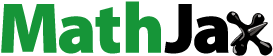
Abstract
This study investigated the zooplankton community structure in Ulungur Lake, located in the arid region of Northwest China, which has been slightly polluted by exogeneous sources. A total of 44 zooplankton species were identified in Ulungur Lake, which were sorted into seven functional groups: protozoan filter feeders (PF), rotifer filter feeders (RF), rotifer carnivores (RC), small copepod and cladoceran filter feeders (SCF), mid-sized copepod and cladoceran filter feeders (MCF), mid-sized copepod and cladoceran carnivores (MCC), and large copepod and cladoceran filter feeders (LCF). The dominant zooplankton functional group varied according to seasonal change. The RF group was dominant in the spring, comprising 87.77% of the total biomass. In the summer, the dominant group was SCF, comprising 90.72% of the total biomass, while the RF group accounted for only 7.24%. In the autumn, the biomass was mainly distributed between the RC (36.38%), SCF (30.62%), and RF (30.06%) groups. This seasonal difference in zooplankton community structure is related to fish production in Ulungur Lake, as well as to the competition and predation relationship among zooplankton species. This study showed a significant positive correlation between the PF and LCF groups, and between the RC, MCC, and MCF groups. This positive correlation was found to be related to the competition and predatory relationships between these functional groups. The predation pressure within the zooplankton functional groups in Ulungur Lake was not as significant as the pressure of food competition. The zooplankton biomass showed no significant difference between spring (0.0615 mg/L) and summer (0.0621 mg/L), but the biomass in autumn (0.3051 mg/L) was significantly increased. Nitrite-nitrogen (NO2-N) and Secchi Depth (SD) had significant correlations between species and functional groups, and were the major driving factors affecting the community structure of Ulungur Lake. In this study, the seasonal variations in the biomass of zooplankton were strongly influenced by environmental factors, which played an important driving role in structuring the ecological communities of zooplankton.
1. Introduction
Ulungur Lake, located in the inland Xinjiang Uygur Autonomous Region of China, is one of the largest 10 freshwater lakes in China. This region experiences low rainfall and intense evaporation, resulting in a dry climate and a shortage of water resources. Ulungur Lake and many other closed inland lakes in arid areas of northwest China have been polluted by exogenous sources such as natural environmental factors and long-term human activities (Hilati, Citation2021).
By searching relevant records, we found that crustaceans and rotifers of zooplankton in Ulungur Lake have been recorded (Yang et al. Citation2011); however, there is no research on the zooplankton community structure itself. Elements of the plankton community structure, such as species composition and biodiversity, directly or indirectly affect the aquatic ecosystem (Einsle Citation1983; Norros et al. Citation2017; McQuatters-Gollop et al. Citation2019). As primary and secondary consumers, zooplankton are consumed by fish and other aquatic organisms. Zooplankton play an important and fundamental role in the transfer of matter and energy in the aquatic food web (Caroni and Irvine Citation2010; Du et al. Citation2015; García-Chicote et al. Citation2018; Gomes et al. Citation2020). To study the plankton community structure, we used a method of grouping structurally and behaviorally similar plankton within an ecosystem into a functional group. This classification method accurately reflects the correlation between plankton communities and aquatic environmental factors across different waters (Peña Citation2003; Hood et al. Citation2006). Functional groups of organisms, classified by their role in the ecosystem, are actively used in various areas of community ecology. In this study, we investigated the community structure of zooplankton in Ulungur Lake by classifying functional groups and analyzing the main environmental driving factors within them. Results of this study provide a reference for research into the prevention of the further deterioration of the lake water ecological environment of Ulungur Lake.
2. Materials and methods
2.1. Study area
Ulungur Lake (46°59′–47°25′N, 87°1′–87°35′E) is located in Fuhai County, Altay Prefecture, of the Xinjiang Uygur Autonomous Region in China. Ulungur Lake is a sag pond located in the northern Junggar Basin at an altitude of 478 m. The total area of the lake is 1,027.6 km2 and consists of two parts: Buluntuohai Lake (858.9 km2) and Jili Lake (168.7 km2). The two parts are connected by a river that is approximately 7 km long, which forms a connected aquatic ecosystem. Ulungur Lake has two inflows and no outflows. The Ulungur River flows into the Jili Lake, and the Irtysh River flows into the Buluntuohai Lake. Ulungur Lake (mean depth 15 m) is one of China’s most northwestern desert lakes and is one of the most important fishing grounds in the region. Comparing with other phytoplankton, Chlorophyta is the major composition of the species and also the major amount of phytoplankton, followed by Bacillariophyta (Li et al. Citation2008; Liu et al. Citation2009). The indigenous fish species of Ulungur Lake dominated by the common perch, Perca fluviatilis and Leuciscus dzungaricus. After the intentional and unintentional introduction of alien species, Hypomesus olidus, Abramis brama and Esox lucius have been introduced and became the most prevalent species (Tang et al. Citation2012). The aquatic macrophyte of the lake is mainly Phragmites australis and Potamogeton spp. (Li and Yang Citation1993; Ye et al. Citation2004).
