Abstract
Non-native crayfish can have impacts on stream communities that are highly variable. A possible explanation for this variability is that crayfish are responding in a context-dependent manner to variation in environmental factors. Among the most critical environmental factors in streams is current velocity. Moving water defines lotic ecosystems and many benthic organisms, including crayfish, respond to its influence. To examine how current velocity affected the impacts of non-native Orconectes rusticus crayfish on benthic communities, we conducted a cage-enclosure experiment in a Wisconsin stream. We hypothesized that current velocities > 50 cm s−1 would decrease the effects that O. rusticus had on community composition (macroinvertebrate richness and numbers) and physical structure (sediment accumulation). Three cage enclosure treatments (open, closed crayfish and closed control) were grouped into eight replicate blocks divided equally among streambed regions of slow (< 50 cm s−1) and fast (> 50 cm s−1) current. Open cages allowed resident crayfish access, closed crayfish cages contained one O. rusticus, and closed controls had no crayfish. After three weeks, crayfish cages had lower macroinvertebrate abundance and richness and less sediment accumulation. Current velocity showed a positive relationship to macroinvertebrates in general, and a negative relationship to sediment build-up. The most common macroinvertebrates were hydropsychid caddisflies that responded to O. rusticus in a context-dependent manner such that cages containing crayfish had decreased hydropsychid numbers in fast current, but not in slow. Open cage treatments showed a similar pattern, suggesting that natural crayfish densities in the stream were also sufficient for lowering hydropsychid numbers in fast current. Our study demonstrates that current velocity may provide an important context for understanding the effects that O. rusticus have on benthic communities, not just in terms of what these crayfish do, but how the benthic communities respond to their presence.
Introduction
There is a considerable body of literature documenting the impacts crayfish, especially non-native crayfish, have on stream communities (see reviews by Phillips et al. Citation2009, Twardochleb et al. Citation2013). These impacts often have far-reaching consequences for stream structure and function and this had led many authors to classify crayfish as ecosystem engineers (Statzner et al. Citation2003, Hansen et al. Citation2013, Jackson et al. Citation2014), or alternatively, keystone consumers (Creed Citation1994, Lodge et al. Citation1994, Weinlander and Fureder Citation2016). As ecosystem engineers, crayfish foraging and burrowing can rearrange streambed substrates, affect bed topography, and influence gravel transport and sediment accumulation (Parkyn et al. Citation1997, Statzner et al. Citation2000, Statzner et al. Citation2003, Statzner and Sagnes Citation2008, Johnson et al. Citation2011, Albertson and Daniels Citation2016, Citation2018). As keystone consumers, omnivorous crayfish can have wide-ranging effects on lotic food webs by preying upon or competitively displacing benthic organisms (Charlebois and Lamberti Citation1996, Parkyn et al. Citation1997, Creed and Reed Citation2004, Bobeldyk and Lamberti Citation2010, Westhoff and Rabeni Citation2013) and by decoupling trophic cascades (Usio Citation2000, Jackson et al. Citation2014). Crayfish may also directly or indirectly affect the abundance of periphyton and algae (Hart Citation1992, Creed Citation1994, Moore et al. Citation2012), increase litter breakdown rates (Huryn and Wallace Citation1987, Schofield et al. Citation2001, Creed and Reed Citation2004, Zhang et al. Citation2004), and have negative effects on fish and amphibians (Dorn and Mittelbach Citation1999, Ilhéu et al. Citation2007).
The extent to which these impacts occur can vary among species and across habitats (Flinders and Magoulick Citation2003, Phillips et al. Citation2009, Larson and Olden Citation2010, Lodge et al. Citation2012, Jackson et al. Citation2014, Weinlander and Fureder Citation2016), and this is certainly true when comparing native and non-native species (Twardochleb et al. Citation2013, James et al. Citation2015). In their meta-analyses examining the ecological impacts of non-native crayfish on aquatic ecosystems worldwide, Twardochleb et al. (Citation2013) found that non-natives generally had greater and more negative effects than did native species on stream communities. They also noted that non-natives had more variable effects on ecological parameters. Significantly, Twardochleb et al. (Citation2013) found that differences among species were insufficient for explaining this variability and suggested that ‘extrinsic factors’ (e.g. available food resources) may be more important than species identity for determining disparities in ecological effects.
Another possible explanation for the observed discrepancies could be that crayfish in general, and non-native species in particular, are responding to variation in environmental factors (Flinders and Magoulick Citation2007, Jackson et al. Citation2017). Like many aquatic organisms, crayfish are subject to factors such as current velocity (Clark et al. Citation2008, Perry and Jones Citation2018), temperature (Gherardi et al. Citation2013, Johnson et al. Citation2014, Stoffels et al. Citation2016), substrate type (Adams and Marks Citation2016) and salinity (Bazer et al. Citation2016), among others. These factors all have the potential to affect crayfish physiology, behavior and performance (Dunson and Travis Citation1991), and this can have consequences for the ecological roles that crayfish play (Wellnitz and Poff Citation2001). For example, the ability of crayfish to effectively prey on benthic macroinvertebrates is partially dependent on their ability to navigate streambeds, and streambeds are subject to a wide range of flows and current velocities. It is easy to imagine that slower velocities would allow for greater manoeuverability and more effective predation, whereas faster velocities might constrain movement and decrease predation. This being the case, crayfish impacts on benthic invertebrates could be considered ‘context-dependent’, and this may help explain the variable effects crayfish have on the stream communities and ecosystems.
