Abstract
The hydrological regimes of many freshwater lakes have been dramatically changed by human activity. Nevertheless, few studies have reported the effects of human water management on the formation mechanisms of lakeshore plant communities. In this study, we investigated the effects of flooding and soil nutrients on the plant diversity of lakeshore Carex communities fringing a pristine lake (Poyang Lake) and a human managed lake (Shengjin Lake), two adjacent floodplain lakes in the middle and lower reaches of the Yangtze River. Over time, the Carex meadow at Shengjin Lake experienced a lower flooding frequency and a smaller amplitude of water level fluctuations in comparison with those obtained at Poyang Lake, but similar flooding durations were found for both sites. Soil total nitrogen (TN), total phosphorus (TP) and the ratio of TN to TP in study plots at Shengjin Lake tended to be higher than those in the plots at Poyang Lake at the 0–100 cm soil depth except for soil TP at the 0–10 cm soil depth. A total of 23 plant species were recorded in the Carex communities at the two lakes. Artificial water management increased plant diversity but had no effect on plant biomass in the Carex community. The primary factor in determining plant diversity changed from the flooding regime to the concentrations of soil nutrients as the water regime underwent management. Flooding regimes had strong direct impacts on the plant communities, while imposing few indirect effects on plants by changing soil nutrients in the pristine lake. Our results represent essential information for enhancing the robustness of management efforts for these large and important freshwater lakes.
Introduction
Freshwater lakes represent one of the most important global ecosystems (Carpenter et al. Citation2011), providing a wide variety of ecological and economic services: mitigating floods, sequestrating carbon, purifying water and maintaining biodiversity (Dudgeon et al. Citation2006; Tranvik et al. Citation2009; Woodward Citation2009; Strayer and Dudgeon Citation2010). Lakeshore plant communities, as the primary producers of the system, profoundly affect the structural and functional stability of lake ecosystems (Strayer and Findlay Citation2010; Lan et al. Citation2019). Studying the formation mechanisms of lakeshore plant communities has been a central issue in wetland ecosystem for decades, providing important theoretical solutions for lake conservation (Keddy and Reznicek Citation1986; Abrahams Citation2008; Yuan et al. Citation2019).
Theoretical and experimental evidence suggest that plant diversity in lakeshore communities may often be controlled by multiple factors. Flooding regimes are the most often discussed disturbance, greatly impacting plant patterns in seasonally flooded lakeshore wetlands (Riis and Hawes Citation2002; Wilcox and Nichols Citation2008; Xu et al. Citation2015; Lan et al. Citation2019). Flood pulses in lakeshore wetlands can cause the plant habitat to shift seasonally from terrestrial to aquatic, modifying soil redox status, soil oxygen concentration and plant light availability, and thus promoting or inhibiting plant germination, growth and reproduction (Blom Citation1999; Colmer and Voesenek Citation2009; Li et al. Citation2019; Zhang et al. Citation2019). Further, edaphic characteristics are also fundamental drivers of lakeshore plant communities (Wang et al. Citation2014; Chen et al. Citation2015). As the physical foundation for various plants, soil is the principal source of nutrients for lakeshore plants, as well as the major medium for material cycling in lakeshore wetland (Beumer et al. Citation2008; Wang et al. Citation2014; Chen et al. Citation2015). To complicate matters further, the effects of flooding regimes and edaphic characteristics on plant communities are not independent. Variations in flooding regimes may create gradients in edaphic variables by controlling sediment deposition, soil aeration and associated physical and chemical reactions (Amorim and Batalha Citation2007; Lan et al. Citation2019). Meanwhile, improvement of edaphic characteristics may facilitate the acclimation of lakeshore plants to flooding. However, until now, few studies have clearly reported the architecture of the direct and indirect effects of flooding regimes and edaphic characteristics on plant communities in lakeshore wetlands.
The relative importance of flooding regimes and edaphic characteristics to plant communities in wetlands is environment dependent. Previous studies have acknowledged that the effects of flooding regimes and edaphic characteristics on wetland plant diversity often rely on spatial scales, as well as the functional groups under study (Xu et al. Citation2015; Liu et al. Citation2018; Lan et al. Citation2019). However, we know little about the effects of human water management on the formation mechanisms of the lakeshore plant community. The water regimes of freshwater lakes were altered dramatically in the twentieth century by levee construction with the goals of flood control and irrigation (Zhang et al. Citation2014a). In seasonally flooded littoral wetlands, artificial water management has always meant divergent patterns in water level fluctuation (WLF). In fact, Zhang et al. (Citation2015) and Yuan et al. (Citation2017) found that changes in water management style could significantly modify lakeshore plant communities in floodplain lakes in the middle and lower reaches of the Yangtze river. Therefore, the causal relationships among flooding regime, soil characteristics and plant diversity might be divergent under different water management scenarios. Further research on the formation mechanisms of lakeshore plant diversity around lakes with different water management patterns would be very helpful in understanding the shifts of lakeshore plant communities in a changing world.
