Abstract
In Brazil, although, high-altitude shallow lakes comprise large parts of conservation units and are considered highly important environments for biodiversity studies, little is known about the factors that regulate the zooplankton community. In the present study, we sought to identify the effect of regional (hydroperiod and altitude) and local (lake area and macrophyte cover) factors on the zooplankton communities in eight shallow high-altitude lakes (from 1080 m a.s.l. to lakes above 1200 m a.s.l.) in the Espinhaço Mountain Range (Brazil). Of the 116 zooplankton species identified, 66 (56%) showed a high degree of spatial aggregation, occurring in only a few lakes. Accessory species occurred predominantly in small lakes, while rare species were better represented in the larger lakes, suggesting species sorting associated with the niche effect. Zooplankton richness was strongly influenced by hydroperiod, indicating that temporary lakes can congregate species resistant to the environmental filter of the drought, playing an important role in the diversification of the zooplankton community. We identified environmental heterogeneity (macrophyte cover) as the factor that most influenced the species diversity: zooplankton responded positively to increased macrophyte cover, confirming that the presence of aquatic plants constitutes a strong environmental filter to explain the variation of the zooplankton community. Zooplankton species composition and abundance differed among lakes, indicating a certain degree of heterogeneity in assemblage structure, but no influence on zooplankton diversity was observed. Our results provide evidences that altitude did not influence zooplankton diversity and this may affect the regional diversity (ß diversity) of tropical high-altitude lakes, and merits further study.
Introduction
High-altitude lakes are unique environments. Although, these waterbodies occupy only a small fraction of montane regions, they can contain pristine aquatic zones that are references in terms of water quality. Compared to lowland lakes, high-altitude lakes have relatively small catchments, which can determine their morphometric features including water characteristics, lake area, depth and hydroperiod (temporary or permanent). The effect of altitude is decisive for aquatic montane-lake biota. Exposure to intense radiation, especially UV-B during the day and the sudden drop in temperature at night produce the wide daily temperature variations that are a distinctive feature of high-altitude lakes (Calkins and Thordardottir Citation1980; Cabrera et al. Citation1997). Altitude also influences remoteness and connectivity, which are fundamentally related to the assembly structure of aquatic communities, especially the composition, richness and species diversity (Frisch et al. Citation2012).
Richness, diversity and equitability are important components of biodiversity, used to characterize communities and ecosystems, and key aspects of biological diversity. Richness refers to the number of different kinds of individuals regardless of their abundances, while the diversity index of Shannon considers species richness and their relative contribution to total abundance (Shannon and Weaver Citation1949). Equitability, however, measures how similar the frequencies of the different variants are, with low evenness indicating dominance of one or a few types, a typical aspect of selective environments (Magurran Citation2004). These powerful concepts of diversity have been explored to understand the spatial and temporal variation of biodiversity, including the strength of local and regional factors in shaping diversity of species. Many studies in lowland lakes have examined the effects of local and regional filters on zooplankton communities, including environmental heterogeneity (Warfe and Barmuta Citation2004; Celewicz-Goldyn and Kuczynska-Kippen Citation2017), connectivity (Cottenie et al. Citation2003; Thomaz, Bini et al. Citation2007; Lopes et al. Citation2014; Loewen et al. Citation2019), lake area (Oertli et al. Citation2002; Søndergaard et al. Citation2005; Scheffer et al. Citation2006) and hydroperiod (Seminara et al. Citation2008; Vanschoenwinkel et al. Citation2009; Zokan and Drake Citation2015). These studies have clarified the individual and combined effects of regional and local factors on zooplankton communities. However, little attention has been devoted to understanding the role of factors that shape zooplankton community structure in high-elevation shallow lakes in tropical regions.
Despite the importance of research in montane habitats for ecological theory and biodiversity conservation, there are still major gaps concerning zooplankton diversity in Brazilian high-altitude shallow lakes. Studies of the zooplankton community in these waterbodies are in their infancy, and have focused primarily on species descriptions, composition and temporal dynamics (Santos-Wisniewski et al. Citation2002; Moreira et al. Citation2019).
Investigations of the relationship between zooplankton biodiversity indexes and environmental filters may help to clarify important paradigms of theories in community ecology. For example, small lakes may potentially harbor more-diverse zooplankton than larger lakes, because of the high environmental heterogeneity, especially the presence and tridimensional nature of macrophytes (Carpenter and Lodge Citation1986; Meerhoff et al. Citation2003). However, it is not known whether this pattern holds for the zooplankton in high-altitude shallow lakes in tropical regions. Additionally, the effects of hydroperiod and altitude on zooplankton diversity has not been considered in studies performed in Brazilian high-altitude lakes.
The present study investigated the factors that may shape the zooplankton communities in shallow high-altitude lakes in the Espinhaço Mountain Range, the second-largest mountain range in Brazil (central-eastern region). This study, to our knowledge, is the first to address this subject in a Brazilian high-altitude environment. We hypothesized that regional (hydroperiod and altitude) and local factors (lake area and macrophyte cover) influence the zooplankton richness, diversity, equitability and occurrence of rare and accessory species in these environments. We predicted that: (I) Permanent lakes would show higher species richness, diversity, equitability and a higher frequency of rare species compared to temporary lakes, because temporary aquatic habitats limits the occurrence of zooplankton species; (II) Because they are spatially more isolated and with little connectivity, higher-altitude shallow lakes would have lower zooplankton richness, diversity, equitability and a higher frequency of rare species compared to shallow lakes situated at lower altitudes; (III) small lakes would show higher species richness, diversity and equitability, besides a higher frequency of rare species compared to larger lakes; (IV) shallow lakes with low macrophyte cover (lower environmental heterogeneity) would show lower richness, diversity, equitability and lower frequency of rare species compared to shallow lakes with high macrophyte cover and (V) The structure of zooplankton community (considering the balance between composition of species and their relative abundances) is influenced by the regional and local factors investigated.