Stream current velocity is a particularly important environmental factor because moving water defines lotic ecosystems and many, if not most, stream organisms respond to its influence (Statzner et al. Citation1988, Hart and Finelli Citation1999). Current velocity can influence the distribution and abundance of benthic organisms (Hart Citation1992, Passy Citation2001, Dewson et al. Citation2007a, b), the interactions that occur between them (Hansen et al. Citation1991, Kuhara et al. Citation2000, Opsahl et al. Citation2003), and the functional contributions that they make to stream ecosystem functioning (Hintz and Wellnitz Citation2013, Poff et al. Citation2003). For crayfish in particular, current has been shown to be a key environmental variable for predicting their occurrence and habitat use in streams (Brooks et al. Citation2005, Flinders and Magoulick Citation2007, Magoulick et al. Citation2017). Current constrains crayfish movement, can affect feeding and positioning on streambeds (Maude and Williams Citation1983, Clark et al. Citation2008, Perry and Jones Citation2018), and may modify their impacts on benthic communities. For example, Hart (Citation1992) and Creed (Citation1994) demonstrated that fast current velocities > 50 cm s−1 limit the ability of native crayfish to remove filamentous Cladophora algae from the streambed. They showed Cladophora was in competition with epilithic diatoms for space, and regions of the streambed having fast current had fewer epilithic diatoms as compared to those having current < 50 cm s−1. This shift in algal dominance led to there being fewer sessile grazers that feed on diatoms. Thus, by modifying crayfish foraging on either side of this 50 cm s−1 threshold, current velocity affected the impact crayfish had on the physical and trophic structure of the benthic community.
To examine how different ranges of current velocity might affect the ecological performance of a non-native crayfish in a Wisconsin stream, we conducted a cage enclosure experiment. The species used was the invasive rusty crayfish, Orconectes rusticus, and our goal was to determine how current velocity affected its impact on benthic assemblages, using 50 cm s−1 as a cutoff between fast and slow current. We chose this velocity because other studies have shown crayfish foraging activity and food acquisition is depressed at current > 50 cm s−1 (Hart Citation1992, Creed Citation1994, Perry and Jones Citation2018). Rather than elucidate precise mechanisms by which current and O. rusticus exerted their effects, our goal here was to ascertain patterns in the macroinvertebrate response. To assess how current velocity influenced the ability of O. rusticus to structure the environment, we measured sediment accumulation, a frequently cited aspect of habitat modification by crayfish (Parkyn et al. Citation1997, Statzner and Sagnes Citation2008, Albertson and Daniels Citation2016). For both macroinvertebrates and sediment, we hypothesized that O. rusticus effects would be significantly less in fast current > 50 cm s−1.
Methods
Focal organism
The rusty crayfish, Orconectes rusticus (Girard, 1852), is native to the Ohio River basin and has been undergoing a northern range expansion into the Great Lakes region since the 1970s (Phillips et al. Citation2009). Much of this expansion has likely occurred through the bait trade and recreational fishing (Kilian et al. Citation2012, Arcella et al. Citation2014), and the spread of this invasive crayfish has significantly reduced native crayfish diversity and altered habitats in Wisconsin lakes and streams (Capelli Citation1982, Bobeldyk and Lamberti Citation2008, Olden et al. Citation2006). Rusty crayfish have been the focus of intensive management and control efforts because of their ecosystem-level impacts and persistence once established (Nilsson et al. Citation2012, Hansen et al. Citation2013). Orconectes rusticus has been shown to outcompete native crayfish, reduce macroinvertebrate and macrophyte abundances (Olden et al. Citation2006), and cause shifts in native fish populations (Wilson et al. Citation2004).
Study site
Lowes Creek is a third-order trout stream in Eau Claire County, Wisconsin, USA. The stream drains an agricultural watershed and flows 19 km north before joining the Chippewa River near the city of Eau Claire. Lowes Creek has a riparian buffer of mixed hardwood-conifer forest and passes through a forested corridor of parkland and dispersed residential development as it nears the city. Summer discharge ranges around 0.5 m3 s−1 and the average stream temperature during the August 2014 study period was 21° C. The Lowes Creek streambed is composed mostly of sand (51%), but there are areas of bedrock (19%), gravel (13%), and silt/clay (11%), and occasional patches of cobble/rubble (4%) and detritus (2%) (Spaeth et al. Citation2004).
Historically, native virile crayfish, Orconectes virilis, inhabited Lowes Creek, but O. rusticus was the only crayfish species encountered during our study. Daytime O. rusticus densities (determined with a 1-m2 quadrat sampler; DiStefano et al. Citation2003) averaged 2.3 individuals m−2 (n = 12). This is a relatively low density for O. rusticus-invaded streams (e.g. Charlebois and Lamberti Citation1996); however, O. rusticus forage primarily at night (Bergman and Moore Citation2003) so this daytime estimate likely under-represents actual stream densities.
Lowes Creek contains a diverse fish assemblage comprised of 27 species that include brook and brown trout (Salvelinus fontinalis and Salmo trutta, respectively), and gamefish such as small mouth bass (Micropterus dolomieu). A 2000 electro-shocking survey conducted by the Wisconsin Department of Natural Resources showed the dominant fish species, comprising 60% of the catch, were white suckers (Catostomus commersonii), mottled sculpin (Cottus bairdii), American brook lamprey (Lethenteron appendix), and brassy minnows (Hybognathus hankinsoni).
Our study reach (44° 46 14 N, 91° 29′ 33″ W) was approximately 60 m in length and chosen because it was relatively wide (14–20 m) and shallow (0.2–0.4 m), and because the streambed was composed almost entirely sandstone bedrock. The bedrock provided stable substrate for fastening experimental cages and the section’s width and depth made it relatively easy to find streambed locations that differed in current velocity, but were similar in depth. We assessed the variability in current across the study reach with a Marsh McBirney Flo-Mate 2000 and found current velocity ranged from 3 to 102 cm s−1 and averaged 44 cm s−1 (n = 24).
Study design
Our experiment employed a 3 × 2 factorial design for cage and current velocity treatments, respectively. Cage treatments were open, closed crayfish and closed control. Open cages were open at both ends to allow resident crayfish access; closed crayfish cages (hereafter, simply ‘crayfish’) contained a single O. rusticus crayfish and had mesh covering both ends to prevent their escape; closed control cages also had mesh over both ends but no crayfish. Current velocity treatments were maintained within two ranges, 20-30 cm s−1 and 50-70 cm s−1, hereafter referred to as ‘slow’ and ‘fast’ current.