The middle-lower Yangtze Plain (MLYP) is a lake rich region containing as many as 651 lakes with areas >1 km2 (Qin Citation2002). Historically, nearly all these floodplain lakes could freely connect with the Yangtze River, but most of these natural river to lake connections were cut off in the late twentieth century by sluices to avoid flooding and to maintain irrigation (Zhang et al. Citation2015; Yuan et al. Citation2019). Interestingly, scientists have intensively studied the structure and determining factors of the lakeshore plant communities in the few pristine lakes in the MLYP, such as Poyang Lake and Dongting Lake (Chen et al. Citation2015; Xu et al. Citation2015; Duan et al. Citation2018; Fu et al. Citation2018; Hu et al. Citation2018; Lan et al. Citation2019), but few studies have investigated the formation mechanisms of lakeshore plant communities in the human managed lakes representing the majority of lakes in the MLYP (Zhao et al. Citation2012; Zhang et al. Citation2014b, Citation2015).
In this study, we investigated the effects of the flooding regimes and soil nutrients on the plant diversity of the Carex communities in the lakeshore wetlands surrounding a pristine lake (Poyang Lake) and a human managed lake (Shengjin Lake) in the MLYP. We hypothesized that (1) the plant diversity of the Carex community would be higher in the human managed lake than in the pristine lake; (2) the flooding regime is the main factor in the development of the lakeshore plant communities in the two lakes; (3) the flooding regime can not only directly affect plant community but can also indirectly impact the plant communities of the two lakes by affecting soil nutrients.
Methods and materials
Study area
This study was conducted at Poyang Lake and Shengjin Lake, both located in the MLYP (). They are very close, separated by a linear distance of approximately 150 km. The MLYP features a typical subtropical monsoon climate. Poyang Lake is the largest freshwater lake in China and has an area of approximately 3283 km2. It has an average annual temperature of 17.90 °C and an average annual precipitation of 1617 mm. Shengjin Lake covers an area of approximately 135 km2 and has an average annual temperature of 16.14 °C and an average annual precipitation of 1600 mm. Wet season (May–September) and dry season (October–April) conditions differ significantly for both lakes on an annual scale (). The Carex community is the dominant plant community in the lakeshore wetlands of both lakes in the dry season (Hu et al. Citation2010). The dominant plant species in Carex community is Carex cinerascens. Other common species includes Phalaris arundinacea, Cardamine lyrata, Polygonum criopolitanum, and Callitriche palustris. The Carex community has two growing seasons per year, fall and spring. In the flooding season, all above ground plant tissue in this community dies in both lakes, leaving bare soil for the next dry season. Historically, the two lakes freely connected with the Yangtze River. Now, Poyang Lake can still directly connect to the Yangtze River and, thus maintains its natural WLFs. In contrast, the water level at Shengjin Lake is manually controlled by means of a sluice established in 1965 at the junction between the lake and the Yangtze River.
Field investigation and sampling
The field investigation for both lakes was performed during the fall growing season in November 2017. The investigation site at Poyang Lake is called East Lake and is located in the Nanji Wetland National Nature Reserve. It is a sublake of Poyang Lake in the lake’s southwest corner and covers an area of approximately 62 km2 (). Nearly all the lakeshore wetlands in East Lake are covered by Carex meadow. We selected a typical Carex littoral wetland approximately 1.2 km in length on the shore of East Lake. Five transects perpendicular to the lake shoreline were established. These transects stretched from the upper to the lower limits of the lakeshore Carex meadow. Three 1 × 1 m plots were set up along each transect and the distance between two adjacent plots was approximately 400 m. The investigation sites at Shengjin Lake were located at Shengjin Lake National Nature Reserve. Considering the dispersed distribution of the Carex community at Shengjin Lake, we built 9 transects perpendicular to the shoreline. Along each transect, we set up 1–3 plots (1 × 1 m), depending on variation in the condition of the vegetation. A total of 30 plots were established in the Carex wetlands of the two lakes. Each lake had 15 plots. The geographical information for each plot in both lakes was recorded using a hand-held GPS. The relative elevation above the water surface of each plot at Shengjin Lake was measured using a level instrument.
At each plot, we first identified all plant species in the community and recorded the total coverage of plants and the coverage of each species. Then, we removed and measured all above ground biomass and collected 3 root cores (0–20 cm in depth) using a 10 cm diameter root auger. Soil was washed away from the root samples using running water in the field. The soil samples at each plot were collected from six depths: 0–10 cm, 10–20 cm, 20–30 cm, 30–50 cm, 50–70 cm and 70–100 cm. Three soil sample cores were collected along the diagonal at each depth with a 3.5 cm diameter soil auger and were completely mixed into one composite soil sample. Soil and plant samples were transported to the laboratory as soon as possible for further analysis.