In 2019, a total of 28 sites were selected evenly across the lake for this study. These were sampled during spring, summer, and autumn. Sites 1–22 are located in Buluntuohai Lake and sites 23–28 in Jili Lake ().
2.2. Methods of sample collection and treatment
Protozoan and rotifer samples were collected using a Schindler sampler at 0.5 m below the lake surface and 0.5 m above the bottom of the lake at each sampling point, and mixed into 1-L water samples. The samples were preserved with Lugol’s iodine, and precipitated for 48 h by precipitation method. Next, the supernatant was removed by siphoning and 30 mL of concentrated solution was retained (Huang Citation1981).
Cladopoda and copepod samples were collected 20-L using a Schindler sampler at 0.5 m below the lake surface and 0.5 m above the lake bottom at each sampling point and mixed then into 20-L water samples. The samples were then filtered with a plankton net (64-mm mesh) and preserved with formaldehyde solution (4% concentration). We also precipitated the samples for 48 h, removed the supernatant by siphoning, and then retained 30 mL of concentrate. In the laboratory, we used a fluorescence microscope (BX-51, OLYMPUS, Tokyo, Japan) to identify species and record the number of zooplankton in the samples. We used the wet-weight method was used to evaluate zooplankton biomass, which was expressed as the number of individuals per liter (ind L−1) (Zuo et al. Citation2003).
2.3. Water environmental factors
In this study, water temperature (WT) and pH were measured using a Professional YSI multiprobe (YSI Inc., USA) during sampling, Secchi Depth (SD) was measured using a Secchi disk, and depth (D) was measured using a water depth gauge (Uwitec, Austria). In the laboratory, total phosphorus (TP), total nitrogen (TN), ammonium‑nitrogen (NH4-N), nitrate‑nitrogen (NO3-N), nitrite‑nitrogen (NO2−-N), permanganate index (CODMn), and chlorophyll-a (Chl. a) were measured according to the standard methods for China (Ministry of Environmental Protection (MEP) Citation2002).
2.4. Comprehensive trophic level index (TLI) method
TLI is a comprehensive eutrophication evaluation methods taking Chl. a, TP, TN, SD, and CODMn as the evaluation indicators (Li et al. Citation2015; Wang et al. Citation2019b). The TLI model is as follows:
(1)
(1)
where TLI (Ʃ) is comprehensive trophic level index, TLI (j) represents trophic state index of parameter j, Wj represents the corresponding weight of parameter j, m represents the numbers of evaluation parameters.
Take Chl-a as the benchmark parameter, the normalized correlation weight of parameter j is as follows:
(2)
(2)
where Wj represents the corresponding weight of parameter j. rij represents the correlation coefficient between benchmark parameter (Chl. a) and parameter j. m is the numbers of evaluation parameters.
Trophic level indexes of each parameter are calculated as EquationEquations (3)-(7).
(3)
(3)
(4)
(4)
(5)
(5)
(6)
(6)
(7)
(7)
Based on a water eutrophication survey of 26 Chinese lakes, the reference value of correlation coefficients rij is as follows: rChl. a = 1, rTN = 0.82, rTP = 0.84, rSD = 0.83, rCODMn = 0.83 [28,32]. Units are as follows: Chl. a (μg/L), TP (mg/L), TN (mg/L), CODMn (mg/L), and SD (m).
The trophic state of the lakes is assigned as follows: TLI (Ʃ) scores of <30, oligotrophic; TLI (Ʃ) scores of 30 to 50, mesotrophic; TLI (Ʃ) scores of 50 to 60, light eutrophic; TLI (Ʃ) scores of 60 to 70, medium eutrophic; TLI (Ʃ) scores of >70, severe eutrophic (Percent et al. Citation2008).
2.5. Statistical analyses
SPSS 24.0 was used to determine the seasonal variation of water environmental factors using a one-way analysis of variance (ANOVA), and was used to determine the correlation between the zooplankton functional groups by a Pearson correlation coefficient (PCC) analysis.
ArcGIS 10.3 was used to show general trends of the zooplankton biomass by using an inverse distance weighted (IDW) analysis (Pflaumann et al. Citation1996; Liu and Wang Citation2001).
Canoco 5.0 software was used for redundancy analysis (RDA) to analyze the relationship between the zooplankton community and water environmental factors (Šmilauer and Lepš Citation2014).