The cylindrical cages measured 45 × 15 cm (length × diameter) and were constructed of polyethylene ‘hardware cloth’ having 20 mm mesh held together with nylon cable ties (). Each cage contained 1.75 L of landscaping gravel that covered the bottom to a depth of 5 cm. The gravel size was 3.8 ± 1.8 cm (mean ± SD) and was chosen because it was sufficiently large to not be displaced by fast current treatment, but was small enough to provide ample interstitial refugia for macroinvertebrates.
Figure 1. Photo showing a block of the three cage treatments on the streambed of Lowes Creek. Gravel substrates look clean and lack sediment build-up because the photo was taken immediately after the block was installed.
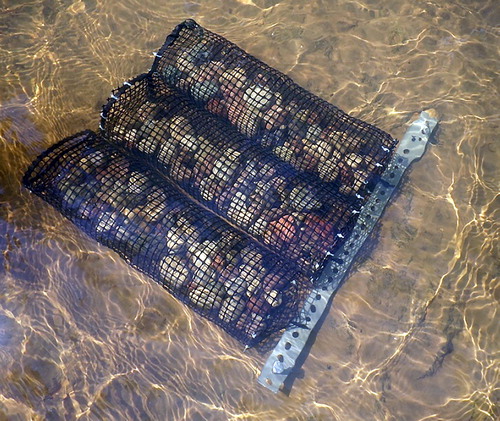
The three cage enclosure treatments (open, crayfish and closed control) were grouped into eight replicate blocks that were divided equally among regions of slow and fast current. The order of treatments within a block was randomized. The three cage treatments were held together by attaching them to a slotted steel bar staked to the streambed (). Locations of fast and slow current were found by walking the study section and measuring current velocity with the Marsh McBirney Flo-Mate 2000. Once a location was identified, the block was held in place on the streambed and the current velocities inside each cage were determined with a MiniWater20 Micro velocity probe (Schiltknecht Messtechnik AG, Zürich). This probe was small enough to insert through the hardware cloth mesh so that measurements could be taken inside cages just above the gravel substrate. Current velocity measurements were taken near the upstream, middle and downstream ends of each cage. The average near-bed current at each position was recorded for 6 s using the Schiltknecht meter ‘rep’ function. If the current velocity within a cage was outside the desired treatment range, the positioning of the cage or the block was adjusted until the appropriate current range was established. After final adjustments, the block was fixed in place by securing its slotted steel bar to the streambed with steel spikes. The cage blocks were left in place for 1 week before adding crayfish. This was done so that macroinvertebrates could begin colonizing the gravel substrates, the stability of within-cage current velocities could be assessed, and to ensure that excessive sand build-up did not occur in cages.
The experiment began on 5 August 2014 and ran three weeks. Eight large O. rusticus crayfish (CL = 25–28 mm) were hand-collected from Lowes Creeks and introduced into each crayfish cage treatment. This was done by opening a gap in the hardware cloth, slipping the crayfish through the gap, then closing it with cable ties. During the experiment, the cages were inspected every-other day to assess crayfish condition, measure within-cage current velocities, and remove accumulated debris from cage surfaces to prevent clogging.
Although our crayfish treatments contained only one individual per cage, the effective density was 14.8 individuals m−2 because of the small cage area (0.0675 m2). This density exceeded the 2.3 individuals m−2 found in Lowes Creek at the time of the experiment, but was within the range reported for other O. rusticus-invaded streams (Momot et al., Citation1978; Charlebois and Lamberti, Citation1996; Kuhlmann and Hazelton, Citation2007), and was below that used in similar cage studies (e.g. 24 crayfish m−2, Albertson and Daniels, Citation2016). The discrepancy between experimental and natural crayfish densities was acceptable because our study was designed to examine how current velocity influenced O. rusticus impacts generally rather than just in Lowes Creek.
At the end of three weeks the cages were sampled for macroinvertebrates, organic matter and sediment. Sampling was accomplished by detaching the cage from the block array, lifting it from the streambed and transferring it into a 12 L plastic tub. The cage contents were emptied into the tub and organisms clinging to the hardware cloth mesh were removed with forceps and also put into the tub. These contents were then transferred to a 3.8 L Ziploc bag and were preserved by adding 75% ethanol. Crayfish then were put into a separate container to be euthanized; a practice mandated by the state of Wisconsin for invasive crayfish.
In the laboratory, Ziploc bag contents were emptied into white enamel sorting trays. Gravel was removed, rinsed with a wash bottle, and macroinvertebrates and coarse particulate organic matter (CPOM) were removed from the trays with forceps. Macroinvertebrates were counted after being identified using Bouchard (Citation2004). Once macroinvertebrates and CPOM were removed, the remaining sediment was rinsed from the tray and filtered through glass fiber filters. CPOM and sediment samples were dried at 60 °C overnight to determine dry weights.
Data analysis
To determine the effects of current velocity and cage treatments and their interaction on macroinvertebrate numbers, we used the General Linear Model function in JMP 8.0.1 (SAS Institute Inc., Citation2009). Models used a Poisson distribution to fit variables and a log link function to create linear models. We used a full model that included main factor effects (i.e., current and cage treatment) and the interaction between them. Chi-squared (χ2) probabilities ascertained significance levels of individual factors and interactions. Pairwise tests were done with Kruskal–Wallis post hoc tests to determine which cage treatments were different. The same analysis was used to examine the effects of current and cage treatments on CPOM and sediments.
Results
Crayfish and current velocity treatments were successfully maintained over the course of the experiment. No crayfish mortality was observed and no resident crayfish were found or seen in either treatment or control cages during regular, daytime observations. Current within cages varied over the course of the experiment, but slow and fast current velocity treatments were maintained within their experimental ranges (t = −14.51, p < 0.0001). Slow current ranged 25–31 cm s−1 and fast current ranged 52–73 cm s−1. The average difference between current measured at the beginning and the end of the experiment was 8 ± 4 (SD) cm s−1.