Laboratory analyses
We measured several physical and chemical variables at the laboratory using the collected soil and plant samples. The above ground and below ground plant samples from each plot were oven-dried at 70 °C for 48 h. Soil samples were air-dried, ground and passed through a 2 mm sieve. To determine soil nutrients, an approximately 10 g subsample of each soil sample was further ground to pass through a 100 mesh sieve. Soil total nitrogen (TN) was measured by means of the Kjeldahl digestion method using an Alpkem autoanalyzer (Kjektec System 1026 Distilling Unit, Sweden). Soil total phosphorus (TP) was measured by means of the molybdenum blue colorimetric method using a UV/visible spectrophotometer (Beckman Coulter DU 800, USA) (Bao Citation2000; Lan et al. Citation2019). The soil N/P ratio (RNP) was calculated as the ratio of TN to TP.
Data analyses
We determined plant above ground biomass (AGB) and below ground biomass (BGB), plant species richness (N), and the Shannon–Wiener index (H) for each plot at Poyang Lake (Np and Hp) and at Shengjin Lake (Ns and Hs). AGB and BGB equaled the dry weights of above ground and below ground plant biomass per square meter. Considering that all above ground plant biomass grew during the new dry season, we used the AGB value to represent above ground plant productivity in the Carex community. Higher AGB always meant higher above ground plant productivity. Plant species richness was estimated as the number of species per square meter. Plant cover data were used to calculate the Shannon–Wiener index (Antheunisse and Verhoeven Citation2008).
It is widely acknowledged that a water regime has five components: magnitude, frequency, duration, timing and rate of change (Poff et al. Citation1997). Considering the local spatial scale in the littoral wetland, we used flooding duration (FD), flooding frequency (FF) and the amplitude of WLF (WD) to characterize flooding regimes (Tan et al. Citation2016). The FD, FF and WD of each plot were calculated by comparing the elevation with daily hydrological data (Zhang et al. Citation2015; Hu et al. Citation2018) (). FD was calculated as the sum of days that the plot was submerged, FF was the number of times that the plot was submerged, and WD was calculated as the difference between highest water level and the elevation within a calendar year. The hydrological data for Poyang Lake (Xinzi hydrological station) and Shengjin Lake (Huangpengzha hydrological station) during 2014–2017 were obtained from the websites of the Water Resources Department of Jiangxi Province and the Hydrographic Bureau of Anhui. The absolute elevations of the plots at Poyang Lake were acquired from a high resolution digital elevation model of Poyang Lake produced by the Surveying and Mapping Institute of Jiangxi Province. Those values at Shengjin Lake were extrapolated from the water level and the measured relative elevations. Using all the above data, we calculated the FD, FF and WD in 2017 (FD2017, FF2017, WD2017) and the long term average FD, FF and WD during 2014–2017 (FDL, FFL, WDL) for both lakes.
One-way ANOVA was used to determine the differences in flooding regime, soil nutrients and plant variables between the two lakes. The relationships among flooding, soil nutrients and plant variables for each lake were investigated by using the bivariate correlation analysis. Structural equation modelling (SEM) was used to analyze the formation mechanisms of plant diversity in the lakeshore wetlands. Following standard SEM approaches, we developed a concept model based on the expected causal relationships among flooding variables, soil nutrients variables, plant biomass variables, and plant diversity variables (Figure S1). Unidirectional arrows represented causal pathways (regressions), while bidirectional arrows stood for unanalyzed relationships (correlations). In the concept model, we considered various direct and indirect effects of flooding variables and soil nutrient variables. Flooding variables could directly affect plant diversity and could indirectly affect plant diversity through changing soil nutrient variables or plant biomass variables. Soil nutrient variables could directly affect plant diversity and could indirectly affect plant diversity through changing plant biomass variables. Because many variables in each functional group are highly correlated, we used principal component analysis (PCA) to create multivariate functional indices (principal components) for each lake to reduce the number of variables in the final model (Chen et al. Citation2018; Lan et al. Citation2019). The flooding variable (FL) in the SEM analysis was the first principal components (PC1) of FD2017, FF2017, WD2017, FDL, FFL and WDL. Soil nutrient variables included soil nutrient concentration and soil nutrient ratio. The soil nutrient concentration variable in the SEM analyses was the PC1 of soil TN and TP (CNP). The soil nutrient ratio variable was the ratio of soil N/P ratio (RNP). The PC1 of flooding variables and soil nutrient concentration variables explained greater than 80% of the total variance at the two lakes. Soil nutrient variables at the 0–10 cm soil depth were used in the final models because these models could achieve the best fit indices. Plant biomass variables included AGB and BGB. We used the Shannon–Wiener index as our measure of plant species diversity. All statistical analyses were performed using SPSS 22 and AMOS 21 (SPSS Inc., Chicago, USA).
