Abstract
The present study was conducted to investigate the potential of the free-floating macrophytes Pistia stratiotes to remediate nutrients and restore the eutrophic drain (Al-Sero Drain), South Nile Delta, Egypt. Plant and water samples were collected monthly for ten months using three randomly distributed quadrats in each of three sites. Monthly significant variation in all investigated water nutrients was recorded. The plant biomass showed bell-shaped distribution, with the lowest shoot and root biomass during May, while their peaks were during September. The plant shoots accumulated higher concentrations of most nutrient elements, except Mg, than the roots. The order of nutrients concentration (%) in P. stratiotes shoot was K > N > Ca > Na > Mg > P, while in the root was K > N > Na > Ca > Mg > P. Most nutrients standing stock (g/m−2) had the same biomass trend with the minimum during May and the maximum during August–October, which is the potential period for mowing the plant to remediate the highest nutrients and restore the eutrophic watercourses. Most investigated nutrients (except Ca) content in water were significantly correlated to their concentration in the different plant organs, which in turn provide a quantitative assessment of the environmental quality that suggests the potential use of this plant as a biomonitor of nutrient elements in eutrophic watercourses.
Introduction
Eutrophication has been recognized as the most common and severe environmental hazard in wetland ecosystems (Eid et al. Citation2020). It is considered the most widespread global water quality issue (Zhang et al. Citation2019). Eutrophication results from the excessive nutrient delivery into natural water bodies, which leads in turn to excess plant growth and the exclusion of less competitive species (Sinha et al. Citation2017; O’Hare et al. Citation2018). These nutrients have a considerable variety of sources; they may not only come from urban and industrial sewage but also agricultural lands (Costa et al. Citation2018). Increasing quantities of agricultural drainage water carrying heavy fertilizer and pesticide loads contribute significantly to the eutrophication and pollution of the water bodies (Okbah Citation2005). The increasing use of fertilizer results in significant buildup of nutrients such as N and P (Smith et al. Citation2007), which are subjected to losses by leaching and surface runoff resulting in impaired water quality and reduced water availability due to accelerated eutrophication (Yamashita and Yamamoto-Ikemoto Citation2014). Common symptoms of eutrophication include decreased water clarity and dense algal blooms (Lapointe et al. Citation2015; El-Amier et al. Citation2016).
In highly eutrophic wetlands, where nutrient loading is high, macrophytes may retain large nutrients content (Meuleman et al. Citation2003), and the denitrification process may remove more nitrogen from soil or sediment, compared with environments having lower nutrient loadings (Eid et al. Citation2020). Aquatic macrophytes have been known to possess the ability to sequester large quantities of nutrients through their uptake by foliar absorption (Shaltout et al. Citation2014). They have high remediation potential for macronutrients because of their general fast growth and high biomass production (Ghazi et al. Citation2019). The growth strategy of floating aquatic macrophytes can have important implications for their relative value for nutrient uptake and sequestration, and an understanding of the allocation of nutrients between plant tissues will be crucial for maximizing nutrient removal via harvesting (Reddy and De Busk Citation1985).
In Egypt, drains are small, linear water bodies, usually less than 1.5 m deep and several meters wide (Ali Citation2011). They have an important ecological function, where they provide a habitat for many macrophytes. Many drains are strongly affected by eutrophication due to agricultural nutrient losses. The vegetation structure in moderately eutrophic drains is often characterized by a dominance of submerged vegetation, besides emergent species (helophytes) and often a phytoplankton bloom (De Groot et al. Citation1987). Further eutrophication associated with decreased light conditions causes a shift from species with a vertical growth strategy to those with a horizontal growth strategy and the species diversity diminishes (Janse and Van Puijenbroek Citation1998). At very high nutrient loading, the vegetation becomes dominated by a surface layer of floating plants such as Lemna gibba, Azolla sp., Eichhornia crassipes, and Pistia stratiotes, while submerged plants have disappeared (Ali et al. Citation2020).
Phytoremediation has been increasingly used to clean up contaminated soil and water systems because of its lower costs and fewer negative effects than physical or chemical engineering approaches (Mahujchariyawong and Ikeda Citation2001). It is considered an effective and environmental friendly technology for the remediation of excessive nutrients and heavy metals from the environment (Ali et al. Citation2020). Using vegetation makes the phytoremediation an appealing green technology for the removal of different nutrients from the environment (Eid et al. Citation2019). In order to make efficient phytoremediation, plants should have the following characteristics: native and quick growth rate, high biomass yield, the uptake of a large amount of nutrients, and the ability to transport nutrients in aboveground parts of plant (Burges et al. Citation2017). Aquatic plants act as a natural absorber for nutrients and heavy metals, which is the most proficient and profitable method (Guittonny-Philippe et al. Citation2015). Therefore, the selection of aquatic plant species for the accumulation of nutrients is a very important matter to enhance the phytoremediation (Galal et al. Citation2018). According to Yasar et al. (Citation2018), water lettuce (Pistia stratiotes) is an excellent contender for the phytoremediation of nutrients as it is more prone than other aquatic vegetation and has the capacity of reducing/removing nutrients from wastewater.