Materials and methods
Sampling area
The eight lakes studied are located in the Espinhaço Mountain Range (), where elevations range from 900 m to 2000 m and landforms include hills, tablelands, steep slopes and deep river valleys (Bünger et al. Citation2014). Different groups of organisms show high levels of endemism, even though the area has not been thoroughly inventoried, and the region is considered a hotspot for biological conservation. The region has a tropical alternating wet and dry climate with a mean annual rainfall between 125 and 200 mm – the rainy period lasts from October to March – and mean annual temperature between 20 and 26 °C (Da Silva and Bates Citation2002). The shallow lakes (maximum depth < 3 m) are fed mainly by rainfall and groundwater. Coutos and Grande lakes are located on the Gandarela Ridge; the other lakes are located in State Conservation Units: Serra do Cipó National Park (Bonita, Pedras, Rasgada and Ossada lakes), Itacolomi State Park (Seca Lake) and Ouro Branco State Park (Gorila Lake). The lakes were assumed to lack connectivity, since they show no evidence of direct connections to other lakes or nearby wetlands. Pedras, Ossada, Rasgada and Bonita lakes, although, located near the Cipó River, are not influenced by flooding, which does not occur on this stretch of the river (Callisto et al. Citation2002). Coutos is the only lake where fishes are reported (exclusively Oreochromis niloticus, personal note of the author). All other lakes are fishless (personal note of the author).
Sampling procedures of zooplankton and environmental variables
Sampling was carried out at the end of the rainy period, in February and March 2015, always in the daytime. The years 2014 and 2015 were exceptionally dry, with mean rainfall below the historical norm, and few temporary lakes filled, which prevented us from selecting a larger number of temporary environments. The altitude of the lakes (m a.s.l.) was obtained with an Oregon altimeter with a precision of 1 m. Lake surface area was estimated with a long (100 m) vinyl measuring tape and included only the open water zone present during the sampling (methodology adapted from Herrera et al. Citation2018). The lakes were classified in two categories according to water fluctuation: permanent or temporary (lake that dry at least once a year). To determine these categories (permanent or temporary), data from previous studies of the lakes (Moreira et al. Citation2015; Moreira et al. Citation2016) and information provided by the management of the Conservation Units were used. The values (lake area, altitude, macrophyte cover) and categories (permanent and temporary) were applied in the multivariate analyses (PERMANOVA) as an independent variable to explain the variations in the richness of zooplankton species. The mean depth was obtained by taking measurements in each sector with a depth gauge (10 m). For a limnological characterization of the lakes, data for water temperature (°C), pH and electrical conductivity (μS cm−1) were obtained with a Horiba U-50 Multiparameter probe, with several measurements in each section of the lakes (margins and center). Concentrations of total phosphorus (μg L−1) and total nitrogen (μg L−1) were obtained after digestion of samples with potassium persulfate by the ascorbic-acid method, according to APHA (Citation1998). Chlorophyll-a (μg L−1) was determined as described by Jespersen and Christoffersen (Citation1988). Limnological characteristics of the lakes were considered only to the environmental description of the studied lakes.
The degree of coverage of aquatic macrophytes was used as an indicator of lake heterogeneity. Macrophyte cover was estimated from a quadrat (50 × 50 cm), from each sampling point, using the following ranking: 0 (no coverage), 25, 50, 75 or 100% (full quadrat fill) of the bottom covered (methodology adapted from Kosten et al. Citation2009).
For collection of zooplankton, each lake was divided into four sections; the grid was oriented north-south and east-west, using a compass. At the margin and center of each section, water samples (40 L) were obtained with a 5 L Van Dorn bottle. Samples were taken from the water surface, middle and bottom in lakes deeper than 1 m and from the subsurface (5 cm depth) in very shallow (<0.5 m) lakes. A sample was obtained for each section of the lake, totalizing 8 samples in from Seca, Gorila, Boi, Rasgada and Pedra lakes and for Grande lake, we analyzed 7 samples. In Coutos and Ossada lakes, because of the small area during the sampling (<5 m2) and small water volume (both are 0.5 m deep or less), collections (2 samples) were made in only two quadrants, at margin and central of the quadrant, at subsurface of the water column. In the total, there were obtained 49 samples of water and zooplankton. Zooplankton was obtained by filtering 40 L of water from the bucket through plankton net (68 µm mesh). The samples were preserved in 4% buffered formaldehyde solution and stored in 150-mL plastic jars.
For taxonomic identification, organisms were inspected under an optical microscope and identified to genus or species when possible. Rotifers were identified to the lowest taxonomic level possible, following the keys of Ruttner-Kolisko (Citation1974), Koste (Citation1978) and Stemberg (Citation1979). Additional information available at the website http://cfb.unh.edu/cfbkey/html/begin.html was also consulted. For cladocerans, taxonomic references included those by Brooks (Citation1959), Elmoor-Loureiro (Citation2007) and Van Damme and Dumont (Citation2010) and the descriptions found in the following website: https://cladocera.wordpress.com/. For copepods the taxonomic keys by Reid (Citation1985) and Fernando (Citation2002). For the quantitative analysis, 5 1-mL subsamples were placed in a Sedgwick-Rafter chamber and analyzed under an optical microscope. The entire sample was then actively searched for rare species. The species richness and relative abundance (%) were calculated from the sample counts.