3. Results
3.1. Seasonal variation of water environmental factors
Water environmental factors in Ulungur Lake are shown in . A one-way ANOVA test of the environmental factors in Buluntuohai Lake revealed that all factors had significant seasonal variation, with the exception of D (P > 0.05) and TN (P > 0.05). A one-way ANOVA test of the environmental factors in Jili Lake revealed that all factors had significant seasonal variation, with the exception of D (P > 0.05) and ammonia‑nitrogen (NH4+-N) (P > 0.05). The mean values of SD, pH, and TN were higher in Buluntuohai Lake than in Jili Lake. On the contrary, the mean values of NO2−-N and TP were higher in Jili Lake than in Buluntuohai Lake. In both lakes, the mean value of CODMn was the highest in autumn, followed by spring and summer, whereas NO3-N showed a decreasing trend from spring to autumn.
Table 1. The seasonal variations of water environmental factors in Ulungur Lake.
TLI indicated that trophic states of Ulungur Lake was mesotrophic (). The TLI of Buluntuohai Lake in autumn was significantly higher than that in spring and summer, and the TLI of Jili Lake was higher in summer and autumn than in spring.
3.2. Species composition and seasonal variation of zooplankton
In total, 44 zooplankton species belonging to five taxonomic categories were identified during the study conducted in the spring, summer, and autumn of 2019 in Ulungur Lake. This included 14 rotifers, which accounted for 31.82 percent of the total zooplankton species, 12 protozoa (27.27%), 10 copepods (22.73%), 7 cladocerans (15.91), and 1 ostracode (2.27%) (). Twenty-nine species were recorded in autumn, followed by 16 species recorded in spring and 12 species recorded in summer. In Buluntuohai Lake, a total of 42 zooplankton species belonging to five taxonomic categories were identified. There were 12 protozoa, 12 rotifers, 10 copepods, 7 cladocerans, and 1 ostracoda found. Fewer species were found in Jili Lake. A total of 22 zooplankton species belonging to four taxonomic categories were identified, including 5 protozoa, 10 rotifers, 5 copepods and 2 cladocerans.
The dominant zooplankton species showed significant seasonal variation. Rotifers, especially Keratella quadrata and Brachionus angularia, were more abundant in the spring; K. quadrata were abundant in both Buluntuohai Lake and Jili Lake at this time of year. In the summer, cladocerans and copepods were more dominant than protozoa and rotifers. Bosmina coregoni and Sinocalanus dorii were found in large numbers in summer and autumn in both Buluntuohai Lake and Jili Lake. Ostracodes were only found in Buluntuohai Lake in the spring. There was a significant difference between the seasonal zooplankton abundance in Ulungur Lake. The zooplankton abundance was significantly highest in autumn (239.27 ind./L), followed by spring (48.76 ind./L) and summer (17.25 ind./L). The zooplankton biomass in Ulungur Lake was not significantly different in spring (0.0615 mg/L) versus summer (0.0621 mg/L), but the biomass in autumn (0.3051 mg/L) was significantly higher than at any other time of year. The zooplankton abundance in spring was higher than that in summer, but the biomass in spring and summer was not significantly different. The reason is that rotifers had a greater advantage in spring, cladocerans had a greater advantage in summer, and cladocerans are larger in size than rotifers.
Inverse distance weighting was plotted for the zooplankton biomass in Ulungur Lake, which showed that the biomass of zooplankton in Jili Lake was higher than that of Buluntuohai Lake in spring and summer; however, the differences were relatively small in autumn (). In Buluntuohai Lake, zooplankton were more abundant in the western part than the eastern part of the lake in spring and summer. In autumn, the zooplankton biomass in both the western and eastern parts was higher than that in the center of the lake. The zooplankton biomass in both Buluntuohai Lake and Jili Lake increased significantly in autumn.
3.3. Zooplankton functional groups
Zooplankton species in Ulungur Lake were classified into seven functional groups according to body size, length, and feeding mode (Benedetti et al. Citation2016; Ma et al. Citation2019). These seven functional groups were protozoan filter feeders (PF), rotifer filter feeders (RF), rotifer carnivores (RC), small copepod and cladoceran filter feeders (SCF), mid-sized copepod and cladoceran filter feeders (MCF), mid-sized copepod and cladoceran carnivores (MCC), and large copepod and cladoceran filter feeders (LCF) (). Abundance and biomass of zooplankton functional group can be seen in .
Table 2. Classification of zooplankton functional groups of Ulungur Lake.
Table 3. Abundance and biomass of zooplankton functional group of Ulungur Lake.
The structure of the zooplankton functional groups in the spring and summer was relatively simple in Ulungur Lake. However, in the autumn, the structure of the zooplankton functional groups was complex and diversified (, ). The RF group (87.77% biomass contribution), mainly including K. quadrata and B. angularia, was dominant in spring. In summer, the SCF group (90.72%), primarily Bosmina sp. and Nitocra sp., became the most dominant functional group, whereas the RF group (7.24%). However, RC (36.38%), SCF (30.62%) and RF (30.06%) had average advantages in autumn. Group RC mainly included Polyarthra trigla and Trichocerca pusilla. The SCF group mainly included Bosmina coregoni. The RF group mainly included Keratella cochlearis and K. quadrata.