Results
Flooding regime
Seasonal water level dynamics produced similar one-peak flooding patterns at Poyang Lake and Shengjin Lake, which were higher in summer and lower in winter (). However, due to the different water management styles, significant differences in some key features of flooding regimes were observed in these two lakes. In 2017, the magnitude of WLF at Shengjin Lake was 6.68 m, much lower than that at Poyang Lake (13.09 m). The FD2017, FF2017 and WD2017 in the Shengjin Lake plots were averaged at 76 days, 1.40 and 2.68 m, respectively (). Comparatively, the FD2017, FF2017 and WD2017 in the Poyang Lake plots were all significantly higher, as their means were 115 days, 2.33 and 6.32 m, respectively (). When considering the water levels in 2014–2017, the average magnitude of WLF at Shengjin Lake (7.37 m) was lower than that at Poyang Lake (12.14 m). The FFL and WDL in the Shengjin Lake plots were 12.63% and 37.68% lower than those in the Poyang Lake plots (), while there was no significant difference in the FDL between the Poyang Lake plots (114–137 days) and the Shengjin Lake plots (87–152 days) (p = 0.319). However, the spatial variances of FDL, FFL and WDL in the Shengjin Lake plots were higher than those in the Poyang Lake plots. The coefficients of variation (CV) of FDL, FFL and WDL in the Poyang Lake plots were 6.28%, 7.70% and 5.08%, respectively. Comparatively, the CVs of FDL, FFL and WDL in the Shengjin Lake plots were 16.00%, 21.46% and 11.85%, respectively.
Table 1. Differences in flooding regimes and plant properties in the Carex communities at Poyang Lake and Shengjin Lake.
Plant diversity and biomass
A total of 23 plant species was recorded in the sampling sites of the two lakes (Table S1). Plant species richness and Shannon–Wiener indices in the Shengjin Lake plots were significantly higher than those in the Poyang Lake plots (). All 23 plant species were found in the Shengjin Lake plots, while only 8 species were found in the Poyang Lake plots. Np varied between 1 and 4 with a mean of 2.60, but Ns varied between 2 and 7 with an average of 4.67 (). Hp ranged from 0.00 to 0.69, with an average of 0.38 and a CV of 68.45%. Hs ranged from 0.05 to 1.37, with an average of 0.68 and a CV of 64.00% (). No significant difference was found in AGB or BGB between Poyang Lake and Shengjin Lake (). At Poyang Lake, the AGB ranged from 153.34 g/m2 to 609.74 g/m2, with an average of 368.27 g/m2 and a CV of 35.91%; the BGB ranged from 435.89 g/m2 to 3078.12 g/m2, with an average of 1626.07 g/m2 and a CV of 46.95%. In Shengjin Lake, the AGB ranged from 218.36 g/m2 to 596.73 g/m2, with an average of 415.61 g/m2 and a CV of 28.28%; the BGB ranged from 1177.36 g/m2 to 2145.75 g/m2, with an average of 1631.55 g/m2 and a CV of 21.78%.
Soil nutrients
Soil TN, TP and RNP all decreased with increasing soil depth in both the Poyang Lake and Shengjin Lake plots (). At a depth of 0–10 cm, there was no significant difference for soil TN between Poyang and Shengjin Lakes (p = 0.876) (). At depths of 10–100 cm, soil TN was significantly higher in the Shengjin Lake plots compared with those in the Poyang Lake plots (all p < 0.001). In comparison, soil TP exhibited a more complex relationship between the plots of the two lakes (). At a depth of 0–10 cm, soil TP was lower in the Shengjin Lake plots compared with Poyang Lake plots (p = 0.015). At a depth of 10–20 cm, no significant difference was found in soil TP between the two lakes (p = 0.436). At depths deeper than 20 cm, soil TP tended to be higher in the Shengjin Lake plots compared with Poyang Lake plots; however, significant differences between the lakes were found only at soil depths of 20–30 cm (p < 0.001) and 50–70 cm (p = 0.029) (). Soil RNP in the Poyang Lake plots was significantly lower than that in the Shengjin Lake plots at all soil depths (all p < 0.001) ().
SEM analyses
Most of the variables or categories that were examined in this study were correlated with each other, making this dataset particularly well suited for SEM analyses (Table S2 and ). At Poyang Lake, plant diversity was negatively correlated with plant biomass, and positively correlated with FL and CNP (Table S2). At Shengjin Lake, plant diversity was significantly and negatively correlated only with AGB and CNP (Table S2).