Data analysis
The possible influence of regional (hydroperiod and altitude) and local (lake area and macrophyte cover) factors on zooplankton community was analyzed based on four indexes: species richness (i.e. α diversity), diversity (Shannon’s index), equitability (Pielou’s index) and frequency of occurrence of rare and accessory species (i.e. species that showed frequency of occurrence up to 20%, between 21% and 50% and greater than 50%, respectively), according to De Bernardi (Citation1984). Each index was tested for set of samples sorted within the factors investigated in this study. The frequency of occurrence was considered as the relationship between the occurrence of the different taxa and the total number of samples. Only one species (the rotifer Lecane bulla) was classified as constant and excluded from the subsequent analyzes. A species accumulation curve was constructed to visualize the increase of species in all the lakes, based on the number of samples analyzed. The accumulation curve was projected to assure that the small numbers of samples wouls allow us to explore in the best way our results. The curve was estimated by the method of random permutations of the data without replacement. The normality and homoscedasticity of richness, diversity, equitability and the percentage of rare and accessory species were tested using the Kolmogorov–Smirnov and Bartlett tests, respectively. The Mann–Whitney test was used to determine possible differences in richness, diversity, equitability and percentages of rare, accessory and constant species based on hydroperiod (temporary vs. permanent lakes), altitude (lakes located up to 1080 m a.s.l. vs. lakes located above 1220 m a.s.l.) and area (smaller than 200 m2 vs. larger than 280 m2). The Kruskal–Wallis ANOVA was used with the Mann–Whitney test for post hoc pairwise comparisons to assess differences in species richness, diversity, equitability and percentage of rare, accessory and constant species among lakes under five levels of macrophyte cover: 0%, 25%, 50%, 75% and 100%. Additionally, the PERMANOVA was used to test the influence of local and regional factors on zooplankton community structure (based on relative abundance data). Posteriorly, Bray–Curtis similarity matrices and non-metric multidimensional scaling (NMDS) plots were produced for each factor tested to visualizing dissimilarities among samples. Hydroperiod and altitude were considered regional factors, while the lake area and macrophyte cover were considered as local factors. Statistical analyses followed the recommendations of Zar (Citation1996) and Anderson (Citation2001). All analyses were conducted using the software R version 3.4.0 (R Core Team Citation2011) with the vegan package (Oksanen et al. Citation2013).
Results
Environmental variables
Limnological conditions of the surveyed lakes indicated the prevalence of neutral to acid conditions (pH from 7 to 5.8). Water temperature (oC) revealed the altitudinal influence in Gorila, Grande and Seca lakes (>1080 masl), with median values from 18.6 °C to 22.5 °C. Eutrophic conditions were prevalent, with high values of chlorophyll a (ranging from 10.1 and 71.5 ug.L−1) and total nitrogen (from 553.3 and 1117.2 ug.L−1; ).
Table 1. Characteristics of the selected shallow lakes, mean (SD) limnological parameters values and hydroperiod classification.
Community composition and spatial distribution
One hundred and sixteen zooplankton taxa were identified in all lakes. Rotifera was the most diverse group (72 species), followed by Cladocera (28 species) and Copepoda (16 species). Seven species were the most frequent (62.5%), occurring in at least 5 of the lakes studied: the rotifers Lecane bulla, Lecane lunaris and Lepadella patella, the cladocerans Alona ossiani, Alonella dadayi and Anthalona verrucosa and the cyclopoid copepod Microcyclops anceps. Of the zooplankton species, 56% (66 species) occurred only in two lakes, suggesting a high degree of spatial aggregation. The most abundant species were the rotifers Polyarthra vulgaris (58.8%), Polyarthra dolichoptera (34.0%), Lecane bulla (32.9%), members of Bdelloidea (29.3%), Anuaeropsis fissa (26.6%), Tricocherca similis (24.3%), Lecane curvicornis (23.7%) and Mytilina ventralis (18.7%); the cladocerans Ephemeroporus tridentatus (26.6%), Alonella dadayi (15.2%) and Chydorus sp. (13%), followed by cyclopoid copepods (16.8%) and the calanoid Notodiaptomus sp. (16.3%) Rotifers dominated the zooplankton community in all the temporary lakes in terms of abundance (Ind.L−1) and number of species, while in permanent lakes cladocerans and copepods were more abundant (except for Pedras lake where rotifers were more abundant). (Supporting Information Figure S1). A complete list of species and their relative abundances (%) is given in . As seen in , maximum zooplankton diversity was obtained in all the lakes and no species was unsampled, even considering the low number of samples.
Table 2. Zooplankton occurrence and relative abundance (%) in the studied lakes.
Richness, diversity and equitability and frequency of occurrence vs. local and regional factors
Total zooplankton richness was higher in the samples from temporary lakes (average of 22.8 species) than from permanent lakes (average of 16.8; ; ). Despite the greater richness, zooplankton from temporary lakes was characterized by dominance of species, reflected by the lower equitability value (0.56) in relation to permanent lakes (0.65; ; Mann–Whitney p < .01; ; ). The diversity and frequency of rare and accessory species were not influenced by hydroperiod (Mann–Whitney p > .01) . Zooplankton richness, diversity and equitability as well as the contribution of rare and accessory species showed no differences between lakes situated at higher (>1220 m a.s.l.) and lower (<1080 m a.s.l.) altitudes ().
Table 3. Total number of species, biotic indices expressed as mean values of the Shannon diversity index (H′), species richness (S), Pielou’s evenness index (J′) and frequency of occurrence of zooplankton species.
Table 4. Nonparametric analysis (Mann–Whitney and Kruskal–Wallis) of the local and regional factors and the relationships between biotic indices and frequency of occurrence of zooplanktonic species.
Lake area did not explain the richness, diversity and equitability of total zooplankton but larger lakes promoted a higher contribution of rare species compared to smaller lakes, while smaller lakes presented a higher contribution of accessory species compared to larger lakes (). Analysis of the local predictor indicated that zooplankton richness, diversity and equitability was more influenced by macrophyte cover (Kruskal–Wallis test, p < .01). Macrophyte cover made notables contributions also in the abundance of rare and accessory species (Kruskal–Wallis test, p < .01; ; ).