Figure 4. Relative biomass of zooplankton functional groups in Ulungur Lake (a), and in its two constituent parts, Buluntuohai Lake (b) and Jili Lake (c).
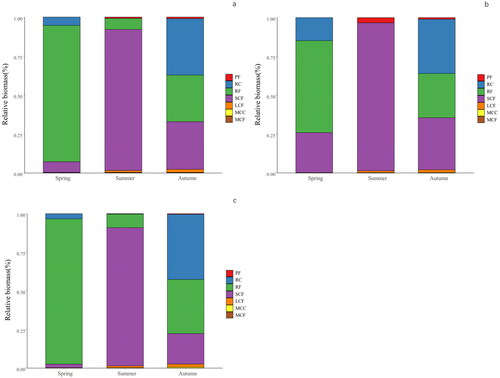
The functional group structure of zooplankton in Buluntuohai Lake was more diverse than that of zooplankton in Jili Lake. In the spring, RF (94.19%) was most abundant in Jili Lake, mainly including K. quadrata and B. angularia. In summer, SCF was dominant in both Buluntuohai Lake (95.42%) and Jili Lake (89.65%). Bosmina sp. was abundant in both lakes, and Nitocra sp. was abundant in Buluntuohai Lake. In autumn, the difference in biomass between the two lakes was insignificant. It can be seen from that the biomass of zooplankton in autumn was significantly higher than that in spring and summer in Buluntuohai Lake, however the seasonal variation of the biomass in Jili Lake was not significant.
Figure 5. Distribution of zooplankton functional groups biomass of Buluntuohai Lake (a) and Jili Lake (b) in Ulungur Lake.
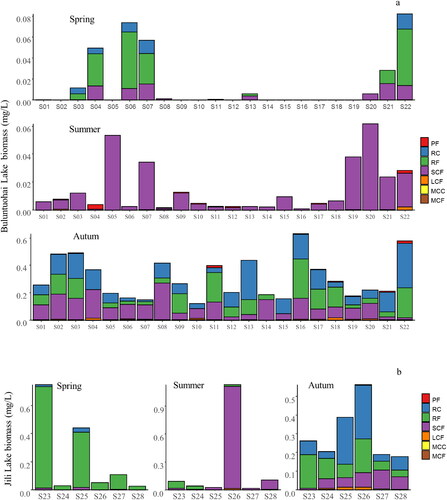
Figure 6. Ordination biplot of redundancy analysis (RDA) between dominant species of zooplankton and water environmental factors (a), and that between zooplankton functional groups and water environmental factors (b).
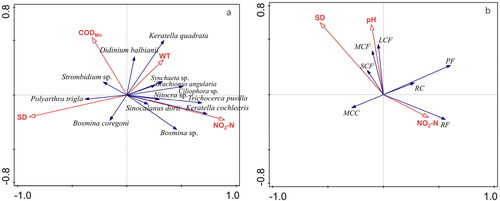
The results of the Pearson correlation coefficient test between the zooplankton functional group biomasses are shown in . There was a significant positive correlation between PF and LCF. The RC, MCC, and MCF groups were also positively correlated. This positive correlation was related to the competition and predatory relationships between these functional groups.
Table 4. Significant Pearson correlation coefficient between the biomass of zooplankton functional groups.
3.4. Relationship between zooplankton and water environmental factors
We used an RDA to explore the relationship between the dominant species of zooplankton and water environmental factors, and the relationship between zooplankton functional groups and water environmental factors .
The RDA showed that the best model included NO2−N, SD, CODMn, and WT as explanatory variables to interpret dominant species of zooplankton . Significant axes 1 and 2 explained 27.44% of the variation of the zooplankton dominant species assemblages (P = 0.002, Monte-Carlo permutation test, 999 permutations). The first axis showed a strong positive correlation with NO2−N, and a strong negative correlation with SD. K. cochlearis and T. pusilla were strongly positively correlated with NO2-N. P. trigla was closely correlated with SD. K. quadrata was strongly positively correlated with WT.
RDA showed that the best model included SD, pH and NO2−N as explanatory variables to interpret zooplankton functional groups . Significant axes 1 and 2 explained 25.87% of the variation of zooplankton functional groups assemblages (P = 0.002, Monte-Carlo permutation test, 999 permutations). The second axis showed a strong positive correlation with pH. RF was strongly positively correlated with NO2−-N. LCF and MCF were highly correlated with pH. SCF was closely positively correlated with SD.
4. Discussion
4.1. Characteristics of zooplankton community structure and functional groups
The zooplankton community structure in Ulungur Lake had significant seasonal variation. Rotifers were more abundant in the spring, and cladocerans and copepods were more abundant in the summer, but there was not one clear dominant taxonomic category in the autumn. This seasonal community structure variation was mainly related to fish production and to competition and predation among zooplankton species in Ulungur Lake. Water temperature, nutrient availability, the bottom-up effect of phytoplankton, the top-down effect of fish, and interspecific competition are all essential factors affecting the growth and characteristics of zooplankton functional groups (Srifa Citation2010; Ma et al. Citation2019).