SEM analyses performed separately for each lake support the conclusion that artificial water management can significantly change the formation mechanisms of plant diversity in lakeshore wetlands (). In the model for Poyang Lake (χ2 = 0.220, df = 1, p = 0.639), flooding, soil nutrients and plant biomass explained 89% of the variance in plant diversity (). According to the magnitudes of the standardized path coefficients, plant diversity at Poyang Lake was most sensitive to FL. Higher plant diversities among the plots at Poyang Lake were associated with higher FL and RNP and lower BGB. FL primarily directly affected plant diversity rather than indirectly impacting plant diversity through changing soil nutrients (). In the model for Shengjin Lake (χ2 = 0.364, df = 1, p = 0.546), flooding, soil nutrients and plant biomass explained 70% of the variance in plant diversity (). Plant diversity at Shengjin Lake was most sensitive to CNP. Higher plant diversities among the plots at Shengjin Lake were associated with higher CNP, and lower BGB ().
Figure 4. The SEM analyses at Poyang Lake (A) and Shengjin Lake (B). PD: Plant diversity; AGB: aboveground biomass; BGB: belowground biomass; FL: flooding variable; CNP: soil nutrient concentration variable; RNP: the ratio of soil total nitrogen to total phosphorus. Numbers at arrows are standardized path coefficients (equivalent to correlation coefficients). Solid and dashed arrows indicate significant positive and negative relationships, respectively (p < 0.05). Numbers close to endogenous variables represented the variance explained by the model.
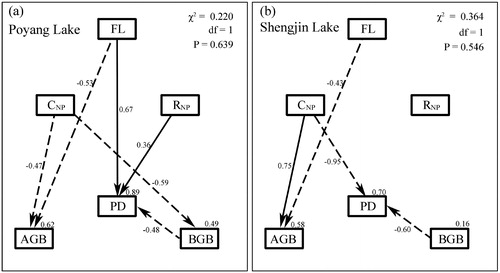
Discussion
This study provides evidence that artificial water management has been very important in shaping the formation mechanisms of plant diversity in lakeshore wetlands in the MLYP. Carex communities in the artificial managed lake have much higher plant diversity than those in the pristine lake. Flooding regime, soil nutrients and plant biomass collectively explain greater than 70% of the spatial variance in plant diversity in lakeshore Carex communities at both lakes, but the dominant determining factors for plant diversity shifted from the flooding regimes to soil nutrients as the lake water regime was artificially managed. Our results are great useful in managing the vast lakes of the MLYP. They suggest that artificial water management diminishes the area of lakeshore wetlands and increases the risk of invasion by mesophytes into the original habitats for hygrophytes. In addition, human water management increases the sensitivity of the lakeshore plant community to eutrophication, one of the most important threats to the freshwater lakes of the MLYP (Le et al. Citation2010; Wang et al. Citation2016).
The finding that plant diversity at Shengjin Lake was much higher than that at Poyang Lake is consistent with our first hypothesis and could be explained by means of the intermediate disturbance hypothesis (Hobbs and Huenneke Citation1992; Roxburgh et al. Citation2004) (). Specifically, the moderate WLF at Shengjin Lake that was induced by human water management can be seen as an intermediate disturbance, strengthening the niche heterogeneity, decreasing interspecific competition and thus increasing plant diversity (Pollock et al. Citation1998; Zhang et al. Citation2015). At Poyang Lake, the large WLF implies very strong flooding disturbance, thus limiting the establishment of many mesophytes and hygrophytes, but provides suitable habitats for the dominant species, C. cinerascens, which is well adapted to the naturally fluctuated environment in the MLYP (Yuan et al. Citation2017; Zhou et al. Citation2018). Similarly, earlier studies in the MLYP, such as Zhang et al. (Citation2015) and Yuan et al. (Citation2017), confirmed that plant richness in the human managed lakes with intermediate amplitudes of WLF was higher than that in the natural lakes.
