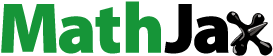
Abstract
Overabundant species can alter a fish community and negatively influence recreationally important species. Introducing new or more predators into a system to control such nuisance fish has been met with limited success and may involve some risk. Understanding to what degree consumption of nuisance species occurs by existing predators and whether an existing predator community is physically capable of consuming the nuisance species can help to determine whether biological control may be possible without new introductions of predators. The objectives of this study were to: (1) document consumption of black bullheads Ameiurus melas by three predators; and (2) determine the relative vulnerability and potential ingestion of bullheads by these predators. We used gastric lavage monthly from May through September to collect stomach contents of channel catfish Ictalurus punctatus, largemouth bass Micropterus salmoides, and walleye Sander vitreus in four reservoirs to calculate the percent occurrence of black bullheads in predator stomachs. We also collected various sizes of juvenile black bullheads to measure body width and depth, with and without the pectoral and dorsal spines extended, respectively. This information was coupled with measured gape widths from the predators to calculate relative vulnerability curves and probabilities of ingestion based on predator size. Black bullheads were rarely consumed by the three predators, and stomach contents generally contained a single black bullhead when consumption did occur. Relative vulnerability based on body depth was similar for all three predators. Potential ingestion was similar among all three predators but was higher for channel catfish compared to the other two predators when black bullhead depths were measured with the dorsal spines extended. This study provides new information about the potential for biological control of black bullheads by an existing predator community.
Introduction
Creating a “balanced” fish community may be possible when energy and biomass inputs are similar to the outputs (Swingle Citation1950; Wydoski and Wiley Citation1999; Kroger and Tucker Citation2012). However, communities may shift out of balance due to external and internal nutrient loading, variations in fish species composition and abundance, water-level fluctuations, or a number of other factors (Scheffer et al. Citation1993; Citation2001). Achieving balance in artificial ecosystems such impoundments may be even more challenging as ongoing changes (e.g., water-level fluctuations, various quantities and quality of water inputs, and rapid aging processes of reservoirs) alter energy and nutrient flows through these systems (Kimmel and Greoger Citation1986; Summerfelt Citation1999). Overabundant and undesirable fish species may affect nutrient distribution and energy cycling within a reservoir and may directly or indirectly affect other fish species within the community (Wydoski and Wiley Citation1999). Some fishes common to impoundments such as common carp Cyprinus carpio and gizzard shad Dorosoma cepedianum have the potential to influence nutrient cycling within an impoundment (Kahl Citation1991; Mundahl Citation1991; Schaus et al. Citation2002; Miller and Crowl Citation2006; Jackson et al. Citation2010). The additional impacts that overabundant species may have on the nutrient loading in a system may lead to further imbalance (Jenkins Citation1958; Wydoski and Wiley Citation1999), but removals of those species may help to mitigate these impacts (Wydoski and Wiley Citation1999).
One common undesirable species in the United States and in some parts of Europe is the black bullhead Ameiurus melas. Nearly 22% of undesirable fish control projects reviewed by Wydoski and Wiley (Citation1999) involved either catostomids, black bullheads, or other Ameiurus species. Black bullheads are native to many waters where they reach nuisance abundances (Jenkins Citation1958; Brown et al. Citation1999) but have been introduced into many waters worldwide (Wheeler Citation1978). Ecological consequences of overabundant black bullheads have been documented. Braig and Johnson (Citation2003) found that an increase of juvenile and adult black bullhead was related to notable increases in turbidity in mesocosms. Nesting activities exhibited by black bullheads may have short-term effects (e.g., 1–2 months) on aquatic food webs due to disruption of aquatic vegetation (Kahl Citation1991; Braig and Johnson Citation2003), sediment mixing, and photic zone reduction (Kahl Citation1991). Leunda et al. (Citation2008) found that introduced black bullheads negatively affected native fish populations directly through consumption or indirectly through reduction of plant material that provided feeding and nursery habitats and invertebrates that were commonly consumed by those fishes. Thus, black bullheads may restructure fish communities and reduce recruitment, survival, and abundance of fish (Sikora et al. Citation2021). Concerns regarding black bullhead overabundance in both their native and introduced ranges has led to interest in identifying effective means of control (Wydoski and Wiley Citation1999; Hanchin et al. Citation2002; Cucherousset et al. Citation2006).