The community structure of zooplankton can be understood by classifying species into functional groups. In the spring, the biomass of the RF functional group had a greater advantage than the other six groups. This group was mainly composed of low-temperature-resistant and filter-feeding rotifers such as K. quadrata, B. angularia, and K. cochlearis. The RF group feeds on phytoplankton, bacteria, and organic detritus. In the summer, the SCF group was the most dominant functional group, which was mainly composed of small crustaceans, such as Bosmina sp., Bosmina coregoni, and Nitocra sp., who feed on phytoplankton, bacteria, and organic detritus. The abundance of the SCF group increased, and SCF replaced RF to become the dominant functional group in summer as a result of the feeding pressure of fish on rotifers. Ulungur Lake is one of the most important inland fishery in China. There are many filter-feeding fishes feed on zooplankton including Aristichthys nobilis, Hypomesus olidus, Pseudorasbora parva, and so on (Adakbek et al. Citation2006). Among them, Hypomesus olidus being the dominant species because of its great fecundity (Tang et al. Citation2012; Zhou et al. Citation2013). Most of the fish are annual fish and spawn from January to April. The juveniles of the fish mostly feeds on rotifers (Zhao Citation1992), higher predation pressure of them leads to a decrease in the biomass of rotifers, which in turn decrease food competition with small crustaceans such as cladocerans and copepods in late spring and summer. The RF group presents the same feeding habits as the SCF group, therefore the dominance of the SCF group in turn reduced the abundance and biomass of the RF group in summer. The increase in SCF abundance could also be due to the fact that cladocerans and copepods had a significant positive correlation with water temperature. The ice on Ulungur Lake begins melting in late April, so spring water samples contained significantly colder water. With the increase of water temperature in summer, the biomass of cladocerans and copepods gradually increased. This is consistent with research from Yang et al. (Citation2011) who found Bosmina sp. had a greater advantage in the summer and autumn in Ulungur Lake. The predation by Hypomesus olidus feeding on cladocerans is significantly higher predation intensity in summer and autumn, especially in summer (Yang et al. Citation2011). Study shows under the high predation pressure of fish and invertebrates, many cladocerans are becoming more miniaturized to avoid predation (Michael Citation1988). This might be the reason why smaller Bosmina sp. showed a significantly predominance in summer and autumn. In the autumn, the abundance of the SCF group was reduced and the abundance of RC and RF grew. This was related to the value of SD, which showed a decreasing trend from the spring to autumn, and the possible reasons for this will be discussed below (Sec. 4.3).
The predation pressures within the zooplankton functional groups in Ulungur Lake are less significant than the pressure of food competition. The PCC test between the biomasses of zooplankton functional groups showed that groups RC, MCC, and MCF were significantly correlated. The RC group is carnivorous, preying on protozoa, other rotifers, and small crustaceans, which puts it in competition with MCC and MCF. In addition, there was a strong positive correlation between PF and LCF. The PF group is filter-feeding, feeding on phytoplankton, bacteria, and organic debris. LCF is carnivorous, feeding on phytoplankton, bacteria, organic debris, and protozoa. LCF can feed on protozoa in the PF group but the two groups are also in competition for food such as phytoplankton. In this study, among zooplankton functional groups, the pressure of food competition is more significant than that of predation.
4.2. The relationship between organic pollution and zooplankton biomass
In comparison with the spring and summer, the zooplankton biomass in Ulungur Lake, especially in Buluntuohai Lake, increased significantly in the autumn. This might be related to the local land-use such as land for fishery industry and planting industry of Ulungur Lake. These production activities lead to an increase in exogenous nutrients, which also promote the growth of zooplankton and affect the zooplankton biomass seasonally and regionally. Zooplankton community structure is strongly associated with the nutritional status of the water body. This can be seen in how nutrient enrichment impacts zooplankton community structure characteristics (Conde-Porcuna et al. Citation2002; Hietala et al. Citation2004; Zhang et al. Citation2010).