Excessive growth of aquatic plant biomass in eutrophic water bodies associated with nutrient-enriched agricultural land is widely reported around the world (Smith and Schindler Citation2009; Shaltout et al. Citation2010; Quilliam et al. Citation2015; Eid et al. Citation2020). To control eutrophication in receiving water bodies, biological nutrient removal has been widely used in wastewater treatment practices (Hu et al. Citation2012). Therefore, the present study was conducted to investigate the potential of the free floating macrophytes Pistia stratiotes to remediate nutrients and restore the eutrophic drain (Al-Sero Drain), South Nile Delta, Egypt.
Materials and methods
Study area
The study area () lies in Giza Province, South Nile Delta, Egypt. This southern region of the Nile Delta lies in a hyper-arid region (UNESCO Citation1977) with an annual rainfall of approximately 87 mm; mean annual maximum and minimum air temperatures of 30.0 °C and 14.8 °C, respectively; mean annual evaporation of 6.9 mm/day (Piche); mean annual relative humidity of 45.5%; and mean annual wind speed of 3.9 m/s (NASA-POWER Citation2020).
Study species
Pistia stratiotes Linnaeus (Araceae) (water lettuce) is a free-floating macrophyte that is capable of rapid vegetative propagation (Täckholm Citation1974). It was listed as an invasive species in the Global Invasive Species Database (Global Invasive Species Database (GISD)) Citation2020). P. stratiotes has a widespread distribution throughout tropical and subtropical regions, but it is absent in Antarctica (Chapman et al. Citation2017). It is one of the worst weeds in the world (Holm et al. Citation1991) and adversely affects the environment and biodiversity due to its capacity to form dense mats capable of blocking navigation channels, obstructing fishing and boat transport, impeding water flow in irrigation and flood control canals, and disrupting hydropower generation (Adebayo et al. Citation2011). However, P. stratiotes has been shown to have major potential for the management of water quality due to its ability to accumulate nutrients and heavy metals from wastewater (Galal and Farahat Citation2015; Galal et al. Citation2018). In Egypt, P. stratiotes grows in slow-flowing canals of the northern region of the Nile Delta, also growing in Embaba near Cairo (Boulos Citation2005) and in stagnant and calm waters, especially around Fariskur (Täckholm Citation1974). Recently, it has been recorded at several locations in Lake Mariut (Galal and Farahat Citation2015) and Lake Manzala (Galal et al. Citation2012) in the northern region of the Nile Delta. Pistia stratiotes is a fast-growing invasive macrophyte dominating the other plants (Eichorrnia crassipes and Lemna gibba) in drainage canals in the Nile Delta (Galal et al. Citation2018).
Field and laboratory
Sampling was performed in monospecific and homogeneous stands of P. stratiotes at three sites (Site 1: Lat. 30° 03′ 18.88′′ N, Long. 31° 08′ 17.56′′ E; Site 2: Lat. 30° 03′ 15.73′′ N, Long. 31° 08′ 28.20′′ E; Site 3: Lat. 30° 03′ 30.00′′ N, Long. 31° 08′ 14.00′′ E. Site 1 is located between Site 2 and 3, ∼ 400 m north of Site 2 and ∼ 400 m south of Site 3; ) along the Al-Sero Drain (as representative of drainage canals in South Nile Delta) in Giza Province. At each selected site, the biomass of P. stratiotes was sampled monthly from May 2013 to February 2014 using three randomly distributed quadrats (each 0.5 × 0.5 m) and all P. stratiotes individuals inside the quadrat were collected and placed in plastic bags and transferred to the laboratory. In the laboratory, samples were separated into shoots and roots, and carefully washed with tap water over a 4 mm mesh sieve to minimize material loss, oven dried at 85 °C to a constant weight, weighed, and then ground using a metal-free plastic mill. All biomass values were determined as g dry matter per square metre (g DM/m2).
Water sampling
At each sampling site, three water samples were collected monthly from the same sampling quadrats. The water samples were taken as integrated composite samples from the top of the water surface down to 20 cm. The samples were collected in 1,000 ml plastic bottles (which were washed with de-ionized water) and brought to the laboratory. Samples were acidified to a pH of 2.0 using sulfuric acid (Analar) to preserve the nutrients in the samples. After that, samples were deep-frozen for the analysis of total N, total P, Ca, Mg, Na and K.