Macroinvertebrate numbers responded to both current and cage treatments (), such that total numbers increased with current () and decreased in crayfish cages (. Macroinvertebrate richness, by comparison, responded only to cage treatments and was lowest in crayfish cages (χ2 = 10.90, df = 2, 23, p = 0.004). A total of 22 taxa were found in cages, of which eight (in decreasing order of abundance) were common: net-spinning caddisflies (Hydropsychidae, 51%), cranefly larvae (Tipulidae, 13%), midges (Chironomidae, 22%), mayflies (Batidae and Hepatgeniidae, 8%), microcaddisflies (Hydroptilidae, 2%), annelid worms (2%), snipe fly larvae (Athericidae, 1%), and blackflies (Simulium spp., 1%). The thirteen other taxa made up <1% of all macroinvertebrates.
Figure 2. The mean ± SE number of benthic invertebrates in cage treatments after 21 d in Lowes Creek. Closed cages excluded crayfish, crayfish cages had one O. rusticus, and open cages had ends removed so resident crayfish could freely enter and leave. The three graphs show total macroinvertebrate (A), hydrospychid (B), and tipulid (C) numbers. Cage treatments that were significantly different are indicated by an asterix (Kruskal-Wallis, p < 0.05).
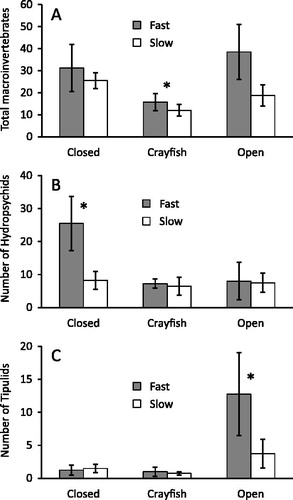
Figure 3. The relationship between stream current and all macroinvertebrates from all cages at the end of the experiment.
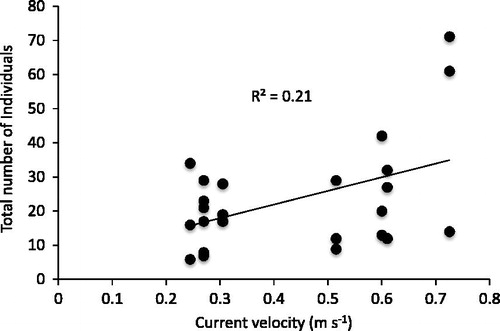
Table 1. Results of the GLMs used to test cage treatment and current velocity effects on the numbers of benthic macroinvertebrates found in cages at the end of the 21d experiment.
Net-spinning caddisflies (Hydropsychidae) were the most abundant macroinvertebrate and the only taxonomic group to respond to both current and cage treatments and the interaction between these factors (). Across current velocities, hydropsychid numbers were highest in the closed control cages and lowest in crayfish and open cage treatments (. The current X cage interaction resulted from hydropsychid numbers increasing as current velocity increased in the closed cage treatment, but not in the open or crayfish cage treatments ().
Figure 4. The interaction between crayfish treatment and current velocity on the abundance of hydropsychids in cages. Hydropsychid numbers increased with current only where O. rusticus crayfish were absent.
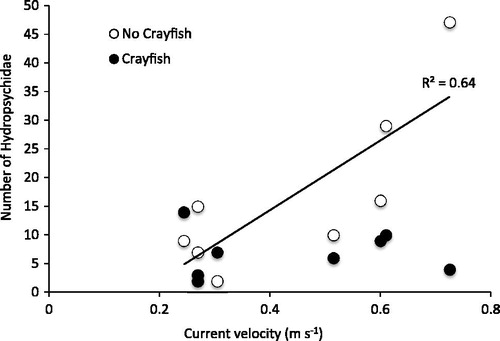
Craneflies (Tipulidae) were the only other taxon to show a response to experimental treatments (). Larval tipulids were most abundant in open cages (), and their distribution mirrored the pattern of CPOM accumulation. By the end of the experiment, CPOM in open cages was two orders of magnitude greater than that seen in other treatments (.
Figure 5. The mean g weight ± SE for CPOM (A) and sediment (B) in cage treatments after 21 d in Lowes Creek. Note CPOM data has been log-transformed. Closed cages excluded crayfish, crayfish cages had one O. rusticus, and open cages allowed resident crayfish to enter and leave freely. Cage treatments that differed significantly are indicated by an asterix (Kruskal–Wallis, p < 0.05).
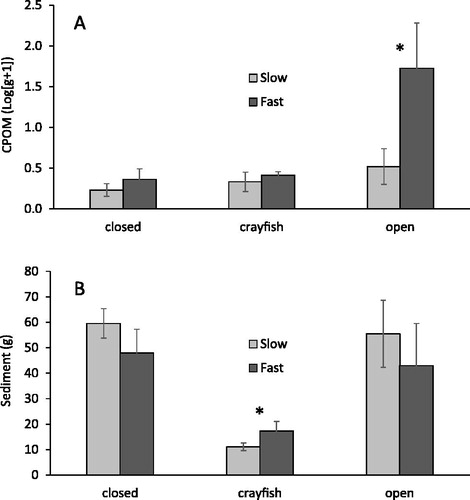
Sediment accumulation was greatest at slower velocities and lowest in crayfish cages (, . Gravel in crayfish cages looked ‘cleaner’ across the range of current velocity, but the difference was most distinct at slow velocities where differences between crayfish and non-crayfish cages were largest. The significant interaction between current and cage treatments resulted from cages with crayfish having less sediment than open cages at slow, but not fast current. Sediment accumulation did not differ between open and closed cage treatments.
Table 2. Results of the GLMs used to test cage treatment and current velocity effects on CPOM and sediment accumulation in cages.
Discussion
Current velocity and O. rusticus crayfish had divergent effects on macroinvertebrate assemblages in cages. Overall, macroinvertebrate numbers increased with current velocity and decreased in the presence of O. rusticus. Macroinvertebrate richness, by contrast, was affected only by O. rusticus and decreased. We had hypothesized that crayfish impacts would be modified by current, and while this did not occur for macroinvertebrates in general, there was an interaction between current velocity and cage treatments for the most common macroinvertebrate, hydropsychid caddisflies.
