall (Cadwallder et al. Citation1980; Baxter et al. Citation2005; Saunders and Fausch Citation2007).
Within the Kenai watershed, late seral stage boreal forests are predicted to be replaced by persistent grasslands (Wolken et al. Citation2011; Hansen et al. Citation2016) as mean annual temperatures continue to increase, total annual precipitation decreases (Wolken et al. Citation2011; Bauret and Stuefer Citation2013; Schoen et al. Citation2017), and the frequency and intensity of disturbance events such as wildfire and insect outbreaks increase (Lynch et al. Citation2002; Klein et al. Citation2005; Berg et al. Citation2006). In addition, human development along the lower Kenai River is resulting in increased clearing within riparian areas (Schoen et al. Citation2017). While change in riparian vegetation from tree/shrub to grass/sedge can be expected to reduce contributions of cross-ecosystem invertebrates to juvenile salmon diet, research has shown that fish response to changing climate and habitat can be complex (Wainwright and Weitkamp Citation2013; Naman et al. Citation2018). Localized reduction of cross-ecosystem contributions to streams may result in reduced fish biomass in stream reaches due to movement of fish to more prey-rich areas (Kawaguchi and Nakano Citation2001; Kawaguchi et al. Citation2003) as well as increased predation of resident aquatic invertebrates (Nakano et al. Citation1999). In freshwater habitats where productivity is low, juvenile salmon are often dependent on the movement of prey resources from the terrestrial environment to streams (Allen Citation1951; Huryn Citation1996; Richardson et al. Citation2010). Widespread reduction in the availability of cross-ecosystem prey for resident fish may therefore result in reduced fish growth, fitness and density when and where fish are food-limited (Sweka and Hartman Citation2008; Fischer et al. Citation2010; Inoue et al. Citation2013). Alternatively, a shift to more open riparian canopy could raise stream temperatures, increasing in-stream production of autotrophic aquatic invertebrate prey and providing thermal habitats that promote fish growth and influence fish distribution (Hartman and Scrivener Citation1990; Armstrong and Schindler Citation2013; Tschaplinski and Pike Citation2017). In addition, increased predation risk due to lack of cover can lead to a shift in foraging behavior and diet that can result in altered growth for stream fishes (Dill and Fraser Citation1984; Reinhardt Citation1999; Allouche and Gaudin Citation2001).
While riparian areas generally represent a small portion of a total watershed, they can have a large effect on stream fish (Naiman et al. Citation2005; Wipfli and Baxter Citation2010; Wipfli and Richardson Citation2015). Our results document decreased aquatic winged adult and cross-ecosystem prey consumption that is correlated with more open riparian canopy. These results allow us to better understand how environmental or anthropogenic caused change in riparian vegetation might affect invertebrate prey contributions to juvenile salmon diet within the Kenai watershed. Monitoring change in riparian habitat using remote sensing data such as LiDAR can help us identify the extent of those changes, and management of riparian buffers along streams is likely to help mitigate potential impacts of those changes.
Acknowledgments
This study was funded by the Alaska Experimental Program to Stimulate Competitive Research (Alaska EPSCoR) NSF award #OIA-1208927 with matching funds from the state of Alaska. The authors gratefully acknowledge review and advice provided by Dr Barbara Adams, Dr Anthony (Dave) McGuire and Dr Daniel Rinella. Technicians Emily Neideigh and Christina Mielke helped with laboratory work and fieldwork throughout the project. LiDAR data were provided by the NOAA Office for Coastal Management, Kenai Watershed Forum, Kenai Peninsula Borough, University of Alaska and Alaska EPSCoR. Database of allometric juvenile salmon prey length–weight relationships is available from authors. This work was conducted under University of Alaska Fairbanks IACUC permits 720490-1 and 720490-2 and State of Alaska Department of Fish and Game fish resource permits SF2015-191 and SF2016-158. Any use of trade, firm, or product names is for descriptive purposes only and does not imply endorsement by the U.S. Government.
Disclosure statement
No potential conflict of interest was reported by the authors.
References
- Albertson LK, Ouellet V, Daniels MD. 2018. Impacts of stream riparian buffer land use on water temperature and food availability for fish. J Freshw Ecol. 33(1):195–210.