Chemical analysis
For plant samples, P, Ca, Mg, Na and K were extracted from 0.5 to 1 g of plant organs (shoots and roots) using a mixed-acid digestion method (HNO3:HClO4:HF, 1:1:2, v:v:v). For plant and water samples, estimation of Ca, Mg, Na and K was carried out by atomic absorption (Shimadzu AA-6200). Molybdenum blue method was applied for the determination of total P using a spectrophotometer (CECIL CE 1021). Total N was determined in plant samples using a CHN Elemental Analyzer (Yanako CHN Corder MT-5 and Auto Sampler MTA-3). The indo-phenol blue method was used for determination of total N in water samples using a spectrophotometer (CECIL CE 1021). All these procedures are outlined in Allen (Citation1989) and American Public Health Association (APHA) (Citation1998). Nutrient concentrations were expressed based on the dried matter. Finally, the nutrient standing stocks of the shoots and roots (g/m2) were calculated by multiplying the nutrient concentrations by the biomass of the respective organ.
Statistical analysis
The P. stratiotes data, as well as the water nutrients data, did not differ significantly among the study sites along Al-Sero Drain (data not presented). Thus, the data of the three sites were combined, which resulted in nine quadrats for each sampling date. Before performing ANOVA, the data were evaluated for normality of distribution and homogeneity of variance using Shapiro-Wilk’s W test and Levene’s test, respectively, and when necessary, the data were log-transformed. The nutrient concentrations in the water were subjected to a repeated measure analysis of variance (ANOVA) to test the differences over time. The biomass and nutrient data for P. stratiotes organs were subjected to a two-way analysis of variance (ANOVA-2) to test the differences between organs over time. Correlations between the concentrations of nutrients in the plant organs and water samples were evaluated using Pearson’s r coefficient. All statistical analyses were performed using Statistica 7.1 software (Statsoft Citation2007).
Results
Water properties
The analysis of water nutrients indicated that there were monthly significant variations in the investigated elements (). The highest values of Ca and Mg (51.3 and 138.5 mg/l, respectively) were recorded in May, while the highest N, P, K and Na (39.3, 14.0, 22.3 and 258.1 mg/l) were recorded during November, February, December and January, respectively. It was clear that the nutrient elements’ concentration in the water were in the order: Na > Mg > Ca > N>K > P.
Figure 2. Monthly variation in the water nutrient concentrations (mg/l) in Al-Sero Drain (South Nile Delta, Egypt) supporting Pistia stratiotes populations. Vertical bars indicate the standard errors of the means (n = 9). F-values represent the repeated measures ANOVA. ***: p < 0.001, df: degrees of freedom.
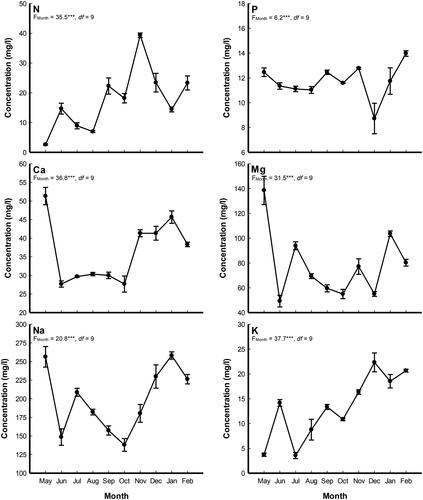
Biomass of P. stratiotes
The statistical analysis (ANOVA-2) showed significant variation in P. stratiotes biomass between the different plant organs along the time (). The plant biomass showed bell-shaped distribution, where the shoot and root had their lowest biomass (25.7 and 3.4 g DM/m2) during May and then they increase gradually until reach their peaks (314.8 and 43.6 g DM/m2) during September. After that they were gradually declined to reach their lower values (35.9 and 5.7 g DM/m2) during February.
Nutrient concentration
The nutrients analysis of the different organs of P. stratiotes showed that the plant shoots accumulated higher concentrations of most nutrient elements, except Mg, than the roots (). In the plant shoot, the highest concentrations of P and Mg (1.15 and 0.87%) were recorded in January. In addition, the highest N and Na concentrations (4.98 and 3.07%) were recorded during February and May, while the highest Ca and K (3.23 and 6.86%) were recorded during September. On the other side, the root of P. stratiotes accumulated the highest concentrations of N, P and K (3.36, 0.36 and 4.63%) were recorded during February, while the highest Mg and Na (1.99 and 2.37%) were recorded during May and Ca (2.03%) were attained in October. It is worth to note that the order of nutrients concentration in P. stratiotes shoot was: K > N > Ca > Na > Mg > P, while in the root was: K > N > Na > Ca > Mg > P.
Figure 4. Monthly variation in the nutrient concentrations (%) in shoot and root systems of Pistia stratiotes populations grown in Al-Sero Drain (South Nile Delta, Egypt). Vertical bars indicate the standard errors of the means (n = 9). F-values represent the two-way analysis of variance (ANOVA-2). ***: p < 0.001.