Hydropsychids showed a context-dependent response to O. rusticus such that hydropsychid numbers were reduced in fast current, but not in slow. This result met our expectation of finding current-mediated effects, but it ran counter to our hypothesis that O. rusticus impacts would decrease as current velocity increased. It is noteworthy that hydropsychid numbers were already quite low in slow current, so it would have been difficult for O. rusticus to have an effect there. Consequently, our result may not so much have been a case of O. rusticus having an impact on hydropsychids in fast over slow current, but rather, O. rusticus having an impact on hydropsychids where they were abundant. The context-dependent interaction we observed, therefore, may have been driven more by hydropsychid responses to current than current-mediated O. rusticus impacts on these caddisflies. The observed response hydropsychids showed to current velocity and crayfish may stem from their feeding ecology.
Hydropsychid larvae are filter-feeders that live in fixed retreats (i.e. immovable cases attached to substrates) and capture drifting food particles with silken nets oriented perpendicular to flow (Wiggins Citation1996). Constructed of sand grains, tiny stones and plant material held together with silk, these retreats are thought to serve two functions: to provide hydropsychids with stable scaffolding for supporting their food-capturing nets, and to serve as refugia from predators (Fuller and Rand Citation1990, Holomuzki and Van Loan Citation2002, Fairchild and Holomuzki Citation2005). Food capture rates in hydropsychid nets often correlate positively with current velocity (Georgian and Thorp Citation1992, Brooks and Haeusler Citation2016), so having stable support for feeding nets becomes critical at higher flows. In our experiment, this positive relationship between current velocity and food capture likely explains why hydropsychids became more abundant as current increased.
In terms of predator defense, fixed retreats afford some protection (Fuller and Rand Citation1990), but they also impose a sedentary lifestyle that may make hydrosphychids vulnerable to crayfish.
Weinlander and Fureder (Citation2016), for example, showed that native and non-native crayfish in Austrian streams preyed more successfully on slow-moving and sessile invertebrates than those that were agile and fast-moving. Unlike filter-feeding Simullidae, which can reposition themselves on substrates with relative ease (Lacoursière Citation1992), hydropsychids cannot move away from or escape threats without vacating their retreats, which means sacrificing the energy and resources it took to construct the retreat, to say nothing of the lost feeding time incurred before another retreat can be built.
Predation is not the only way crayfish affect macroinvertebrates. Disturbance resulting from crayfish foraging can make substrates less habitable. O. rusticus foraging could potentially damage or dislodge hydropsychid retreats, causing the larvae to abandon them, and also stir up sediments that could foul capture nets or bury larvae (Runde and Hellenthal Citation2000). Crayfish are known bioturbators and their activity can increase streambed erosion (Statzner et al. Citation2000) and disturb fine sediments and gravels (Statzner et al. Citation2003, Johnson et al. Citation2011, Harvey et al. Citation2014, Albertson and Daniels Citation2016). Our data show that crayfish cages had less sediment than non-crayfish cages, which suggests that O. rusticus activity disturbed substrates, and we believe this deterred macroinvertebrate colonization in general, and hydropsychid establishment in particular. The difference in sediment accumulation between crayfish and non-crayfish cages was greatest at slow current velocities (), which may partially explain why fewer hydropsychids were found at slow velocity, since greater mobilization of sediment may have led to more net clogging (Runde and Hellenthal Citation2000).
It should be noted that sediment removal by crayfish can also benefit benthic organisms. Albertson and Daniels (Citation2016) conducted a similar cage-enclosure experiment with O. rusticus in a Pennsylvania stream, and like us, found O. rusticus decreased sediment accumulation on gravel. Unlike us, they found that this crayfish-mediated disturbance increased macroinvertebrate abundance. These authors attributed this positive effect to the way in which sediment removal improved macroinvertebrate habitat by clearing sediments from interstitial spaces between gravel substrates, a key habitat for many macroinvertebrates (Bruno et al. Citation2012). That this did not occur in our experiment may have been due to the gravel size used. Whereas Albertson and Daniels (Citation2016) used particle ranging between 5.6 and 31 mm, our gravel particles were larger (averaging 38 mm diameter) and more homogenous. This meant the interstitial spaces in our substrates were generally larger, since there were not smaller particles to fill gaps between larger particles. Because of this gravel size difference, we speculate that interstitial clogging by sediment was less a factor in our study than it was for Albertson and Daniels (Citation2016). In the present study, we suspect the benificial effects of sediment removal by O. rusticus were countermanded by the negative effects of O. rusticus-mediated disturbance.
When comparing hydropsychid abundance across cage treatments it is interesting to note that numbers did not differ between crayfish and open cage treatments. When checking cages over the course of the experiment, we did not observe crayfish in the open cages; however, we checked them only during the day and O. rusticus forage primarily at night (Bergman and Moore Citation2003). There is evidence to suggest that naturally occurring O. rusticus did visit the open cages at night. While the total numbers of macroinvertebrates were greater in open cages compared to crayfish cages, hydropsychid numbers in open and crayfish cages were similarly low. This would make sense if crayfish visitation to the cages was sporadic. Occasional disturbance and/or predation events could have been sufficient to keep hydropsychid densities low, whereas mobile species could reoccupy cages soon after the crayfish left so their numbers would be unaffected. It should be noted that ambient crayfish densities in Lowes Creek were > 6X lower than in crayfish cage treatments (2.3 vs. 14.8 individuals m−2, respectively). Thus, if crayfish did sporadically visit open cages as we hypothesize, our data suggest that ambient crayfish densities were sufficient to reduce hydropsychid numbers.
Open cages were distinctive for another reason: they had significantly more CPOM and Tipulidae than any other treatment. CPOM biomass took the form of leaves, small twigs and detritus and was highly variable among the open cages. CPOM was more abundant in open cages because the treatment’s openings allowed drifting CPOM to enter and become entrapped inside. CPOM provides additional habitat and food resources for macroinvertebrates in general and shredders in particular (Cummins and Klug Citation1979), and this probably accounted for tipulid shredders being 5X more abundant in open cages.