The finding that the flooding variable had a positive relationship with plant diversity at Poyang Lake is consistent with our second hypothesis and is in accordance with the studies of Lan et al. (Citation2019) and Li et al. (Citation2018), which were carried out in two pristine lakes in the MLYP (Poyang Lake and Dongting Lake). However, the finding that the flooding variable had no significant relationship with plant diversity at Shengjin Lake contradicts the second hypothesis. These contrasting conclusions might result from the different landscape morphologies of the typical Carex meadows at these two lakes (Yuan et al. Citation2019). Due to the large WLF, the lakeshore wetland at Poyang Lake often is a very gentle slope. Although they can be visually very long (>1 km), most of the typical Carex wetlands at Poyang Lake still cannot cover all the hydrologically suitable habitats for hygrophytes due to the complicated wetland landscape. The typical Carex wetlands at Poyang Lake mainly cover the lower part of the suitable habitat for hygrophytes, where C. cinerascens faces strong flooding stress (Tan et al. Citation2016; Yuan et al. Citation2017). At sampling sites at higher elevation with short flooding durations, favorable environmental conditions lead to the accumulation of the biomass of C. cinerascens, in turn leading to competitive exclusion. At sampling sites at lower elevations with long flooding durations, helophytes with strong adaptations to flooding stress take advantage of the available ecological niches when the growth of C. cinerascens is depressed (Zhou et al. Citation2018). Indeed, the 1.2 km long transects at Poyang Lake in this study had an elevation difference of less than 1 m. The sampling sites at higher elevations always had only C. cinerascens, while the sampling sites at the lower elevations could have 2–3 helophyte or hygrophyte species besides C. cinerascens. On the other hand, due to the low WLF, the littoral wetlands at Shengjin Lake are very short and steep. Many hygrophytes or helophytes, even mesophytes, can randomly exist in the Carex community, leading to a weak relationship between plant community and flooding duration. In fact, the short Carex meadows at Shengjin Lake had relatively higher elevation differences (1.32 m in this study). The typical Carex meadows at Shengjin Lake had higher spatial variance in the flooding regime than those at Poyang Lake.
Our results show that the flooding regime impacts plant diversity mainly through direct effects rather than indirect effects in the littoral wetlands at Poyang Lake. This result contradicted our third hypothesis. The complicated relationships between flooding and soil biogeochemical cycles in the lakeshore wetlands might be one possible explanation for our results. For example, the flooding regime could create a soil nutrient gradient in the littoral wetland by determining sediment deposition but could eliminate the gradient by driving sediment lateral transportation. However, this result by no means suggests that soil nutrients had no significant effects on plant diversity in the lakeshore wetland in the MLYP. In fact, soil nutrients had stronger effects on lakeshore plant diversity than the flooding regime at Shengjin Lake. The negative effects of soil nutrients on plant diversity at Shengjin Lake were in full agreement with the results of previous fertilization experiments all over the world (Bedford et al. Citation1999; Baer et al. Citation2003; DeMalach Citation2018). The productivity of most natural ecosystems is limited by one or multiple nutrient elements. An increase in soil nutrients may directly mitigate the limitation of plant biomass by these nutrients, thereby stimulating plant biomass (Baer et al. Citation2003; Ren et al. Citation2010). However, the richness of soil nutrients cannot benefit all plant species equally. Some plants that grow rapidly and have tall branches, such as C. cinerascens, might quickly use soil nutrients and eliminate other plant species via aboveground (light limitation) or belowground competition (Suding et al. Citation2005; DeMalach et al. Citation2017).
Notes on contributors
Ruichang Shen is a ecosystem ecologist who works on lakeshore wetland ecosystem.
Zhichun Lan is a wetland ecologist who works on plant community in the lakeshore wetland.
Yasong Chen is a postgraduate student who works on plant community in the lakeshore wetland.
Fei Leng is a volunteer from UNDP-GEF Anhui Wetland Conservation Project.
Binsong Jin is a ecologist who work on lake ecosystem.
Changming Fang is a senior scientist in Fudan university who works on ecosystem ecology.
Jiakuan Chen is a senior scientist who works on biodiversity science.
Supplemental Material
Download MS Word (196.1 KB)Disclosure statement
No potential conflict of interest was reported by the authors.
Additional information
Funding
References
- Abrahams C. 2008. Climate change and lakeshore conservation: a model and review of management techniques. Hydrobiologia. 613(1):33–43.
- Amorim PK, Batalha MA. 2007. Soil-vegetation relationships in hyperseasonal cerrado, seasonal cerrado, and wet grassland in Emas National Park (central Brazil). Acta Oecol. 32(3):319–327.
- Antheunisse AM, Verhoeven JTA. 2008. Short-term responses of soil nutrient dynamics and herbaceous riverine plant communities to summer inundation. Wetlands. 28(1):232–244.
- Baer SG, Blair JM, Collins SL, Knapp AK. 2003. Soil resources regulate productivity and diversity in newly established tallgrass prairie. Ecology. 84(3):724–735.
- Bao S. 2000. Soil agro-chemistrical analysis. Beijing: China Agriculture Press.
- Bedford BL, Walbridge MR, Aldous A. 1999. Patterns in nutrient availability and plant diversity of temperate North American wetlands. Ecology. 80(7):2151–2169.
- Beumer V, van Wirdum G, Beltman B, Griffioen J, Grootjans AP, Verhoeven JTA. 2008. Geochemistry and flooding as determining factors of plant species composition in Dutch winter-flooded riverine grasslands. Sci Total Environ. 402(1):70–81.