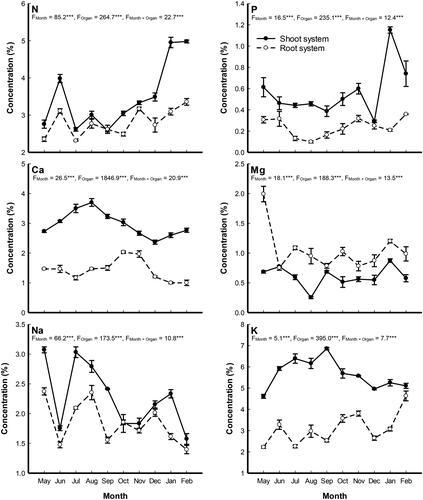
Nutrient standing stock
The nutrient standing stock (g/m2) data indicated that the tissues of P. stratiotes extracted higher nutrients content per its area from the study drain (). The plant shoots accumulated greater contents of the investigated nutrients than the root. Most nutrients content had its minimum values during May, reached its maximum during August - October and then decreased again to reach the lower during February. The plant shoot accumulated the highest contents of N and P (9.41 and 1.55 g/m2) during October, while the highest Mg and K (2.16 and 21.58 g/m2) during September, and Ca and Na (11.42 and 8.62 g/m2) during August. On the other side, the plant root accumulated the highest contents of N, P, Ca, Mg and K (1.14, 0.07, 0.65, 0.34 and 1.10 g/m2) during September, while the highest Na content (0.82 g/m2) during August. The annual averages of the nutrient standing stocks accumulated per unit area of P. stratiotes shoots ranked in the following order: K > N > Ca > Na > Mg > P, while that of the plant root was: K > N > Na > Ca > Mg > P.
Figure 5. Monthly variation in the nutrient standing stocks (g/m2) in shoot and root systems of Pistia stratiotes populations grown in Al-Sero Drain (South Nile Delta, Egypt). Vertical bars indicate the standard errors of the means (n = 9). F-values represent the two-way analysis of variance (ANOVA-2). ***: p < 0.001.
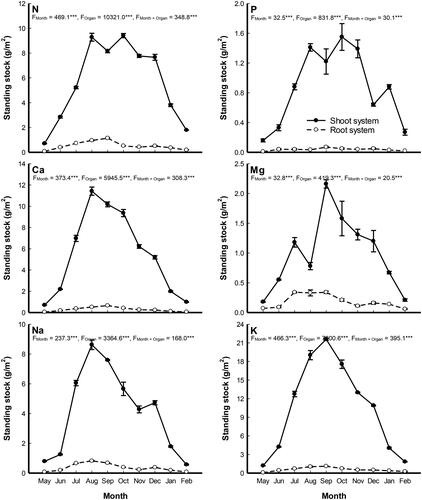
Plant-water correlations
The Pearson’s correlation coefficient (r-values, n = 90) showed significant correlation between the concentrations of some nutrient elements in the water and the different organs of P. stratiotes (). It was found that water N and K were significantly correlated (r = 0.398, p = 0.000; r = 0.248, p = 0.019, respectively) to the same elements in the plant root, while P and Na in the water were significantly correlated (r = 0.324, p = 0.002; r = 0.219, p = 0.038, respectively) to the corresponding elements in the plant shoot. Meanwhile, Mg had significant positive correlations (r = 0.724, p = 0.000; r = 0.282, p = 0.007, respectively) with the corresponding element in the plant root and shoot, respectively. On the contrary, significant negative correlation (r = −0.470, p = 0.000) was found between Ca in water and plant shoot.
Discussion
Water physical and chemical characteristics can be determined by the prevailing climatic, geomorphologic, and geochemical conditions in the drainage basin and the underlying aquifer (Eid et al. Citation2020). The high nutrient concentrations in Al-Sero drain resulted from domestic and agricultural drainage from the adjacent area (Dakhil et al. Citation2016). The highest values of Ca and Mg were recorded during May, while the highest N, P, K and Na were recorded during November, February, December, and January, respectively. This result indicate that eutrophication increased during winter rather than summer months and this in accordance with Galal et al. (Citation2019a) in the same study area and Eid et al. (Citation2020) on Phragmites australis in Lake Burullus (a Ramsar site in Egypt). Eutrophication increased during this season due to the excessive waste discharges during this period which brought high load of nutrients into the drain (Dakhil et al. Citation2016). The nutrient elements’ concentration in the water fell in the order: Na > Mg > Ca > N>K > P, (which is similar to that recorded in Burullus wetland (Eid et al. Citation2020) and in the main watercourses in Greater Cairo, Egypt (Ghazi et al. Citation2019).