Our study demonstrates that current velocity can provide an important context for understanding crayfish impacts on benthic stream communities. That crayfish can reduce the abundance of benthic organisms on streambeds is well documented (Twardochleb et al. Citation2013), but for some taxa, such as the hydropsychids, this may only occur within certain ranges of flow. We had hypothesized that O. rusticus impacts would be less at higher current velocities, but found the opposite to be true for hydropsychid caddisflies. And the impact O. rusticus had on hydropsychids appeared to be driven more by the caddisfly’s response to current than current-mediated O. rusticus effects. We did not examine underlying mechanisms, yet it is likely that multiple factors contributed to the patterns found. The hydropsychid response may have involved such factors as current-mediated food capture rates, sediment accumulation, and vulnerability to crayfish predation and/or disturbance. This study provides evidence that current velocity-dependent species impacts and responses may have relevance for assessing the influence of invasive species like O. rusticus. It remains to be seen if O. rusticus effects differ from those of native crayfish across the gradient of stream current.
Notes on contributors
Dr. Todd Wellnitz is a professor of biology at the University of Wisconsin – Eau Claire where he teaches courses in field biology, aquatic ecology and invertebrate zoology. He studies how current and flow structure stream communities and the impact that humans have on wilderness areas.
Drew Frase studied chemistry and biology at the University of Wisconsin – Eau Claire and is currently a research assistant in the Program in Gene Expression and Regulation at the Wistar Institute's Center for Chemical Biology and Translational Medicine.
Margaret Gapinski is a high school teacher at Rufus King International Baccalaureate High School in the Milwaukee Public School District. She teaches Biology and Environmental Health with a focus on environmental toxins and water quality.
Hayley Emerson Haggerty studied Ecology, Evolutionary Biology and Psychology at the University of Wisconsin – Eau Claire.
Acknowledgments
We wish to thank Andrew Dyson, Daniel Schnick and Lee Vang for their help in the lab and the field, and Lois Helland for allowing us access to Lowes Creek.
Disclosure statement
No potential conflict of interest was reported by the authors.
Additional information
Funding
References
- Adams KJ, Marks JC. 2016. Population response of the invasive crayfish Orconectes virilis (Hagen, 1870) (Decapoda: Astacoidea: Cambaridae) to restoration: what are the consequences of changes in predatory regulation and physical habitat in Fossil Creek, Arizona, USA? J Crustacean Biol. 36(5):597–606.
- Albertson LK, Daniels MD. 2016. Effects of invasive crayfish on fine sediment accumulation, gravel movement, and macroinvertebrate communities. Freshwater Sci. 35(2):644–653.
- Albertson LK, Daniels MD. 2018. Crayfish ecosystem engineering effects on riverbed disturbance and topography are mediated by size and behavior. Freshwater Sci. 37(4):836–844.
- Arcella TE, Perry WL, Lodge DM, Feder JL. 2014. The role of hybridization in a species invasion and extirpation of resident fauna: hybrid vigor and breakdown in the rusty crayfish, Orconectes rusticus. J Crustacean Biol. 34(2):157–164.
- Bazer CE, Preston RL, Perry WL. 2016. Increased salinity affects survival and osmotic response of rusty crayfish Orconectes rusticus and northern clearwater crayfish O. propinquus (Decapoda: Astacoidea: Cambaridae) as salinity increases: the potential for estuarine invasions. J Crustacean Biol. 36(5):607–614.
- Bergman DA, Moore PA. 2003. Field observations of intraspecific agonistic behavior of two crayfish species, Orconectes rusticus and Orconectes virilis, in different habitats. Biolog Bull. 205(1):26–35.
- Bobeldyk AM, Lamberti GA. 2008. A decade after invasion: Evaluating the continuing effects of rusty crayfish on a Michigan river. J Great Lakes Res. 34(2):265–275.
- Bobeldyk AM, Lamberti GA. 2010. Stream food web responses to a large omnivorous invader, Orconectes rusticus (Decapoda, Cambaridae). Crustaceana 83:641–657.
- Bouchard RW. Jr. 2004. Guide to Aquatic Invertebrates of the Upper Midwest. St. Paul, Minnesota: Water Resources Center, University of Minnesota, 208pp.
- Brooks A, Haeusler T. 2016. Invertebrate responses to flow: trait-velocity relationships during low and moderate flows. Hydrobiologia. 773(1):23–34.
- Brooks AJ, Haeusler T, Reinfelds I, Williams S. 2005. Hydraulic microhabitats and the distribution of macroinvertebrate assemblages in riffles. Freshw Biol. 50:331–344.
- Bruno MC, Bottazzi E, Rossetti G. 2012. Downward, upstream or downstream? Assessment of meio- and macrofaunal colonization patterns in a gravel-bed stream using artificial substrates. Ann Limnol - Int J Lim. 8:371–381.
- Capelli GM. 1982. Displacement of northern Wisconsin crayfish by Orconectes rusticus (Girard). Limnol Oceanogr . 27(4):741–745.
- Charlebois PM, Lamberti GA. 1996. Invading crayfish in a Michigan stream: Direct and indirect effects on periphyton and macroinvertebrates. Journal of the North American Bentholog Soc. 15(4):551–563.
- Clark JM, Kershner MW, Holomuzki JR. 2008. Grain size and sorting effects on size-dependent responses by lotic crayfish to high flows. Hydrobiologia. 610(1):55–66.
- Creed RP. 1994. Direct and indirect effects of crayfish grazing in a stream community. Ecology. 75(7):2091–2103.
- Creed RP, Reed JM. 2004. Ecosystem engineering by crayfish in a headwater stream community. J North Amer Bentholog Soc. 23(2):224–236.
- Cummins KW, Klug MJ. 1979. Feeding ecology of stream invertebrates. Annu Rev Ecol Syst. 10(1):147–172.
- Dewson ZS, James ABW, Death RG. 2007a. A review of the consequences of decreased flow for instream habitat and macroinvertebrates. J North Amer Bentholog Soc. 26(3):401–416.
- Dewson ZS, James ABW, Death RG. 2007b. Stream ecosystem functioning under reduced flow conditions. Ecolog Appl. 17(6):1797–1808.