Figure 4. Relative abundance (%) of rare and accessory species in lakes with <200 and >280 m2 of area.
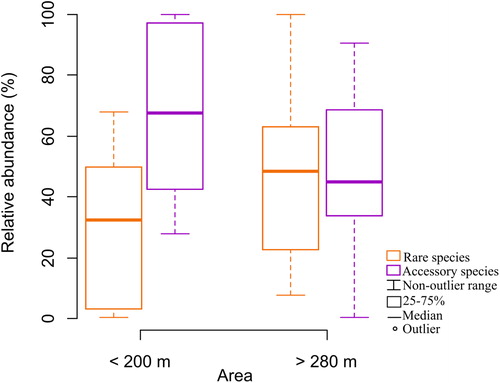
Figure 5. Effects of macrophyte cover on biotic indices of zooplankton community (A, B and C) and relative abundance (%) of rare and accessory species an macrophyte cover (D and E). RA: relative abundance.
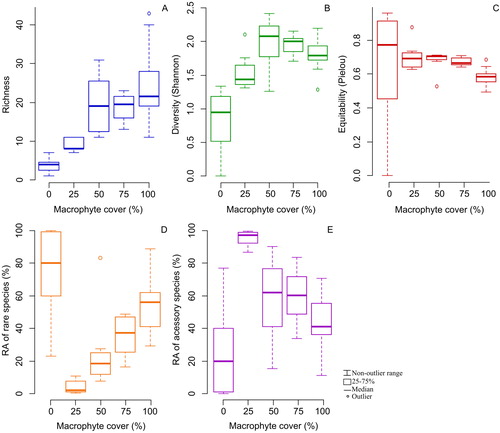
PERMANOVA analysis based on the zooplankton community abundance indicated that different zooplankton groups and total zooplankton were statistically different and significantly influenced by a balance of regional (hydroperiod and altitude) and local (area and macrophyte cover) factors (). Comparisons between communities revealed that lake area, macrophyte cover and hydroperiod presented the most visible separation of the sections in a multidimensional space used to illustrate univariate zooplankton community structure ().
Figure 6. Non-metric multidimensional scaling plot illustrating the model based on the zooplankton abundance composition and selected local (area and macrophyte cover) and regional (altitude and hydroperiod) factors. Symbols represent the different communities.
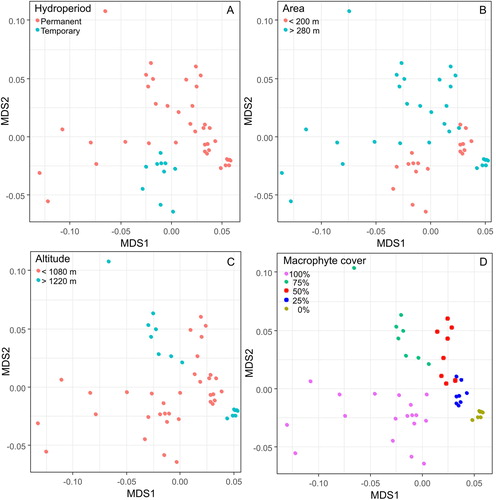
Table 5. Results from multivariate PERMANOVA analysis testing differences in zooplankton community abundance for differences between local (area and macrophyte cover) and regional (hydroperiod and altitude) factors and interaction between these factors.
Discussion
The results of our study indicated that the high-altitude tropical shallow lakes in the Espinhaço Mountain Range harbor a significant richness of zooplankton and that zooplankton richness, diversity and equitability were strongly influenced by a balance between some regional (hydroperiod) and local (macrophyte cover) factors.
The predictable and stable hydrological pattern of the permanent montane lakes did not prevent zooplankton from showing higher richness in temporary habitats compared to permanent ones, refuting our first hypothesis and confirming the magnitude of the regional factor (hydroperiod) in affecting zooplankton richness. It is worthy to point out that, most samples from permanent lakes contained many species that occurred only once, in a single sample. Therefore, the high levels of constant species in temporary lakes increased the richness in these lakes, although, the permanent lakes showed a high number of species occurrences.
Hydroperiod is one of the key selective agents that influences local adaptations of populations and interactions of aquatic biota (Boven and Brendonck Citation2009; Incagnone et al. Citation2015). Although, dry periods can affect the viability of the resting-stage bank of zooplankton in temporary waters (Brock et al. Citation2003; Cáceres and Tessier Citation2003), shaping zooplankton community structure in short and long time, a positive effect of the fluctuating hydroperiod on zooplankton richness has been described, including constraints on the abundance and biomass of predators and increases in spatial and temporal heterogeneity (Spencer et al. Citation1999; Jenkins et al. Citation2003).
Altitude was not a determining factor for the total zooplankton richness, which did not differ between lakes at lower (<1008 m a.s.l.) and higher altitudes (>1280 m a.s.l.). Even though in high-altitude montane areas (>3000 m a.s.l.) the limiting effect of extreme environmental conditions on the diversity of aquatic biota is amply documented (Morales-Baquero et al. Citation1989; Coronel et al. Citation2007; Madsen et al. Citation2015). Our results showed that these lower-altitude tropical montane lakes (<2000 m a.s.l.) had very similar zooplankton assemblages and can be considered a homogeneous community in terms of species richness, diversity and equitability as well as regarding to the frequency of occurrence of rare and accessory species. Several factors may be associated with this homogenization: similar environmental characteristics among the lakes (environmental heterogeneity and limnological conditions, for example) and the dispersal and emergence of individuals through propagules. Therefore, factors associated with dispersal and colonization may help to explain this similarity between zooplankton assemblages between higher- and lower-altitude lakes. In fact, the study by Eskinazi-Sant’Anna and Pace (Citation2018) showed that recolonization of the zooplankton through emergence from the resting-egg bank is a strong force driving the zooplankton community in some of these temporary high-altitude shallow lakes.