- Blom C. 1999. Adaptations to flooding stress: from plant community to molecule. Plant Biol. 1(3):261–273.
- Carpenter SR, Stanley EH, Vander Zanden MJ. 2011. State of the world's freshwater ecosystems: physical, chemical, and biological changes. Annu Rev Environ Resour. 36(1):75–99.
- Chen S, Wang W, Xu W, Wang Y, Wan H, Chen D, Tang Z, Tang X, Zhou G, Xie Z, et al. 2018. Plant diversity enhances productivity and soil carbon storage. Proc Natl Acad Sci USA. 115(16):4027–4032.
- Chen X, Li X, Xie Y, Li F, Hou Z, Zeng J. 2015. Combined influence of hydrological gradient and edaphic factors on the distribution of macrophyte communities in Dongting Lake wetlands, China. Wetlands Ecol Manage. 23(3):481–490.
- Colmer TD, Voesenek LACJ. 2009. Flooding tolerance: suites of plant traits in variable environments. Funct Plant Biol. 36(8):665–681.
- DeMalach N. 2018. Toward a mechanistic understanding of the effects of nitrogen and phosphorus additions on grassland diversity. Perspect Plant Ecol Evol Syst. 32:65–72.
- DeMalach N, Zaady E, Kadmon R. 2017. Light asymmetry explains the effect of nutrient enrichment on grassland diversity. Ecol Lett. 20(1):60–69.
- Duan H-L, Zhao A, Yao Z. 2018. Species relationship among typical marshland plants in Poyang Lake region on different sample scale. J Trop Subtrop Bot. 26:449–456.
- Dudgeon D, Arthington AH, Gessner MO, Kawabata Z-I, Knowler DJ, Leveque C, Naiman RJ, Prieur-Richard A-H, Soto D, Stiassny MLJ, et al. 2006. Freshwater biodiversity: importance, threats, status and conservation challenges. Biol Rev. 81(2):163–182.
- Fu H, Lou Q, Dai T, Yuan G, Huang Z, Ge D, Zou D, Li W, Liu Q, Wu A, et al. 2018. Hydrological gradients and functional diversity of plants drive ecosystem processes in Poyang Lake wetland. Ecohydrology. 11(4):e1950.
- Hobbs RJ, Huenneke LF. 1992. Disturbance, diversity, and invasion – implications for conservations. Conserv Biol. 6(3):324–337.
- Hu J-Y, Xie Y-H, Tang Y, Li F, Zou Y-A. 2018. Changes of vegetation distribution in the east Dongting Lake after the operation of the Three Gorges Dam, China. Front Plant Sci. 9:582.
- Hu Z, Ge G, Liu C, Chen F, Li S. 2010. Structure of Poyang Lake wetland plants ecosystem and influence of lake water level for the structure. Resour Environ Yangtze Basin. 19:597–605 (in Chinese).
- Keddy PA, Reznicek AA. 1986. Great Lakes vegetation dynamics: the role of fluctuating water levels and buried seeds. J Great Lakes Res. 12(1):25–36.
- Lan Z, Chen Y, Li L, Li F, Jin B, Chen J. 2019. Testing mechanisms underlying elevational patterns of lakeshore plant community assembly in Poyang Lake, China. J Plant Ecol. 12(3):438.
- Le C, Zha Y, Li Y, Sun D, Lu H, Yin B. 2010. Eutrophication of lake waters in China: cost, causes, and control. Environ Manage. 45(4):662–668.
- Li F, Hu J, Xie Y, Yang G, Hu C, Chen X, Deng Z. 2018. Foliar stoichiometry of carbon, nitrogen, and phosphorus in wetland sedge Carex brevicuspis along a small-scale elevation gradient. Ecol Indic. 92:322–329.
- Li Y, Bian H, Ren B, Xie Y, Ding X, Yao X, Zhou Q. 2019. Morphological responses of two plant species from different elevations in the Dongting Lake wetlands, China, to variation in water levels. Nordic J Bot. 37(1):e01987.
- Liu SL, Hou XY, Yang M, Cheng FY, Coxixo A, Wu X, Zhang YQ. 2018. Factors driving the relationships between vegetation and soil properties in the Yellow River Delta, China. Catena. 165:279–285.
- Poff NL, Allan JD, Bain MB, Karr JR, Prestegaard KL, Richter BD, Sparks RE, Stromberg JC. 1997. The natural flow regime. Bioscience. 47(11):769–784.
- Pollock MM, Naiman RJ, Hanley TA. 1998. Plant species richness in riparian wetlands – a test of biodiversity theory. Ecology. 79:94–105.