- Allan JD, Wipfli MS, Caouette JP, Prussian A, Rodgers J. 2003. Influence of streamside vegetation on inputs of terrestrial invertebrates to salmonid food webs. Can J Fish Aquat Sci. 60(3):309–320.
- Allen KR. 1951. The Horokiwi stream: a study of a trout population. Bull: New Zealand Department of Fish, 10, 231pp.
- Allouche S, Gaudin P. 2001. Effects of avian predation threat, water flow and cover on growth and habitat use by chub, Leuciscus cephalus, in an experimental stream. Oikos. 94(3):481–492.
- Armstrong JB, Schindler DE. 2013. Going with the flow: Spatial distributions of juvenile Coho Salmon track an annually shifting mosaic of water temperature. Ecosystems. 16(8):1429–1441.
- Armstrong JB, Schindler DE, Ruff CP, Brooks GT, Bentley KE, Torgersen CE. 2013. Diel horizontal migration in streams: juvenile fish exploit spatial heterogeneity in thermal and trophic resources. Ecology. 94(9):2066–2075.
- Bauret S, Stuefer S. 2013. Kenai Peninsula precipitation and air temperature trend analysis. 19th International Northern Research Basins Symposium and Workhop. Southeast Alaska. p. 35–44.
- Baxter CV, Fausch KD, Carl Saunders W. 2005. Tangled webs: reciprocal flows of invertebrate prey link streams and riparian zones: prey subsidies link stream and riparian food webs. Freshw Biol. 50(2):201–220.
- Berg EE, David Henry J, Fastie CL, De Volder AD, Matsuoka SM. 2006. Spruce beetle outbreaks on the Kenai Peninsula, Alaska, and Kluane National Park and Reserve, Yukon Territory: Relationship to summer temperatures and regional differences in disturbance regimes. Forest Ecol Manage. 227(3):219–232.
- Bohonak AJ, Jenkins DG. 2003. Ecological and evolutionary significance of dispersal by freshwater invertebrates. Ecol Lett. 6(8):783–796.
- Briers RA, Cariss HM, Gee JH. 2003. Flight activity of adult stoneflies in relation to weather. Ecol Entomol. 28(1):31–40.
- Briers RA, Cariss HM, Geoghegan R, Gee JH. 2005. The lateral extent of the subsidy from an upland stream to riparian lycosid spiders. Ecography. 28(2):165–170.
- Briers RA, Gee JH. 2004. Riparian forestry management and adult stream insects. Hydrol Earth Syst Sci. 8(3):545–549.
- Cadwallder PL, Eden AK, Hook RA. 1980. Role of Streamside Vegetation as a Food Source for Galaxias Olidus Günther (Pisces: Galaxiidae). Mar Freshw Res. 31(2):257–257–262.
- Collier KJ, Smith BJ. 1997. Dispersal of adult caddisflies (Trichoptera) into forests alongside three New Zealand streams. Hydrobiologia. 361(1/3):53–65.
- Dauwalter DC, Fesenmyer KA, Bjork R, Leasure DR, Wenger SJ. 2017. Satellite and airborne remote sensing applications for freshwater fisheries. Fisheries. 42(10):526–537.
- Delettre YR, Morvan N. 2000. Dispersal of adult aquatic Chironomidae (Diptera) in agricultural landscapes. Freshw Biol. 44(3):399–411.
- Dill LM, Fraser AHG. 1984. Risk of predation and the feeding behavior of juvenile eoho salmon (Oncorhynchuskisutch). Behav Ecol Sociobiol . 16(1):65–71.
- Edwards ED, Huryn AD. 1995. Annual contribution of terrestrial invertebrates to a New Zealand trout stream. N Z J Marine Freshw Res. 29(4):467–477.
- Fischer JR, Quist MC, Wigen SL, Schaefer AJ, Stewart TW, Isenhart TM. 2010. Assemblage and population-level responses of stream fish to riparian buffers at multiple spatial scales. Trans Amer Fish Soc. 139(1):185–200.
- Garman GC. 1991. Use of terrestrial arthropod prey by a stream-dwelling cyprinid fish. Environ Biol Fish. 30(3):325–331.
- Greenwood MJ. 2014. More than a barrier: the complex effects of ecotone vegetation type on terrestrial consumer consumption of an aquatic prey resource: Riparian Plants Alter Aquatic Prey Supply. Austral Ecol. 39(8):941–951.