Our results indicated that a large variation of zooplankton species was influenced by lake area (local factor), mostly because large lakes congregated a high portion of rare species and smaller lakes presented a higher contribution of accessory species. Larger pools may provide more microhabitats for aquatic biota, reducing the chance of local extinction during the harsh seasonal period of dry, while the contribution of rare species is predicted to be lower in small lakes (Eitam et al. Citation2004). Besides this, large pools can provide more stable physicochemical conditions than smaller pools (Kiflawi et al. Citation2003), contributing to enhance local biodiversity.
We identified that lakes with small area were determinant to the highest abundance of zooplankton accessory species. Although, small lakes can be very limiting habitats for some aquatic groups (e.g. fish), these waterbodies can show a reverse pattern of abundance and richness for many other aquatic organisms, helping to preserve metapopulations and communities (Leibold et al. Citation2004; Williams et al. Citation2004). Small lakes are more exposed to frequent events of desiccation and inundations, which is a selection pressure to zooplankton species with severe consequences for species with a longer life cycle such as copepods and cladocerans. The high frequency of production of dormant stages can contribute to the structure of the actual communities and the dominance of the species less affected by the constrains imposed by environmental fluctuations in small lakes. The higher contribution of accessory species in small lakes may reflect a higher dominance of zooplankton species adapted to the fewer niches that small lakes can provide compared to larger ones, which suggest that small lakes may promote increases in dominance of specific species in zooplankton communities of tropical high-altitude lakes.
Macrophyte cover was the most prominent local factor that significantly influenced zooplankton richness, diversity, equitability and the frequency of occurrence of rare and accessory species. Many representatives of the zooplankton assembly present a strong relationship between the presence of aquatic vegetation and high levels of abundance and diversity, been highly specialized to exploit microenvironments and substrate availability provided by vegetation (Scheffer Citation2004; Forró et al. Citation2008). Others mechanisms associated with zooplankton occurrence in densely macrophyte-covered aquatic ecosystems are also described, including the less intense predation (Rennie and Jackson Citation2005), the antipredador refuge effect (Meerhoff et al. Citation2007), the increase of niche availability, including food sources (Wellborn et al. Citation1996; Frisch et al. Citation2006; Waterkeyn et al. Citation2008) and the physical complexity habitat provides by banks of macrophytes, which has been shown to be positively associated to zooplankton diversity (Bolduc et al. Citation2016).
The phytoplanton Chl-a concentration was not significantly correlated to diversity and richness of zooplankton, although, high availability has been detected in all studied lakes (> Chl-a 10 µg.L−1). The direct trophic connection between zooplankton and Chl-a seems to be less significant to zooplankton diversity in small, shallow lakes, densely covered by macrophyte beds (Phiri et al Citation2011; Braghin et al. Citation2016) which are colonized mainly for epibenthonic zooplanktonic species. For these species, the most abundant forms detected in the present study, others food sources such as periphytic algae, bacteria and ciliates present in macrophytes, can be the most important factor to influence diversity (Hinojosa-Garro et al. Citation2010; Algarte et al. Citation2017).
Although, caution is needed when generalizing patterns extracted from different communities, it is indubitable the extreme importance of the heterogeneity effect of macrophyte beds enhancing invertebrate diversity as indicated in our study, as also observed in others no-altitudinal tropical lakes (Thomaz, Dibble et al. Citation2007; Maia-Barbosa et al. Citation2008).
It is worth mentioning that a potential predator–prey interaction between zooplankton and fish and macroinvertebrates in the studied habitats was not considered in the present paper due to the lack of data of fish and macroinvertebrates composition and abundance. In particular, the presence and identity of predators can be affected by the hydroperiod length with fish as the dominant predators in permanent ponds while in temporary ponds invertebrates and larval amphibians are the dominant predators in fishless habitats (Pintar and Resetarits Citation2018). The study of Tavares-Junior et al. (Citation2020) reveals that tapdoles community presents an expressive species diversity in two of the studied lakes (Gorila and Seca), but functional diversity of tapdoles was not quantified, making it difficult to assess the potential impact of tapdoles predation on zooplankton.
The significant difference in the zooplankton structure (composition and abundance) may be associated with the local environmental constrains, shaping the patterns of occurrence of rare and accessory species. Hydroperiod is considered to be decisive forcing force to zooplankton community (Havel et al. Citation2000; Dias et al. Citation2017), mainly because they influence the dynamics of populations subject to the severe conditions imposed by the extension of the drought period. In addition, due to the small area and shallow depth of the studied lakes, even little variations in environmental conditions (e.g. altitudinal local effect, different mosaics and degree of macrophyte coverage) may represent decisive conditions to zooplankton species selection in small waterbodies.
In summary, our results indicated that there was a noticeable influence of the local and regional factors on the zooplankton community. The zooplankton richness, diversity, equitability and the frequency of occurrence of rare and accessory species in the high-altitude lakes of the Espinhaço Mountain Range are shaped by the action of different local and regional factors, which affect the zooplankton groups in different ways. Hydroperiod and lake area (regional), and macrophyte cover (regional) are the factors that positively influenced the biodiversity indexes tested. Environmental heterogeneity can be considered the most important factor affecting zooplankton community, since all indexes tested responded significantly to increased macrophyte cover. The altitudinal lakes harbored zooplankton assemblages consisting mainly of rare and accessory species, probably in response to the strong environmental filter of drying on species that are most sensitive to this type of disturbance. Rare species were better represented in the larger lakes, indicating species sorting associated with the niche effect.