- Qin B. 2002. Approaches to mechanisms and control of eutrophication of shallow lakes in the middle and lower reaches of the Yangze River. J Lake Sci. 14:193–202 (in Chinese).
- Ren Z, Li Q, Chu C, Zhao L, Zhang J, Ai D, Yang Y, Wang G. 2010. Effects of resource additions on species richness and ANPP in an alpine meadow community. J Plant Ecol. 3:25–31.
- Riis T, Hawes I. 2002. Relationships between water level fluctuations and vegetation diversity in shallow water of New Zealand lakes. Aquat Bot. 74(2):133–148.
- Roxburgh SH, Shea K, Wilson JB. 2004. The intermediate disturbance hypothesis: patch dynamics and mechanisms of species coexistence. Ecology. 85(2):359–371.
- Strayer DL, Dudgeon D. 2010. Freshwater biodiversity conservation: recent progress and future challenges. J N Am Benthol Soc. 29(1):344–358.
- Strayer DL, Findlay SEG. 2010. Ecology of freshwater shore zones. Aquat Sci. 72(2):127–163.
- Suding KN, Collins SL, Gough L, Clark C, Cleland EE, Gross KL, Milchunas DG, Pennings S. 2005. Functional- and abundance-based mechanisms explain diversity loss due to N fertilization. Proc Natl Acad Sci USA. 102(12):4387–4392.
- Tan Z, Zhang Q, Li M, Li Y, Xu X, Jiang J. 2016. A study of the relationship between wetland vegetation communities and water regimes using a combined remote sensing and hydraulic modeling approach. Hydrol Res. 47:278–292.
- Tranvik LJ, Downing JA, Cotner JB, Loiselle SA, Striegl RG, Ballatore TJ, Dillon P, Finlay K, Fortino K, Knoll LB, et al. 2009. Lakes and reservoirs as regulators of carbon cycling and climate. Limnol Oceanogr. 54(6part2):2298–2314.
- Wang H-Z, Liu X-Q, Wang H-J. 2016. The Yangtze River floodplain: threats and rehabilitation. In: Chen Y, Chapman DC, Jackson JR, Chen D, Li Z, Killgore KJ, Phelps Q, Eggleton MA, editors. Fishery resources, environment, and conservation in the Mississippi and Yangtze; Bethesda, Maryland: American Fisheries Society, p. 263–291.
- Wang X, Han J, Xu L, Wan R, Chen Y. 2014. Soil characteristics in relation to vegetation communities in the wetlands of Poyang Lake, China. Wetlands. 34:829–839.
- Wilcox DA, Nichols SJ. 2008. The effects of water-level fluctuations on vegetation in a Lake Huron wetland. Wetlands. 28(2):487–501.
- Woodward G. 2009. Biodiversity, ecosystem functioning and food webs in fresh waters: assembling the jigsaw puzzle. Freshw Biol. 54(10):2171–2187.
- Xu X, Zhang Q, Tan Z, Li Y, Wang X. 2015. Effects of water-table depth and soil moisture on plant biomass, diversity, and distribution at a seasonally flooded wetland of Poyang Lake, China. Chin Geogr Sci. 25(6):739–756.
- Yuan S, Yang Z, Liu X, Wang H. 2017. Key parameters of water level fluctuations determining the distribution of Carex in shallow lakes. Wetlands. 37(6):1005–1014.
- Yuan S, Yang Z, Liu X, Wang H. 2019. Water level requirements of a Carex hygrophyte in Yangtze floodplain lakes. Ecol Eng. 129:29–37.
- Zhang D, Qi Q, Wang X, Tong S, Lv X, An Y, Zhu X. 2019. Physiological responses of Carex schmidtii Meinsh to alternating flooding-drought conditions in the Momoge wetland, northeast China. Aquat Bot. 153:33–39.
- Zhang Q, Ye X-C, Werner AD, Li Y-L, Yao J, Li X-h, Xu C-y. 2014a. An investigation of enhanced recessions in Poyang Lake: comparison of Yangtze River and local catchment impacts. J Hydrol. 517:425–434.
- Zhang X, Liu X, Wang H. 2014b. Developing water level regulation strategies for macrophytes restoration of a large river-disconnected lake, China. Ecol Eng. 68:25–31.
- Zhang X, Liu X, Wang H. 2015. Effects of water level fluctuations on lakeshore vegetation of three subtropical floodplain lakes, China. Hydrobiologia. 747(1):43–52.
- Zhao DH, Jiang H, Cai Y, An SQ. 2012. Artificial regulation of water level and its effect on aquatic macrophyte distribution in Taihu Lake. PLoS One. 7:e44836.
- Zhou Y, Bai X, Ning L. 2018. Productivity of Carex cinerascens population and its response to hydrological conditions in the Poyang Lake wetland. Acta Ecol Sin. 38:4953–4963.