In arid and semi-arid regions, the growth of aquatic macrophytes starts in spring, where the above-ground biomass reaches its maximum during summer and then decreases due to the beginning of senescence (Zhao et al. Citation2013). In the present study, the seasonal pattern of P. stratiotes biomass had a collapse phase during winter and explosive growth during late summer, which is similar to that of the same species in south Florida and Lake Volta in Ghana (Hall and Okali Citation1974; Dewald and Lounibos Citation1990); P. australis in Lake Burullus (Eid et al. Citation2020), Ludwigia stolonifera (Galal et al. Citation2019b); Vossia cuspidata and Cyperus articulatus (Ghazi et al. Citation2019); and Eichhornia crassipes (Shaltout et al. Citation2010; Eid et al. Citation2019) in the Egyptian watercourses. According to Eid et al. (Citation2019), low air temperature in winter is an important limiting factor determining the growth of floating macrophytes. Moreover, the highest shoot and root biomass was recorded during September, however it showed remarkable reduction in winter season which in accordance with Šajna et al. (Citation2007) and Galal et al. (Citation2019a). The high plant biomass recorded during September was associated with relatively high-water P and N concentrations. According to Maddison et al. (Citation2009), the increase in plant biomass may be attributed to the increase in nutrients, such as N and P in water. In addition, the maximum shoot biomass was recorded in September, which has a significant survival value because the plant can avoid the competition for light and nutrients with the other aquatic plants which have their peak growth in summer (Vossia cuspidata in August, Ghazi et al. Citation2019; T. domingensis in July, Eid et al. Citation2012; P. australis in August, Eid et al. Citation2010; E. crassipes in July, Eid et al. Citation2019).
The standing stock of nutrients depends on the concentrations of N and P in the plant tissue as well as on the amount of plant biomass (Maddison et al. Citation2009). In addition, macronutrient such as N, P and K are the most frequently to limit plant growth (Eid et al. Citation2020). The highest concentrations of N and P (49.8 and 11.5 g/kg) in the P. stratiotes shoots were recorded during January and February, respectively. These figures are higher than those recorded for P. stratiotes (17.0 and 3.0 g/kg) by Lu et al. (Citation2010) in the St. Lucie Estuary in Florida, and (15.0-40.0 and 4.0-10.0 g/kg) by Aoi and Hayashi (Citation1996). According to Irfan (Citation2014), the biomass and nutrient uptake of P. stratiotes was influenced by the mass of nutrient rate reaching to the plant rather than by its concentration, though, the critical nutrients concentration, water volume and plant surface area are also important for nutrient uptake (Galal et al. Citation2019a). The highest plant N and P concentration associated with lower biomass may be attributed to the utilization of these nutrients in building up plant biomass in the growing season during summer, while in the winter season they can be stored in the tissues. As reported by Irfan (Citation2014), the increase of plant N enhances plant biomass till maturity, while further increase only enhance the nitrogen storage in plant tissues. Therefore, nitrogen cannot be assigned as the single factor for plant growth and biomass yield. The reduction in dry biomass may be one of the common symptoms of heavy metal stress on the plant in Al-Sero drain (Galal et al. Citation2018). Moreover, sodium content is lower in P. stratiotes root than in the shoot, which is coincided with the results of Wasagu et al. (Citation2013). It is worth to note that the order of nutrients concentration in P. stratiotes shoot was: K > N > Ca > Na > Mg > P, while in the root was: K > N > Na > Ca > Mg > P. This result is coincided with Dakhil et al. (Citation2016) on P. stratiotes and Eid et al. (Citation2020) on P. australis.
Aquatic macrophytes play a crucial role by creating a favourable environment for a variety of complex chemical, biological, and physical processes that contribute to the removal and degradation of nutrients (Lu et al. Citation2010; Abdallah et al. Citation2020). Determination of the nutrient standing stock in wetland ecosystems requires information about the nutrient concentrations and biomass of the investigated plant, to calculate its nutrient standing stock per unit area (Eid et al. Citation2020). The nutrient standing stock (g/m2) of P. stratiotes had its minimum values during May, reached its maximum during August - October, and then decreased again to reach the lower during February. According to Dhir (Citation2013), N and P removal are temperature dependent as higher removal is noted in summer than winter. The highest nutrients content was associated with the highest biomass, although the nutrient concentrations declined throughout the growing season during winter, which indicates the decisive role of biomass in determining the quantity of nutrients per unit area of the plant (Eid and Shaltout Citation2014). In the present study, it was found that P. stratiotes had the potential to remove 9.3-9.4 g N/m2 and 1.4-1.6 g P/m2 if harvested during maximum biomass period (August-October). The nutrient storage capacity of P. stratiotes was generally high respecting the nutrient loadings in the surface water of Al-Sero drain, which is a potential factor affecting the nutrient storages of the study plant. Furthermore, the high nutrient content of P. stratiotes in Al-Sero drain supports its use as a “green filter” to mitigate the inflow of nutrients through harvesting the shoot biomass.