To increase the size and sustainability of the catch, local government has imposed fishing ban periods in Ulungu Lake from April to July every year. Therefore after July, fishery production activities increased in the western lakeshore areas of Buluntuohai Lake (CODMn and TN increased in sample point 2–4 and sample point 22), and eastern lakeshore areas of Jili Lake (TP increased in sample point 26), which enriches nutrients and leads to higher zooplankton biomass in these areas in the autumn. Allowing fishing in autumn also reduced the feeding pressure of fish on zooplankton to a certain extent. Moreover, higher biomass in the eastern lakeshore areas of Buluntuohai Lake (CODMn and TN increased in sample point 11 and sample point 16–17) might due to exogenous pollution from nearby farmland. The above results are consistent with the research of Ulungu Lake water quality in 2017 from Zou et al. (Citation2021). In addition to exogenous pollution source, another source of nutrients might release from water sediments in autumn. These pollutants reached the maximum release amount in the autumn after the WT rose under the strong sunshine in the summer in Xinjiang. Studies have shown that TP in the water body of Ulungur Lake had a significant positive correlation with the TP and the inorganic phosphorus (P) in the sediments (Zhu et al. Citation2005; Wang et al. Citation2019a). Similarly, CODMn might be derived from the discharge of organic pollutants in the catchment area or the release of organic pollutants in the sediment of the lake (Zhu et al. Citation2005). The pollutants in the sediment of Ulungur Lake mainly come from external input such as runoff from agricultural land, livestock, poultry breeding, aquaculture, and domestic sewage (Mischke and Zhang Citation2011). This was also the main reason for the increase in nutrients of the Ulungur Lake water ecosystem in the autumn. For fishery management and environmental protection, it should be a priority to ensure that the amount of pollutants entering the lake is reduced.
4.3. Environmental driving factors of zooplankton community
The RDA showed that NO2−N, SD, CODMn, and WT had significant correlations with the dominant species of the lake, whereas NO2−N, SD, and pH were more significantly correlated with zooplankton functional groups. In contrast, rotifers were highly affected by environmental influences. From the RF group, K. cochlearis and T. pusilla were closely related to NO2−N. In addition, there was a significant correlation between RF and NO2−N. K. cochlearis was found in Ulungur Lake in all seasons and shared the same seasonal trend as that of NO2−N, which gradually increased from the spring to autumn. As a result, K. cochlearis became the dominant species until the autumn. Nutrients such as nitrogen and phosphorus generally affect the abundance of zooplankton indirectly by affecting the growth of phytoplankton (Smith et al. Citation2016; Fanny et al. Citation2021). The Chl. a increase is relevant to the primary production. Under the control of the seasonal increased nutrients such as NO2−N, CODMn and NH4+-N, Chl. a showed a gradual increase from spring to autumn in Buluntuohai Lake, which had the same seasonal trends with K. cochlearis mainly feeding on planktonic algae. NO2−N can indirectly affect the growth of filter-feeding rotifers that obtain food sources from the bottom-up effect of phytoplankton, through effect on phytoplankton community (Martino et al. Citation2012; Wan et al. Citation2021).
SD was also an important water environmental factor for zooplankton species and the functional groups, especially for P. trigla and the SCF group. SD showed a decreasing trend from the spring to autumn, as the lake became more turbid. The SCF group is filter-feeding, and phytoplankton is its essential food source, but lower transparency inhibited photosynthesis of phytoplankton, and suspended solids could block filter-feeding organs, which contributed to the diminishing trend in the SCF group’s dominance in the autumn. This reduction of SCF dominance indirectly led to the increase in P. trigla biomass which competed with SCF for food. This is because P. trigla feeds mainly on phytoplankton, such as golden algae, cryptophytes, and diatoms.
5 Conclusions
In this study, a total of 44 zooplankton species belonging to five taxonomic categories were identified in Ulungur Lake. These species were categorized into seven functional groups: protozoan filter feeders (PF), rotifer filter feeders (RF), rotifer carnivores (RC), small copepod and cladoceran filter feeders (SCF), mid-sized copepod and cladoceran filter feeders (MCF), mid-sized copepod and cladoceran carnivores (MCC), and large copepod and cladoceran filter feeders (LCF). The RF group was dominant in the spring, SCF was dominant in the summer, and groups RC, SCF, RF were relatively equal in abundance in the autumn. This seasonal community structure difference is mainly related to fish production in Ulungur Lake, but it is also related to the competition and predation relationships among zooplankton species.
There was a significant positive correlation between the PF and LCF groups and between the RC, MCC, and MCF groups. The pressure of food competition was more significant than predation between the zooplankton functional groups.
There was more zooplankton biomass in the autumn in Ulungur Lake due to the increase of biochemical organic pollutants caused by the local land-use such as land for fishery industry and planting industry, and also released from water sediments during that time of year.
NO2-N and SD showed significant correlations between zooplankton species and functional groups and were the major driving factors affecting the zooplankton community structure of Ulungur Lake. K. cochlearis and T. pusilla, which were classified into RF, were correlated with NO2-N. P. trigla and the SCF group were more correlated with SD.
In this study, the seasonal variations in the biomass of zooplankton were heavily influenced by environmental factors, which played an important driving role in structuring the zooplankton ecological communities in Ulungur Lake.
Acknowledgments
This work was financially supported by the National Key Research and Development Program of China (2019YFD0900605 and 2019YFD0900602) and the Finance Special Fund of Ministry of Agriculture and Rural Affairs (Fisheries Resources and Environment Survey in the Key Water Areas of Northwest China).
Disclosure statement
No potential conflict of interest was reported by the authors.
Data availability statement
The data that support the findings of this study are available on request from the corresponding author [TH].