- DiStefano RJ, Gale CM, Wagner BA, Zweifel RD. 2003. A sampling method to assess lotic crayfish communities. J Crustacean Biol. 23(3):678–690.
- Dorn NJ, Mittelbach GG. 1999. More than predator and prey: a review of interactions between fish and crayfish. Vie et Milieu. 49:229–237.
- Dunson WA, Travis J. 1991. The role of abiotic factors in community organization. Amer Natural. 138(5):1067–1091.
- Fairchild MP, Holomuzki JR. 2005. Multiple predator effects on microdistributions, survival, and drift of stream hydropsychid caddisflies. J North Amer Bentholog Soc. 24(1):101–112.
- Flinders CA, Magoulick DD. 2003. Effects of stream permanence on crayfish community structure. Amer Midland Natural. 149(1):134–147.2.0.CO;2]
- Flinders CA, Magoulick DD. 2007. Habitat use and selection within Ozark lotic crayfish assemblages: spatial and temporal variation. J Crustacean Biol. 27(2):242–254.
- Fuller RL, Rand PS. 1990. Influence of substrate type on vulnerability of prey to predaceous aquatic insects. J North Amer Bentholog Soc. 9(1):1–8.
- Georgian T, Thorp JH. 1992. Effects of microhabitat selection on feeding rates of net-spinning caddisfly larvae. Ecology. 73(1):229–240.
- Gherardi F, Coignet A, Souty‐Grosset C, Spigoli D, Aquiloni L. 2013. Climate warming and the agonistic behaviour of invasive crayfishes in Europe. Freshw Biol. 58(9):1958–1967.
- Hansen RA, Hart DD, Merz RA. 1991. Flow mediates predator-prey interactions between triclad flatworms and larval black flies. Oikos. 60(2):187–196.
- Hansen GJA, Hein CL, Roth BM, Vander Zanden MJ, Gaeta JW, Latzka AW, Carpenter SR. 2013. Food web consequences of long-term invasive crayfish control. Can J Fish Aquat Sci. 70(7):1109–1122.
- Hart DD. 1992. Community organization in streams - the importance of species interactions, physical factors, and chance. Oecologia. 91(2):220–228.
- Hart DD, Finelli CM. 1999. Physical-biological coupling in streams: The pervasive effects of flow on benthic organisms. Annu Rev Ecol Syst. 30(1):363–395.
- Harvey GL, Henshaw AJ, Moorhouse TP, Clifford NJ, Holah H, Grey J, Macdonald DW. 2014. Invasive crayfish as drivers of fine sediment dynamics in rivers: field and laboratory evidence. Earth Surf Process Landforms. 39(2):259–271.
- Hintz WD, Wellnitz T. 2013. Current velocity influences the facilitation and removal of algae by stream grazers. Aquat Ecol. 47(2):235–244.
- Holomuzki JR, Van Loan AS. 2002. Effects of structural habitat on drift distance and benthic settlement of the caddisfly, Ceratopsyche sparna. Hydrobiologia. 477(1/3):139–147.
- Huryn AD, Wallace JB. 1987. Production and litter processing by crayfish in an Appalachian mountain stream. Freshwater Biol. 18(2):277–286.
- Ilhéu M, Bernardo JM, Fernandes S. 2007. Predation of invasive crayfish on aquatic vertebrates: the effect of Procambarus clarkii on fish assemblages in Mediterranean temporary streams. In F. Gherardi (editor). Biological invaders in inland waters: profiles, distribution, and threats. Dordrecht, The Netherlands: Springer, p. 543–558
- Jackson MC, Evangelista C, Zhao T, Lecerf A, Britton R, Cucherousset J. 2017. Between-lake variation in the trophic ecology of an invasive crayfish. Freshw Biol. 62(9):1501–1510.
- Jackson MC, Jones T, Milligan M, Sheath D, Taylor J, Ellis A, England J, Grey J. 2014. Niche differentiation among invasive crayfish and their impacts on ecosystem structure and functioning. Freshw Biol. 59(6):1123–1135.
- James J, Slater FM, Vaughan IP, Young KA, Cable J. 2015. Comparing the ecological impacts of native and invasive crayfish: could native species’ translocation do more harm than good? Oecologia. 178(1):309–316.
- Johnson MF, Rice SP, Reid I. 2011. Increase in coarse sediment transport associated with disturbance of gravel river beds by signal crayfish (Pacifastacus leniusculus). Earth Surf Process Landforms. 36(12):1680–1692.
- Johnson MF, Rice SP, Reid I. 2014. The activity of signal crayfish (Pacifastacus leniusculus) in relation to thermal and hydraulic dynamics of an alluvial stream, UK. Hydrobiologia. 724(1):41–54.
- Kuhlmann ML, Hazelton PD. 2007. Invasion of the upper Susquehanna River watershed by rusty crayfish (Orconectes rusticus). Northeastern Natur. 14(4):507–518.
- Lacoursière JO. 1992. A laboratory study of fluid flow and microhabitat selection by larvae of Simulium vittatum (Diptera: Simuliidae). Can J Zool. 70(3):582–596.
- Larson ER, Olden JD. 2010. Latent extinction and invasion risk of crayfishes in the southeastern United States. Conser Biol. 24(4):1099–1110.
- Lodge DM, Kershner MW, Aloi JE, Covich AP. 1994. Effects of an omnivorous crayfish (Orconectes rusticus) on a freshwater littoral food web. Ecology. 75(5):1265–1281.
- Lodge DM, Deines A, Gherardi F, Yeo DCJ, Arcella T, Baldridge AK, Barnes MA, Chadderton WL, Feder JL, Gantz CA, et al. 2012. Global introductions of crayfishes: evaluating the impact of species invasions on ecosystem services. Annu Rev Ecol Evol Syst. 43(1):449–472.
- Magoulick DD, DiStefano RJ, Imhoff EM, Nolen MS, Wagner B. 2017. Landscape- and local-scale habitat influences on occupancy and detection probability of stream-dwelling crayfish: implications for conservation. Hydrobiologia. 799(1):217–231.
- Maude SH, Williams DD. 1983. Behavior of crayfish in water currents: hydrodynamics of eight species with reference to their distribution patterns in southern Ontario. Can J Fish Aquat Sci. 40(1):68–77.