According to Ruiz and Velasco (Citation2010), the nutrients concentration of an aquatic plant increases with increasing its level in the environment, and this relationship depends mainly on both the species and the elements. The Pearson’s correlation coefficient showed that water N, Mg and K were significantly correlated to the same elements in the plant root, while P and Na in the water were significantly correlated to the corresponding elements in the plant shoot. These correlations indicate the cumulative effects of nutrients from Al-Sero Drain water, which provide quantitative assessment of the environmental quality (Galal et al. Citation2019a) that suggests the potential use of this plant as a biomonitor of nutrient levels in the eutrophic watercourses. According to Ghazi et al. (Citation2019), aquatic macrophytes have a crucial role in nutrient cycle in wetland ecosystems through their potential accumulation of nutrients and the high ability to sequester them in their tissues. The most efficient way to achieve this target is to harvest these macrophytes before their leaves reach senescence to avoid leaching of nutrients from the plant materials to the water. Moreover, the present study addresses an important issue related to the ideal time of harvesting P. stratiotes for remediating the maximum nutrient content from Al-Sero Drain. August-October harvest of P. stratiotes is the potential for remediating the highest nutrients from eutrophic watercourses. A positive side effect of removing P. stratiotes from eutrophic watercourses is a reduction in secondary pollution arising from plant decomposition and sedimentation (Eid and Shaltout Citation2014).
Conclusions
The seasonal pattern of P. stratiotes biomass had a collapse phase during winter and explosive growth during late summer. Phytoremediation can be an important approach for cleaning eutrophic stormwaters from agriculture and urban areas. P. stratiotes has a great potential for removing macronutrients, reducing water suspended solids and turbidity from stormwaters, and improving water quality. The nutrient standing stock of P. stratiotes reached its maximum during August - October, which is the potential period for remediating the highest nutrients from eutrophic watercourses. Thus, for efficient phytoremediation, grown-up biomass of P. stratiotes must be removed from watercourses to prevent returning of nutrients to the water through plant decomposition. Harvested plant biomass can be used as soil amendment, substrate for biogas production or could be processed into livestock feed.
Disclosure statement
No potential conflict of interest was reported by the authors.
Additional information
Funding
References
- Abdallah SM, Farahat EA, Shaltout KH, Eid EM. 2020. Assessing macro-nutrient removal potential of nine native plant species grown at a sewage sludge dump site. Appl Ecol Env Res. 18(1):1799–1817.
- Adebayo AA, Briski E, Briski E, Kalaci O, Hernandez MEP, Ghabooli S, Beric B, Chan FT, Zhan A, Fifield E, et al. 2011. Water hyacinth (Eichhornia crassipes) and water lettuce (Pistia stratiotes) in the Great Lakes: Playing with fire? AI. 6(1):91–91.
- Ali EM. 2011. Impact of drain water on water quality and eutrophication status of Lake Burullus, Egypt, a southern Mediterranean lagoon. Afr J Aquat Sci. 36(3):267–277.
- Ali S, Abbas Z, Rizwan M, Zaheer IE, Yava I, Ünay A, Abdel-Daim MM, Bin-Jumah M, Hasanuzzaman M, Kalderis D. 2020. Application of floating aquatic plants in phytoremediation of heavy metals polluted water: a review. Sustainability. 12(5):1927.
- Allen SE. 1989. Chemical analysis of ecological materials. London: Blackwell Scientific Publications.
- American Public Health Association (APHA). 1998. Standard methods for the examination of water and wastewater. Washington, DC: American Public Health Association.
- Aoi T, Hayashi T. 1996. Nutrient removal by water lettuce (Pistia stratiotes). Water Sci. Tech. 34(7-8):407–412.
- Boulos L. 2005. Flora of Egypt. Vol. 4 (Monocotyledons). Cairo: Al-Hadara Publishing.
- Burges A, Epelde L, Blanco F, Becerril JM, Garbisu C. 2017. Ecosystem services and plant physiological status during endophyte-assisted phytoremediation of metal contaminated soil. Sci. Total Environ. 584-585:329–338.
- Chapman D, Coetzee J, Hill M, Hussner A, Netherland M, Pescott O, Stiers I, van Valkenburg J, Tanner R. 2017. Pistia stratiotes L. EPPO Bull. 47:537–543.
- Costa JA, Souza JP, Teixeira AP, Nabout JC, Carneiro FM. 2018. Eutrophication in aquatic ecosystems: a scientometric study. Acta Limnol Bras. 30(0):e2.
- Dakhil MA, Galal TM, Hassan LM. 2016. Population dynamics and nutrient cycling of Pistia stratiotes L. Saarbrücken: LAP Lambert Academic Publishing.
- De Groot WT, De JF, Van den Berg MM. 1987. Population dynamics of duckweed cover in polder ditches. Arch. Hydrobioi. 109:601–618.
- Dewald LB, Lounibos LP. 1990. Seasonal growth of Pistia stratiotes in South Florida. Aquat. Bot. 36(3):263–275.
- Dhir B. 2013. Phytoremediation: role of aquatic plants in environmental clean-up. New Delhi-Heidelberg-New York-Dordrecht-London: Springer.