- Hansen WD, Chapin FS, Naughton HT, Rupp TS, Verbyla D. 2016. Forest-landscape structure mediates effects of a spruce bark beetle (Dendroctonus rufipennis) outbreak on subsequent likelihood of burning in Alaskan boreal forest. Forest Ecol Manage. 369:38–46.
- Hartman GF, Scrivener JC. 1990. Impacts of forestry practices on a coastal stream ecosystem, Carnation Creek, British Columbia. Canad Bull Fish Aquat Sci., Department of Fisheries and Oceans, Ottawa.
- Helle P, Muona J. 1985. Invertebrate numbers in edges between clear-fellings and mature forests in northern Finland. Silva Fenn. 19(3):281–294.
- Hetrick NJ, Brusven MA, Bjornn TC, Keith RM, Meehan WR. 1998. Effects of Canopy Removal on Invertebrates and Diet of Juvenile Coho Salmon in a Small Stream in Southeast Alaska. Trans Amer Fish Soc. 127(6):876–888.
- Hunt RL, Krokhin EM. 1975. Food Relations and Behavior of Salmonid Fishes. Pages 137–156 in A. D. Hasler, editor. Coupling of land and water systems. Berlin: Springer Berlin Heidelberg.
- Huryn AD. 1996. An appraisal of the Allen paradox in a New Zealand trout stream. Limnol Oceanogr. 41(2):243–252.
- Inoue M, Sakamoto S, Kikuchi S. 2013. Terrestrial prey inputs to streams bordered by deciduous broadleaved forests, conifer plantations and clear-cut sites in southwestern Japan: effects on the abundance of red-spotted masu salmon. Ecol Freshw Fish. 22(3):335–347.
- Jackson JK, Fisher SG. 1986. Secondary production, emergence and export of aquatic insects of a Sonoran desert stream. Ecology. 67(3):629–638.
- Kawaguchi Y, Nakano S. 2001. Contribution of terrestrial invertebrates to the annual resource budget for salmonids in forest and grassland reaches of a headwater stream. Freshw Biol. 46(3):303–316.
- Kawaguchi Y, Taniguchi Y, Nakano S. 2003. Terrestrial invertebrate inputs determine the local abundance of stream fishes in a forested stream. Ecology. 84(3):701–708.2.0.CO;2]
- Klein E, Berg EE, Dial R. 2005. Wetland drying and succession across the Kenai Peninsula Lowlands, south-central Alaska. Can J Res. 35(8):1931–1941.
- Kuusela K, Huusko A. 1996. Post-emergence migration of stoneflies (Plecoptera) into the nearby forest. Ecol Entomol. 21(2):171–177.
- Lynch JA, Clark JS, Bigelow NH, Edwards ME, Finney BP. 2002. Geographic and temporal variations in fire history in boreal ecosystems of Alaska. J Geophys Res. 108(D1):801–817.
- Mason CF, Macdonald SM. 1982. The input of terrestrial invertebrates from tree canopies to a stream. Freshw Biol. 12(4):305–311.
- Merritt, R. W., and K. W. Cummins, editors. 1996. An introduction to the aquatic insects of North America, 3rd ed. Kendall/Hunt Pub. Co, Dubuque, Iowa. p. 862.
- Naiman RJ, Decamps H, McClain ME. 2005. Riparia: ecology, conservation and management of streamside communities. Burlington (MA): Elsevier Academic Press.
- Nakano S, Miyasaka H, Kuhara N. 1999. Terrestrial-aquatic linkages: riparian arthropod inputs alter trophic cascades in a stream food web. Ecology. 80(7):2435–2441.
- Naman SM, Rosenfeld JS, Kiffney PM, Richardson JS. 2018. The energetic consequences of habitat structure for forest stream salmonids. J Anim Ecol. (5):1383. 87
- Parkyn SM, Smith BJ. 2011. Dispersal constraints for stream invertebrates: setting realistic timescales for biodiversity restoration. Environ Manage. 48(3):602–614.
- Petersen I, Winterbottom JH, Orton S, Friberg N, Hildrew AG, Spiers DC, Gurney WSC. 1999. Emergence and lateral dispersal of adult Plecoptera and Trichoptera from Broadstone Stream. Freshw Biol. 42(3):401–416.