Although, the zooplankton community structure has shown dissimilarities in abundance and composition among the lakes, we did not observe a clear influence of altitude in zooplankton richness, diversity and equitability, as opposed to observations in other high-altitude environments, suggesting that in lower-altitude tropical montane environments the zooplankton community is subject to a greater effect of species homogenization, which could influence the regional diversity (ß diversity). Further studies would be useful to better understand the influence of altitude on zooplankton richness in these still insufficiently studied environments. To improve predictions derived from the present study of the temporary and permanent lakes from Espinhaço Mountain Range, future research should include a greater number of aquatic habitats (pools, ponds and lakes) and the effects of biotic interactions specially competition and vertebrate and invertebrate predation. All around the globe, mountain areas are centers of endemism and diversity on account of their isolation and altitudinal diversity. In view of its distinctive characteristics, anthropic pressure and endemism, the Espinhaço Mountain Range was declared an area of extreme biological importance (Drummond et al. 2005). The study is a first attempt to elucidate the influence of local and regional factors on zooplankton diversity in aquatic environments from a threatened and still insufficiently studied mountain range from Southeast Brazil.
Acknowledgements
Sincere thanks to Emerson Silva and Francisco W. A. Moreira for assistance in the field and laboratory processing of samples. We gratefully acknowledge Dr. Janet Reid for English revision and comments to the early version of the manuscript. Two reviewers provided helpful comments on draft of this manuscript.
Disclosure statement
No potential conflict of interest was reported by the author(s).
Additional information
Funding
References
- Algarte VM, Siqueira T, Landeiro VL, Rodrigues L, Bonecker CC, Rodrigues LC, Santana NF, Thomaz SM, Bini LM. 2017. Main predictors of periphyton species richness depend on adherence strategy and cell size. PLoS One. 12(7):e0181720.
- Anderson MJ. 2001. A new method for non-parametric multivariate analysis of variance. Austral Ecol. 26(1):32–46.
- APHA. 1998. Standard methods for the examination of water and wastewater. Washington (DC): American Public Health Association.
- Bolduc P, Bertolo A, Pinel-Alloul B. 2016. Does submerged aquatic vegetation shape zooplankton community structure and functional diversity? A test with a shallow fluvial lake system. Hydrobiologia. 778(1):151–165.
- Boven L, Brendonck L. 2009. Impact of hydroperiod on seasonal dynamics in temporary pool cladocerans communities. Fund App Lim. 174(2):147–157.
- Braghin LSM, Simões NR, Bonecker CC. 2016. Hierarchical effects of local factors on zooplankton species diversity. Inland Waters. 6(4):645–654.
- Brock MA, Nielsen DL, Shiel RJ, Green JD, Langley JD. 2003. Drought and aquatic community resilience: the role of eggs and seeds in sediments of temporary wetlands. Freshwater Biol. 48(7):1207–1218.
- Brooks JL. 1959. Cladocera. In: Edmondson WT, editor. Freshwater biology. Vol. 2. New York (NY): Wiley; p. 587–656.
- Bünger MO, Stehmann JR, Oliveira-Filho AT. 2014. Myrtaceae throughout the Espinhaço Mountain Range of central-eastern Brazil: floristic relationships and geoclimatic controls. Acta Bot Bras. 28(1):109–119.
- Cabrera S, López M, Tartarotti B. 1997. Phytoplankton and zooplankton response to ultraviolet radiation in a high-altitude Andean lake: short – versus long-term effects. J Plankton Res. 19(11):1565–1582.
- Cáceres CE, Tessier AJ. 2003. How long to rest: the ecology of optimal dormancy and environmental constraint. Ecology. 84(5):1189–1198.
- Calkins J, Thordardottir T. 1980. The ecological significance of solar UV radiation on aquatic organisms. Nature. 283(5747):563–566.
- Callisto M, Moreno P, Goulart M, Medeiros AO, Petrucio M, Moretti M, Mayrink N, Rosa CA. 2002. The assessment of aquatic biodiversity along an altitudinal gradiente at the Serra do Cipó. Verh Internat Verein Limnol. 28(4):1814–1817.
- Carpenter SR, Lodge DM. 1986. Effects of submerged macrophytes on ecosystem process. Aquat Bot. 26:341–370.
- Celewicz-Goldyn S, Kuczynska-Kippen N. 2017. Ecological value of macrophyte cover in creating habitat for microalgae (diatoms) and zooplankton (rotifers and crustaceans) in small field and forest water bodies. PLoS One. 12:1–14.
- Coronel JS, Declerck S, Brendonck L. 2007. High-altitude peatland temporary pools in Bolivia house a high cladocerans diversity. Wetlands. 27(4):1166–1174.
- Cottenie K, Michels E, Nuytten N, De Meester L. 2003. Zooplankton metacommunity structure: regional vs. local processes in highly interconnected ponds. Ecology. 84(4):991–1000.
- Da Silva JMC, Bates JM. 2002. Biogeographic patterns and conservation in the south American Cerrado: a tropical savanna hotspot. Bioscience. 52:225–253.
- De Bernardi R. 1984. Methods for the estimation of zooplankton abundance. In: Downing J, Rigler FH, editors. A manual on methods for the assessment of secondary productivity in freshwaters. London: Blackwell Scientific Publication, (IBP Handbook n. 17); p. 59–86.
- Dias JD, Miracle MR, Bonecker CC. 2017. Do water levels control zooplankton secondary production in Neotropical floodplain lakes? Fund App Lim. 190(1):49–62.
- Drummond GM, Martins CS, Machado ABM, Sebaio FA, Antonini Y, editors. 2005. Biodiversidade em Minas Gerais: um atlas para sua conservação. 2nd ed. Belo Horizonte (Brazil): Fundação Biodiversitas; p. 222.
- Eitam A, Blaustein L, Damme KV, Dumont HJ, Martens K. 2004. Crustacean species richness in temporary pools: relationships with habitat traits. Hydrobiologia. 525(1–3):125–130.