- Eid EM, Shaltout KH, Al-Sodany YM, Haroun SA, Galal TM, Ayed H, Khedher KM, Jensen K. 2020. Seasonal potential of Phragmites australis in nutrient removal to eliminate the eutrophication in Lake Burullus, Egypt. J Freshwater Ecol. 35(1):135–155.
- Eid EM, Shaltout KH, Al-Sodany YM, Soetaert K, Jensen K. 2010. Modeling growth, carbon allocation and nutrient budget of Phragmites australis in Lake Burullus, Egypt. Wetlands. 30(2):240–251.
- Eid EM, Shaltout KH, El-Sheikh MA, Asaeda T. 2012. Seasonal courses of nutrients and heavy metals in water, sediment and above- and below-ground Typha domingensis biomass in Lake Burullus (Egypt): perspective for phytoremediation. Flora. 207(11):783–794.
- Eid EM, Shaltout KH, Moghanm FS, Youssef MS, El-Mohsnawy E, Haroun SA. 2019. Bioaccumulation and translocation of nine heavy metals by Eichhornia crassipes in Nile Delta, Egypt: perspectives for phytoremediation. Int J Phytoremediat. 21(8):821–830.
- Eid EM, Shaltout KH. 2014. Monthly variations of trace elements accumulation and distribution in above- and below-ground biomass of Phragmites australis (Cav.) Trin. ex Steudel in Lake Burullus (Egypt): a biomonitoring application. Ecol Eng. 73:17–25.
- El-Amier YA, Elnaggar AA, El-Alfy MA. 2016. Investigation of eutrophication state of Manzala and Burullus Lakes in Egypt by using remote sensing and GIS. J Environ Sci Pollut Res. 2:121–125.
- Galal TM, Al-Sodany YM, Al-Yasi HM. 2019b. Phytostabilization as a phytoremediation strategy for mitigating water pollutants by the floating macrophyte Ludwigia stolonifera (Guill. & Perr.) P.H. Raven. Int J Phytoremediat. 22(4):373–382.
- Galal TM, Dakhil MA, Hassan LM, Eid EM. 2019a. Population dynamics of Pistia stratiotes L. Rend Fis Acc Lincei. 30(2):367–378.
- Galal TM, Eid EM, Dakhil MA, Hassan LM. 2018. Bioaccumulation and rhizofiltration potential of Pistia stratiotes L. for mitigating water pollution in the Egyptian wetlands. Int J Phytoremediat. 20(5):440–447.
- Galal TM, Farahat EA. 2015. The invasive macrophyte Pistia stratiotes L. as a bioindicator for water pollution in Lake Mariut. Egypt. Environ Monit Assess. 178(11):701.
- Galal TM, Shaltout KH, Hassan LM. 2012. The Egyptian northern lakes: habitat diversity, vegetation and economic importance. Saarbrücken: LAP Lambert Academic Publishing.
- Ghazi SM, Galal TM, Hussein KM. 2019. Monitoring water pollution in the Egyptian watercourses: a phytoremediation approach. Saarbrücken: LAP LAMBERT Academic Publishing.
- Global Invasive Species Database (GISD). 2020. Species profile: Pistia stratiotes. [accessed 30 April 2020]. http://www.iucngisd.org/gisd/speciesname/Pistia+stratiotes.
- Guittonny-Philippe A, Petit M-E, Masotti V, Monnier Y, Malleret L, Coulomb B, Combroux I, Baumberger T, Viglione J, Laffont-Schwob I. 2015. Selection of wild macrophytes for use in constructed wetlands for phytoremediation of contaminant mixtures. J Environ Manag. 147:108–123.
- Hall JB, Okali DU. 1974. Phenology and productivity of Pistia stratiotes L. on the Volta Lake, Ghana. J Appl Ecol. 11(2):709–725.
- Holm LG, Plucknett DL, Pancho JV, Herberger JP. 1991. The world's worst weeds: distribution and biology. Malabar: Krieger Publishing Co.
- Hu Z, Houweling D, Dold P. 2012. Biological nutrient removal in municipal wastewater treatment: new directions in sustainability. J Environ Eng. 138(3):307–3017.
- Irfan S. 2014. Nirogen uptake and storage by free-floating wetland species Pistia stratiotes L. J Selcuk Univ Nat Appl Sci. 3:1100–1114.
- Janse JH, Van Puijenbroek PJ. 1998. Effects of eutrophication in drainage ditches. Environ Pollut. 102(1):547–552.
- Lapointe BE, Herren LW, Debortoli DD, Vogel MA. 2015. Evidence of sewage-driven eutrophication and harmful algal blooms in Florida’s Indian River lagoon. Harm Algae. 43:82–102.