- Momot WT, Gowing H, Jones PD. 1978. The dynamics of crayfish and their role in ecosystems. Amer Midland Natur. 99(1):10–35.
- Moore JW, Carlson SM, Twardochleb LA, Hwan JL, Fox JM, Hayes SA. 2012. Trophic tangles through time? Opposing direct and indirect effects of an invasive omnivore on stream ecosystem processes. PLoS ONE. 7(11):e50687.
- Kilian JV, Klauda RJ, Widman S, Kashiwagi M, Bourquin R, Weglein S, Schuster J. 2012. An assessment of a bait industry and angler behavior as a vector of invasive species. Biol Invasions. 14(7):1469–1481.
- Kuhara N, Nakano S, Miyasaka H. 2000. Flow mediates the competitive influence of a grazing caddisfly on mayflies. Ecolog Res. 15(2):145–152.
- Nilsson E, Solomon CT, Wilson KA, Willis TV, Larget B, Vander Zanden MJ. 2012. Effects of an invasive crayfish on trophic relationships in north-temperate lake food webs. Freshw Biol. 57(1):10–23.
- Olden JD, McCarthy JM, Maxted JT, Fetzer WW, Vander Zanden MJ. 2006. The rapid spread of rusty crayfish (Orconectes rusticus) with observations on native crayfish declines in Wisconsin (USA) over the past 130 years. Biol Invasions. 8(8):1621–1628.
- Opsahl RW, Wellnitz T, Poff NL. 2003. Current velocity and invertebrate grazing regulate stream algae: results of an in situ electrical exclusion. Hydrobiologia. 499(1/3):135–145.
- Parkyn SM, Rabeni CF, Collier KJ. 1997. Effects of crayfish (Paranephrops planifrons: Parastacidae) on in-stream processes and benthic faunas: a density manipulation experiment. NZ J Marine Freshw Res. 31(5):685–692.
- Passy SI. 2001. Spatial paradigms of lotic diatom distribution: A landscape ecology perspective. J Phycol. 37(3):370–378.
- Perry WL, Jones HM. 2018. Effects of elevated water velocity on the invasive rusty crayfish (Orconectes rusticus Girard, 1852) in a laboratory mesocosm. J Crustacean Biol. 38(1):13–22.
- Phillips ID, Vinebrooke RD, Turner MA. 2009. Ecosystem consequences of potential range expansions of Orconectes virilis and Orconectes rusticus crayfish in Canada – a review. Environ Rev. 17(NA):235–248.
- Poff NL, Wellnitz T, Monroe JB. 2003. Redundancy among three herbivorous insects across an experimental current velocity gradient. Oecologia. 134(2):262–269.
- Runde JM, Hellenthal RA. 2000. Behavioral responses of Hydropsyche sparna (Trichoptera: Hydropsychidae) and related species to deposited bedload sediment. Environ Entomol. 29(4):704–709.
- SAS Institute Inc. 2009. JMP 8.0.2. Cary, NC: SAS Institute Inc.
- Schofield KA, Pringle CM, Meyer JL, Sutherland AB. 2001. The importance of crayfish in the breakdown of rhododendron leaf litter. Freshw Biol. 46(9):1191–1204.
- Spaeth B, M, Washebek M, Marshall J, Pierce J. 2004. Lowes Creek monitoring report. Fall Creek, WI: Beaver Creek Citizen Science Center, 8pp.
- Statzner B, Fievet E, Champagne JY, Morel R, Herouin E. 2000. Crayfish as geomorphic agents and ecosystem engineers: Biological behavior affects sand and gravel erosion in experimental streams. Limnol Oceanogr. 45(5):1030–1040.
- Statzner B, Gore JA, Resh VH. 1988. Hydraulic stream ecology: observed patterns and potential applications. J North Amer Bentholog Soc. 7(4):307–360.
- Statzner B, Peltret O, Tomanova S. 2003. Crayfish as geomorphic agents and ecosystem engineers: effect of a biomass gradient on baseflow and flood-induced transport of gravel and sand in experimental streams. Freshw Biol. 48(1):147–163.
- Statzner B, Sagnes P. 2008. Crayfish and fish as bioturbators of streambed sediments: assessing joint effects of species with different mechanistic abilities. Geomorphology. 92:267–287.
- Stoffels RJ, Richardson AJ, Vogel MT, Coates SP, Müller WJ. 2016. What do metabolic rates tell us about thermal niches? Mechanisms driving crayfish distributions along an altitudinal gradient. Oecologia. 180(1):45–54.
- Twardochleb LA, Olden JD, Larson ER. 2013. A global meta-analysis of the ecological impacts of nonnative crayfish. Freshw Sci. 32(4):1367–1382.
- Usio N. 2000. Effects of crayfish on leaf processing and invertebrate colonisation of leaves in a headwater stream: decoupling of a trophic cascade. Oecologia. 124(4):608–614.
- Weinlander M, Fureder L. 2016. Native and alien crayfish species: do their trophic roles differ?. Freshw Sci. 35:1340–1353.
- Wellnitz T, Poff NL. 2001. Functional redundancy in heterogeneous environments: implications for conservation. Ecol Lett. 4(3):177–179.
- Westhoff JT, Rabeni CF. 2013. Resource selection and space use of a native and an invasive crayfish: evidence for competitive exclusion? Freshw Sci. 32(4):1383–1397.
- Wiggins GB. 1996. Larvae of the North American caddisfly. 2nd ed. Toronto: University of Toronto Press Incorporated.
- Wilson KA, Magnuson JJ, Lodge DM, Hill AM, Kratz TK, Perry WL, Willis TV. 2004. A long-term rusty crayfish (Orconectes rusticus) invasion: dispersal patterns and community change in a north temperate lake. Can J Fish Aquat Sci. 61(11):2255–2266.
- Zhang Y, Richardson JS, Negishi JN. 2004. Detritus processing, ecosystem engineering and benthic diversity: a test of predator–omnivore interference. J Anim Ecology. 73(4):756–766.