- Elmoor-Loureiro LMA. 2007. Phytophilous cladocerans (Crustacea, Anomopoda and Ctenopoda) from Paranã River Valley, Goiás, Brazil. Rev Bras Zool. 24(2):344–352.
- Eskinazi-Sant’Anna EM, Pace M. 2018. The potential of the zooplankton resting-stage bank to restore communities in permanent and temporary waterbodies. J Plankton Res. 40(4):458–470.
- Fernando CH. 2002. A guide to tropical freshwater zooplankton -identification. In: Fernando CH, editor. Ecology and impact on fisheries. Leiden (The Netherlands): Backhuys Publishers; p. 291.
- Forró I, Korovchinsky MM, Kotov AA, Petrusek A. 2008. Global diversity of cladocerans (Cladocera; Crustacea) in freshwater. Hydrobiologia. 595(1):177–184.
- Frisch D, Cottenie K, Badosa A, Green AJ. 2012. Strong spatial influence on colonization rates in a pioneer zooplankton metacommunity. PLoS One. 7(7):e40205.
- Frisch D, Moreno-Ostos E, Green AJ. 2006. Species richness and distribution of copepods and cladocerans in temporary ponds of Doñana Natural Park. Hydrobiologia. 556(1):327–340.
- Havel JE, Eisenbacher EM, Black AA. 2000. Diversity of crustacean zooplankton in riparian wetlands: colonization and egg banks. Aquat Ecol. 34(1):63–76.
- Herrera EQ, Jacobsen D, Casas J, Dangles O. 2018. Environmental and spatial filters of zooplankton metacommunities in shallow pools in high-elevation peatlands in the tropical Andes. Freshw Biol. 63(5):432–442.
- Hinojosa-Garro D, Mason CF, Underwood GJC. 2010. Influence of macrophyte spatial architecture on periphyton and macroinvertebrate community structure in shallow water bodies under contrasting land management. Fund App Lim. 177(1):19–37.
- Incagnone G, Marrone F, Barone R, Robba L, Naselli-Flore L. 2015. How do freshwater organisms cross the “dry ocean”? A review on passive dispersal and colonization process with a special focus on temporary ponds. Hydrobiologia. 750(1):103–123.
- Jenkins DG, Grissom S, Miller K. 2003. Consequences of prairie wetland drainage for crustacena biodiversity and metapopulations. Conserv Biol. 17(1):158–167.
- Jespersen AM, Christoffersen K. 1988. Measurements of chlorophyll a from phytoplankton using ethanol as extraction solvent. Arch Fur Hydrobiol. 109:445–454.
- Kiflawi M, Eitam A, Blaustein L. 2003. The relative impact of local and regional processes on macroinvertebrate species richness in temporary pools. J Anim Ecol. 72(3):447–452.
- Koste W. 1978. Rotatoria. Vol. 2. Berlin (Germany): Gebruder Borntraeger.
- Kosten S, Lacerot G, Jeppesen E, Marques DM, van Nes EH, Mazzeo N, Scheffer M. 2009. Effects of submerged vegetation on water clarity across climates. Ecosystems. 12(7):1117–1129.
- Leibold MA, Holyoak M, Mouquet N, Amarasekare P, Chase JM, Hoopes MF, Holt RD, Shurin JB, Law R, Tilman D, et al. 2004. The metacommunity concept: a framework for multi-scale community ecology. Ecol Lett. 7(7):601–613.
- Loewen CJG, Strecker AL, Larson GL, Vogel A, Fischer JM, Vinebrooke RD. 2019. Macroecological drivers of zooplankton communities across the mountains of western North America. Ecography. 42(4):791–803.
- Lopes PM, Bini LM, Declerck SAJ, Farjalla VF, Vieira LCG, Bonecker CC, Lansac-Toha FA, Esteves FA, Bozelli RL. 2014. Correlates of zooplankton beta diversity in tropical lake systems. PLoS One. 9(10):e109581.
- Madsen PB, Morabowen A, Andino P, Espinosa R, Cauvy-Fraunié S, Dangles O, Jacobsen D. 2015. Altitudinal distribution limits of aquatic macroinvertebrates: an experimental test in a tropical alpine stream. Ecol Entomol. 40(5):629–638.
- Magurran AE. 2004. Medindo a diversidade biológica. Curitiba (Brazil): Editora UFPR; 261 p.
- Maia-Barbosa PM, Peixoto RS, Guimarães AS. 2008. Zooplankton in littoral waters of a tropical lake: a revisited biodiversity. Braz J Biol. 68(4 Suppl):1069–1078.
- Meerhoff M, Iglesias C, DE Mello FT, Clemente JM, Jensen E, Lauridsen TL, Jeppesen E. 2007. Effects of habitat complexity on community structure and predator avoidance behavior of littoral zooplankton in temperate versus subtropical shallow lakes. Freshwater Biol. 52(6):1009–1021.
- Meerhoff M, Mazzeo N, Moss B, Rodríguez-Gallego L. 2003. The structuring role of free-floating versus submerged plants in a subtropical shallow lake. Aquat Ecol. 37(4):377–391.
- Morales-Baquero R, Cruz-Pizarro L, Carrillo P. 1989. Patterns in the composition of the rotifer communities from high mountain lakes and ponds in Sierra Nevada (Spain). Hydrobiologia. 186–187(1):215–221.
- Moreira FW, Dias ES, Sant’Anna EME. 2015. First record of the endemic phytophilous cladoceran Celsinotum candango Sinev & Elmoor-Loureiro, 2010, in Minas Gerais state, in a threatened shallow lake at Serra do Gandarela. Biota Neotrop. 15(4):1–5.
- Moreira RA, Eskinazi-Sant’anna EM, Previattelli D. 2019. Forficatocaris odeteae n. sp., a new Parastenocarididae (Copepoda, Harpacticoida) from a high-altitude pond in Minas Gerais state, Brazil. Crustaceana. 92(2):163–176.