References
- Adakbek K, Su D-X, Yang Y, Ye S-M, Huderat. 2006. The current situation and the countermeasures of protection and development of fish resources in Ulungur Lake. J Shanghai Fish Univ. 15(3):308–314.
- Benedetti F,Gasparini S,Ayata S D. 2016. Identifying copepod functional groups from species functional traits. J Plankton Res. 38(1):159–166.
- Caroni R, Irvine K. 2010. The potential of zooplankton communities for ecological assessment of lakes: redundant concept or political oversight? Biol Environ Proc R Irish Acad. 110(1):35–53.
- Conde-Porcuna JM, Ramos-Rodríguez E, Pérez-Martínez C. 2002. Correlations between nutrient concentrations and zooplankton populations in a mesotrophic reservoir. Freshw Biol. 47(8):1463–1473.
- Du X, García-Berthou E, Wang Q, Liu J, Zhang T, Li Z. 2015. Analyzing the importance of top-down and bottom-up controls in food webs of Chinese lakes through structural equation modeling. Aquat Ecol. 49(2):199–210.
- Einsle U. 1983. Long-term changes in planktonic associations of crustaceans in Lake Constance and adjacent waters and their effects on competitive situations. Hydrobiologia. 106(2):127–134.
- Fanny C, Pascal R, Mark DO. 2021. On the sensitivity of plankton ecosystem models to the formulation of zooplankton grazing. PLoS One. 16(5).
- García-Chicote J, Armengol X, Rojo C. 2018. Zooplankton abundance: A neglected key element in the evaluation of reservoir water quality. Limnologica. 69:46–54.
- Gomes ACAM, Gomes LF, Roitman I, Pereira HR, Junior AFC, Costa EMM, da Silva MLC, da Jacobson TKB, Ribeiro RJ, da C, et al. 2020. Forest cover influences zooplanktonic communities in Amazonian streams. Aquat Ecol. 54(4):1067–1078.
- Hietala J, Vakkilainen K, Kairesalo T. 2004. Community resistance and change to nutrient enrichment and fish manipulation in a vegetated lake littoral. Freshw Biol. 49(12):1525–1537.
- Hilati A, Peng X-W, Liu X-W, Zhou D-K, Lu W-Z, Xie J-B.2021. Study on the Countermeasures of Water Ecological Environment Protection of Ulungur Lake in Xinjiang. Environment Protection of Xinjiang. 43(2):15–21.
- Hood RR, Laws EA, Armstrong RA, Bates NR, Brown CW, Carlson CA, Chai F, Doney SC, Falkowski PG, Feely RA, et al. 2006. Pelagic functional group modeling: progress, challenges and prospects. Deep Sea Res II. 53(5–7):459–512.
- Huang X. 1981. Application of the simplified method of weight determination to various species of planktonic rotifers in Lake Donghu, Wuhan. Acta Hydrobiol Sin. 7(3):409–416.
- Li W-C, Yang Q-X. 1993. Aquatic vegetation of Ulungur Lake. Oceanol Limnol Sin. 24(1):100–108.
- Li Z, Jiang Z-F, Zhao W-G, Ma B, Huo T-B, Guo Y, Cai L-G, Tang F-J. 2008. Phytoplankton population structure in Ulungur Lake. Chin J Fish. 21(2):15–20.
- Li Z-Z, Li X-D, Li F, Gao Z, Yan W, Liang J, Zeng G. 2015. Improved assessment model for comprehensive trophic state index based on dynamic cluster analysis and blind theory. Chin J Environ Eng. 9(4):2021–2026.
- Liu R-M, Wang X-J. 2001. The theory and method of the spatial optimal estimation on the water quality parameters of lake. China Environ Sci. 21(2):177–179.
- Liu Y, Shen J-Z, Li H, Zhao Y-J, Ma X-F, Liu Q-G, Jiang M, Dong Y, Liu J, Hao Z-C. 2009. Phytoplankton community structure in Lake Ulungur in Xinjiang Uygur Autonomous Region. J Lake Sci. 21(6):855–864.
- Ma C-X, Mwagona PC, Yu H-X, Sun X-W, Liang L-Q, Mahboob S, Al-Ghanim KA. 2019. Seasonal dynamics of zooplankton functional group and its relationship with physico-chemical variables in high turbid nutrient-rich Small Xingkai Wetland Lake, Northeast China. J Freshw Ecol. 34(1):65–79.
- Martino EM, Sean RC, Kirsten H. 2012. Nitrate–nitrite dynamics and phytoplankton growth. Limnol Oceanogr. 57(5):1555–1571.
- McQuatters-Gollop A, Atkinson A, Aubert A, Bedford J, Best M, Bresnan E, Cook K, Devlin M, Gowen R, Johns DG, et al. 2019. Plankton lifeforms as a biodiversity indicator for regional-scale assessment of pelagic habitats for policy. Ecol Indic. 101(1):913–925.