- Lu Q, He ZL, Graetz DA, Stoffella PJ, Yang X. 2010. Phytoremediation to remove nutrients and improve eutrophic storm waters using water lettuce (Pistia stratiotes L.). Environ Sci Pollut Res. 17(1):84–96.
- Maddison M, Soosaar K, Mauring T, Mander U. 2009. The biomass and nutrient and heavy metal content of cattails and reeds in wastewater treatment wetlands for the production of construction material in Estonia. Desalination. 247:121–129.
- Mahujchariyawong J, Ikeda S. 2001. Modelling of environmental phytoremediation in eutrophic river-the case of water hyacinth harvest in Tha-Chin River. Thailand. Ecol Model. 142(1–2):121–134.
- Meuleman AFM, Van Logtestijn R, Rijs GBJ, Verhoeven JTA. 2003. Water and mass budgets of a vertical-flow constructed wetland used for wastewater treatment. Ecol Eng. 20(1):31–44.
- NASA-POWER. 2020. Climatology resource for agroclimatology. NASA Prediction of Worldwide Energy. [accessed 28 April 2020]. http://power.larc.nasa.gov/cgi-bin/cgiwrap/solar/agro.cgi.
- O’Hare MT, Baattrup-Pedersen A, Baumgarte I, Freeman A, Gunn IDM, Lázár AN, Sinclair R, Wade AJ, Bowes MJ. 2018. Responses of aquatic plants to eutrophication in rivers: a revised conceptual model. Front Plant Sci. 9:451.
- Okbah MA. 2005. Nitrogen and phosphorus species of Lake Burullus water (Egypt). Egypt J Aquat Res. 31:186–198.
- Quilliam RS, van Niekerk MA, Chadwick DR, Cross P, Hanley N, Jones DL, Vinten AJ, Willby N, Oliver DM. 2015. Can macrophyte harvesting from eutrophic water close the loop on nutrient loss from agricultural land? J Environ Manag. 152:210–217.
- Reddy KR, De Busk WF. 1985. Nutrient removal potential of selected aquatic macrophytes. J Environ Qual. 14(4):459–462.
- Ruiz M, Velasco J. 2010. Nutrient bioaccumulation in Phragmites australis: management tool for reduction of pollution in the Mar Menor. Water Air Soil Pollut. 205(1–4):173–185.
- Šajna N, Haler M, Škornik S, Kaligarič M. 2007. Survival and expansion of Pistia stratiotes L. in a thermal stream in Slovenia. Aquat Bot. 87(1):75–79.
- Shaltout KH, Galal TM, El-Komi TM. 2010. Evaluation of nutrient status of some hydrophytes in the water courses of Nile Delta. Egypt Ecol Mid. 36:77–87.
- Shaltout KH, Galal TM, El-Komi TM. 2014. Biomass, nutrients and nutritive value of Persicaria salicifolia Willd in the water courses of Nile Delta. Rend Fis Acc Lincei. 25(2):167–179.
- Sinha E, Michalak AM, Balaji V. 2017. Eutrophication will increase during the 21st century as a result of precipitation changes. Science. 357(6349):405–408.
- Smith DR, Owens PR, Leytem AB, Warnemuende EA. 2007. Nutrient losses from manure and fertilizer applications as impacted by time to first runoff event. Environ Pollut. 147(1):131–137.
- Smith VH, Schindler DW. 2009. Eutrophication science: where do we go from here? Trend Ecol Evol. 24(4):201–207.
- Statsoft. 2007. Statistica version 7.1. Tulsa (OK): Statsoft Inc.
- Täckholm V. 1974. Students’ flora of Egypt. Beirut: Cooperative Printing Company.
- UNESCO. 1977. Map of the world distribution of arid regions. Paris: MAB Technical Notes.
- Wasagu RS, Lawal M, Shehu S, Alfa HH, Muhammad C. 2013. Nutritive values, mineral and antioxidant properties of Pista stratiotes (water lettuce). Nig J Basic Appl Sci. 21(257).
- Yamashita T, Yamamoto-Ikemoto R. 2014. Nitrogen and phosphorus removal from wastewater treatment plant effluent via bacterial sulfate reduction in an anoxic bioreactor packed with wood and iron. IJERPH. 11(9):9835–9853.
- Yasar A, Zaheer A, Tabinda AB, Khan M, Mahfooz Y, Rani S, Siddiqua A. 2018. Comparison of reed and water lettuce in constructed wetlands for wastewater treatment. Water Environ Res. 90(2):129–135.
- Zhang Y, Liu H, Yan S, Wen X, Qin H, Wang Z, Zhang Z. 2019. Phosphorus removal from the hyper-eutrophic Lake Caohai (China) with large-scale water hyacinth cultivation. Environ Sci Pollut Res. 26(13):12975–12984.
- Zhao Y, Xia X, Yang Z. 2013. Growth and nutrient accumulation of Phragmites australis in relation to water level variation and nutrient loadings in a shallow lake. J Environ Sci. 25(1):16–25.