- Polis GA, Anderson WB, Holt RD. 1997. Toward an integration of landscape and food web ecology: the dynamics of spatially subsidized food webs. Annu Rev Ecol Syst. 28(1):289–316.
- Reinhardt UG. 1999. Predation risk breaks size-dependent dominance in juvenile Coho Salmon (Oncorhynchus kisutch) and provides growth opportunities for risk-prone individuals. Can J Fish Aquat Sci . 56(7):1206–1212.
- Richardson JS, Zhang Y, Marczak LB. 2010. Resource subsidies across the land-freshwater interface and responses in recipient communities. River Res Appl. 26(1):55–66.
- Ricker WE. 1973. Linear regressions in fishery research. J Fish Res Bd Can. 30(3):409–434.
- Roon DA, Wipfli MS, Kruse JJ. 2018. Riparian defoliation by the invasive green alder sawfly influences terrestrial prey subsidies to salmon streams. Ecol Freshw Fish. (4):963. 27
- Roon DA, Wipfli MS, Wurtz TL, Blanchard AL. 2016. Invasive European bird cherry (Prunus padus) reduces terrestrial prey subsidies to urban Alaskan salmon streams. Can J Fish Aquat Sci. 73(11):1679–1690.
- Saunders WC, Fausch KD. 2007. Improved Grazing Management Increases Terrestrial Invertebrate Inputs that Feed Trout in Wyoming Rangeland Streams. Trans Amer Fish Soc. 136(5):1216–1230.
- Schoen ER, Wipfli MS, Trammell EJ, Rinella DJ, Floyd AL, Grunblatt J, McCarthy MD, Meyer BE, Morton JM, Powell JE, et al. 2017. Future of Pacific Salmon in the Face of Environmental Change: Lessons from One of the World’s Remaining Productive Salmon Regions. Fisheries. 42(10):538–553.
- Sweka JA, Hartman KJ. 2008. Contribution of terrestrial invertebrates to yearly brook trout prey consumption and growth. Trans Amer Fish Soc. 137(1):224–235.
- Sweka JA, Keith Cox M, Hartman KJ. 2004. Gastric evacuation rates of brook trout. Trans Am Fish Soc. 133(1):204–210.
- Tschaplinski PJ, Pike RG. 2017. Carnation creek watershed experiment-long-term responses of Coho Salmon populations to historic forest practices: carnation creek watershed experiment. Ecohydrol. 10(2):e1812.
- Viereck LA, Dyrness CT, Batten AR, Wenzlick KJ. 1992. The Alaska vegetation classification. Portland (OR): Department of Agriculture, Forest Service, Pacific Northwest Research Station. 278p.
- Wainwright TC, Weitkamp LA. 2013. Effects of climate change on Oregon Coast Coho Salmon: habitat and life-cycle interactions. Northwest Science. 87(3):219–242.
- Whitaker DM, Carroll AL, Montevecchi WA. 2000. Elevated numbers of flying insects and insectivorous birds in riparian buffer strips. Can J Zool. 78(5):740–747.
- Winterbourn MJ, Chadderton WL, Entrekin SA, Tank JL, Harding JS. 2007. Distribution and dispersal of adult stream insects in a heterogeneous montane environment. Fund App Lim. 168(2):127–135.
- Wipfli MS. 1997. Terrestrial invertebrates as salmonid prey and nitrogen sources in streams: contrasting old-growth and young-growth riparian forests in southeastern Alaska, USA. Can J Fish Aquat Sci. 54(6):1259–1269.
- Wipfli MS, Baxter CV. 2010. Linking ecosystems, food webs, and fish production: subsidies in Salmonid watersheds. Fisheries. 35(8):373–387.
- Wipfli MS, Richardson JS. 2015. Management and the conservation of freshwater ecosystems. In G. Closs, M. Krkosek, & J. Olden (Eds.), Conservation of Freshwater Fishes (Conservation Biology, pp. 270-291). Cambridge: Cambridge University Press.
- Wolken JM, Hollingsworth TN, Rupp TS, Chapin FS, Trainor SF, Barrett TM, Sullivan PF, McGuire AD, Euskirchen ES, Hennon PE, et al. 2011. Evidence and implications of recent and projected climate change in Alaska’s forest ecosystems. Ecosphere. 2(11):art124–35.