- Michael JV. 1988. Freshwater zooplankton community structure: Introduction of large invertebrate predators and large herbivores to a small species community. Can J Fish AquatSci. 45(10):1758–1770.
- Ministry of Environmental Protection (MEP), China. 2002. China’s national standard: GB3838-2002: Environmental quality standards for surface water. Beijing: Ministry of Environmental Protection.
- Mischke S, Zhang C-J. 2011. Ostracod distribution in Ulungur Lake (Xinjiang, China) and a reassessed Holocene record. Ecol Res. 26(1):133–145.
- Norros V, Laine M, Lignell R, Thingstad F. 2017. Parameterization of aquatic ecosystem functioning and its natural variation: Hierarchical Bayesian modelling of plankton food web dynamics. J Mar Syst. 174(1):40–53.
- Peña MA. 2003. Plankton size classes, functional groups and ecosystem dynamics: an introduction. Prog Oceanogr. 57(3–4):239.
- Percent SF, Frischer ME, Vescio PA, Duffy EB, Milano V, McLellan M, Stevens BM, Boylen CW, Nierzwicki-Bauer SA. 2008. Bacterial community structure of acid-impacted lakes: what controls diversity? Appl Environ Microbiol. 74(6):1856–1868.
- Pflaumann U, Duprat J, Pujol C, Labeyri LD. 1996. SIMMAX: A modern analog technique to deduce Atlantic sea surface temperatures from planktonic foraminifera in deep-Sea sediments. Paleoceanography. 11(1):15–35.
- Šmilauer P, Lepš J. 2014. Multivariate analysis of ecological data using Canoco 5. Boston: Cambridge University Press. p. 1–362.
- Smith SL, Vallina SM, Merico A. 2016. Phytoplankton size-diversity mediates an emergent trade-off in ecosystem functioning for rare versus frequent disturbances. Sci Rep. 6(1824):34170.
- Srifa A. 2010. Factors controlling zooplankton dynamics in a subtropical lake during cyanobacterial bloom events [thesis]. Gainesville (FL): University of Florida.
- Tang F-J, Brown A, Keerjiang A. 2012. Fish community successions in Lake Ulungur: a case of fish invasions in fragile oasis. Russ J Biol Invasions. 3(1):76–80.
- Wan XS, Sheng H-X, Dai M, Church MJ, Zou W-B, Li X-L, Hutchins DA, Ward BB, Kao S-J. 2021. Phytoplankton-nitrifier interactions control the geographic distribution of nitrite in the upper ocean. Global Biogeochem Cycles. 35(11)
- Wang X-L,Luan F-J,Bai X-H,Bai Z-L. 2019a. Correlation between overlying water quality factors and physical and chemical characteristics of sediment in the Ulungur Lake. Environmental Protection of Xinjiang. 41(3):15–21.
- Wang J-L, Fu Z-S, Qiao H-X, Liu F-X. 2019b. Assessment of eutrophication and water quality in the estuarine area of Lake Wuli, Lake Taihu, China. Total Environ. 650:1392–1402.
- Yang L-L, Zhou X-Y, Liu Q-G, Hu Z-J, Wang Y-B, Jang M, Shen J-Z, Liu J, Li Z-Y, Hao Z-C. 2011. Seasonal succession of crustacean zooplankton in relation to the major environmental factors in Lake Ulungur, Xinjiang. Acta Ecol Sin. 31(15):4468–4476.
- Ye S-M, Su D-X, Liu L, Liu X-H, Asiya Han X-L, Liu J, Li Z-Y. 2004. Investigation of aquatic biological resources in Ulungur Lake, Xinjiang. Reserv Fish. 24(2):51–53.
- Zhang S-Y, Zhou Q-H, Xu D, Lin J-D, Cheng S-P, Wu Z-B. 2010. Effects of sediment dredging on water quality and zooplankton community structure in a shallow of eutrophic lake. J Environ Sci. 22(2):218–224.
- Zhao B-X. 1992. Artificial incubation technologies of Hypomesus olidus. Fish Hebei. (3):15–17.
- Zhou X-Y, Hu Z-J, Liu Q-G, Yang L-L, Wang Y-B. 2013. Feeding ecology of the non-indigenous fish Hypomesus nipponensis in Lake Ulungur, China: insight into the relationship between its introduction and the collapse of the native Eurasian perch population. Mar Freshw Res. 64(6):549–557.
- Zhu G-W, Qin B-Q, Zhang L. 2005. Phosphorus species and algae availability in lake sediments in the middle and lower reaches of the Yangtze River. Sci China D Earth Sci. 35(suppl 2):24–32.
- Zou L,Gao F,Ma Y-J. 2021. Spatial distribution of water quality in ulungur lake. Journal of Hydroecology. 42(1):35–41.
- Zuo T, Wang K, Li C-L. 2003. Length-dry weight relationship of Calanus sinicus in the southern part of the Yellow Sea. J Fish China. 27(suppl):103–107.