- Moreira RA, Rocha O, Santos RM, Dias ES, Moreira FWA, Eskinazi-Sant’anna EM. 2016. Composition, body-size structure and biomass of zooplankton in a high-elevation temporary pond (Minas Gerais, Brazil). Oecol Aust. 20:81–93.
- Oertli B, Joye DA, Castella E, Juge R, Cambin D, Lachavanne J-B. 2002. Does size matter? The relationship between pond area and biodiversity. Biol Conserv. 104(1):59–70.
- Oksanen JFG, Blanchet R, Kindt P. 2013. Vegan: community ecology package. [accessed 2019 Feb 02]. http://cran.r-project.org/web/packages/vegan/index.html.
- Phiri C, Chakona A, Day JA. 2011. The effect of plant density on epiphytic macroinvertebrates associated with a submerged macrophyre, Lagarosiphon ilicifolis Obermeyer, n Lake Kaniba, Zimbabwe. Afr J Aquat Sci. 36(3):289–297.
- Pintar MR, Resetarits WJ. 2018. Filling ephemeral ponds affects development and phenotypic expression in Ambystoma talpoideum. Freshw Biol. 63(9):1173–1183.
- R Core Team. 2011. R: a language and environment for statistical computing. Vienna (Austria): R Foundation for Statistical Computing.
- Reid JW. 1985. Chave de identificação e lista de referências bibliográficas para as especies continentais sulamericanas de vida livre da Ordem Cyclopoida (Crustacea, Copepoda). Bol Zool. 9(9):17–143.
- Rennie MD, Jackson LJ. 2005. The influence of habitat complexity on littoral invertebrate distributions: patterns differ in shallow prairie lakes with and without fish. Can J Fish Aquat Sci. 62(9):2088–2099.
- Ruttner-Kolisko A. 1974. Plankton rotifers biology and taxonomy. Vol 26. Stuttgart (Germany): Lubrecht & Cramer Ltd.
- Santos-Wisniewski MJ, Rocha O, Güntzel AM, Matsumura-Tundisi T. 2002. Cladocera Chydoridae of high altitude water bodies (Serra da Mantiqueira), in Brazil. Braz J Biol. 62(4A):681–687.
- Scheffer M. 2004. Ecology of shallow lakes. Dordrecht (Netherlands): Springer; p. 357.
- Scheffer M, van Geest GJ, Zimmer K, Jeppesen E, Søndergaard M, Butler MG, Hanson MA, Declerck S, De Meester L. 2006. Small habitat size and isolation can promote species richness: second-order effects on biodiversity in shallow lakes ponds. Oikos. 112(1):227–231.
- Seminara M, Vagaggini D, Margaritora FG. 2008. Differential responses of zooplankton assemblages to environmental variation in temporary and permanent ponds. Aquat Ecol. 42(1):129–140.
- Shannon CE, Weaver W. 1949. The mathematical theory of communication. Urbana (IL): University of Illinois Press.
- Søndergaard M, Jeppesen E, Jensen JP. 2005. Pond or lake: does it make any difference? Arch Hydrobiol. 162(2):143–165.
- Spencer M, Blaustein L, Schwartz SS, Cohen JE. 1999. Species richness and the proportion of predatory animals species in temporary freshwater pools: relationships with habitat size and permanence. Ecol Lett. 2:157–166.
- Stemberg R. 1979. A guide to Rotifers. Vol. 1. Cincinnati (OH): U.S. Environmental Protection Agency.
- Tavares-Junior C, Eskinazi-Sant'anna EM, Pires MRS. 2020. Environmental drivers of tadpole community structure in temporary and permanent ponds. Limnologica. 81:125764.
- Thomaz SM, Bini LM, Bozelli R. 2007. Floods increase similarity among aquatic habitats in river-floodplain systems. Hydrobiologia. 579(1):1–13.
- Thomaz SM, Dibble ED, Evangelista LR, Higuti J, Bini LM. 2007. Influence of aquatic macrophyte habitat complexity on invertebrate abundance and richness in tropical lagoons. Freshwater Biol. 071116231725007–071116231725367.
- Van Damme K, Dumont HJ. 2010. Cladocera of the Lençóis Maranhenses (NE - Brazil): faunal composition and a reappraisal of Sars' Method. Braz J Biol. 70(3 Suppl):755–779.
- Vanschoenwinkel B, Hulsmans A, De Roeck E, De Vries C, Seaman M, Brendonck L. 2009. Community structure in temporary freshwater pools: disentangling the effects of habitat size and hydroregime. Freshwater Biol. 54(7):1487–1500.
- Warfe DM, Barmuta LA. 2004. Habitat structural complexity mediates the foraging success of multiple predator species. Oecologia. 141(1):171–178.
- Waterkeyn A, Grillas P, Vanschoenwinkel B, Brendonck L. 2008. Invertebrate community patterns in Mediterranean temporary wetlands along hydroperiod and salinity gradients. Freshwater Biol. 53(9):1808–1822.
- Wellborn GA, Skelly DK, Werner EE. 1996. Mechanisms creating community structures across a freshwater habitat gradient. Annu Rev Ecol Syst. 27(1):337–363.
- Williams P, Whitfield M, Biggs J, Bray S, Fox G, Nicolet P, Sear D. 2004. Comparative biodiversity of rivers, streams, ditches and ponds in a agricultural landscape in Southern England. Biol Conserv. 115(2):329–341.
- Zar JH. 1996. Biostatistical analysis. 3rd ed. Upper Saddle River (NJ): Prentice-Hall International Editions.
- Zokan M, Drake JM. 2015. The effect of hydroperiod and predation on the diversity of temporary pond zooplankton communities. Ecol Evol. 5(15):3066–30074.