Introductions of predator fishes could potentially control black bullheads, but this approach has not been well studied to date. Blankman (Citation2004) found that introduced flathead catfish Pylodictis olivaris were able to consume and reduce black bullheads to undetectable levels within 14 months after initial introduction in several small farm ponds in Iowa, USA. However, though no flathead catfish were recovered after initial introduction these flatheads could potentially continuously control black bullhead abundance via consumption if the flatheads were to survive (Blankman Citation2004). Other previous studies of black bullhead consumption by native predators provide at least some evidence for the utility of biological control. Liao et al. (Citation2002) found that largemouth bass Micropterus salmoides consumed black bullheads throughout the growing season (i.e., May – October) and that bullheads occurred in ∼ 50% of all bass stomachs sampled in Spirit Lake, Iowa, USA. Northern pike Esox lucius in one shallow bog lake consumed some black bullheads, but bullheads only constituted a small proportion of food items (2%; Pierce et al. Citation2003). The use of existing predators in a community to control black bullheads may be advantageous as costs are relatively low and concerns of introducing new species into a community are avoided (Meronek et al. Citation1996; United States Army Corps of Engineers Citation2014).
However, biological control of black bullheads by any predator may not be feasible when bullhead body size exceeds predator mouth gape. Black bullheads, like other ictalurids, do have the ability to appear larger when threatened by flaring their serrated dorsal and pectoral spines and may cause injury or death of predators during ingestion (Forbes Citation1989; Holm et al. Citation2009; Fine et al. Citation2011). Predator mouths should be wider than these flared sizes in order for consumption to occur, and black bullhead consumption has been linked to predator body size in previous research. Blankman (Citation2004) found that black bullheads were only consumed by flathead catfish greater than 1.4 kg. Isaak et al. (Citation1993) found that only walleye Sander vitreus that had reached at least 380 mm total length were able to consume black bullheads. To determine whether biological control of black bullheads by various predator species is possible, information is needed on the frequency of bullhead consumption by piscivores and whether the sizes of bullheads are potentially vulnerable to predator consumption based on predator gape widths and bullhead body dimensions. The objectives of this study were to: (1) document consumption of black bullheads by three extant predators common to four impoundments; and (2) determine the relative vulnerability and potential ingestion of black bullheads by these predators.
Materials and methods
Black bullheads and their potential predators were sampled from four small (<200 ha, as defined by Pope et al. Citation2009) impoundments across South Dakota, USA (). These four impoundments were selected to encompass physical geographic variability within the state from the east (Lake Alvin and Marindahl Lake) to the west (Bear Butte and Curlew Lakes). All four lakes differ in area and mean and maximum depths ().
Figure 1. Locations of the four small reservoirs in South Dakota, USA used in this study. Bear Butte and Curlew Lakes were sampled in 2017. Lakes Alvin and Marindahl were sampled in 2018.
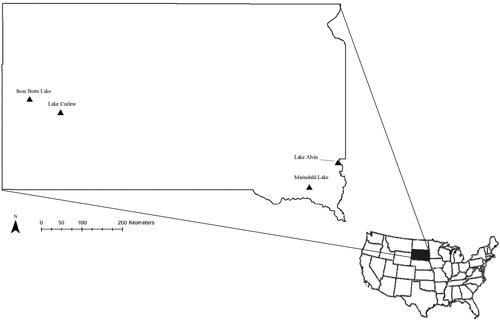
Table 1. Surface area and mean and maximum depths of the four small impoundments sampled for this study. Specific locations of all four waterbodies are shown in .
Western impoundments were sampled in 2017 and eastern impoundments were sampled in 2018. Three predators commonly collected across all four impoundments included channel catfish Ictalurus punctatus, largemouth bass, and walleye. All three species have been stocked at various points in time within the past 15 years in each impoundment in order to maintain populations (stocking reports available at https://apps.sd.gov/GF56FisheriesReports/). Two age groups (i.e., juveniles and adults) of predators were identified based on total length (TL; mm) to account for any potential ontogenetic shifts in food habits (). Predators were collected during the last two weeks of each month from May through September primarily using daytime and nighttime direct current (12–16 A; 170 V), high-pulse frequency (120 pulses *s−1) electrofishing. Supplemental sampling was completed with short-term (10–120 min) gill-net sets with either a large-mesh net (45.7 × 1.8 m; bar mesh = 76.2 mm) or an experimental net (45.7 × 1.8 m; 7.6-m panels; bar mesh = 12.7, 19.1, 25.4, 31.8, 38.1, and 50.8 mm) and short-term (60–120 min) and overnight sets of modified-fyke nets (frame = 0.9 × 1.5 m; bar mesh = 19 mm). All predators were measured for TL and gape width (mm). Gapes were measured via caliper by a single individual to reduce measurement error. Mouths were opened with the individual’s fingers without expanding the flesh to avoid overextension.
Table 2. Length ranges used to classify juvenile and adult channel catfish, largemouth bass, and walleye captured in this study. Categories are based on previous studies documenting the total lengths of predators when they reach sexual maturity.
Stomach contents of predators were collected using gastric lavage (if fish were collected alive) or stomach excision (if mortality occurred during sampling) and preserved in an 80% ethanol solution for later identification and enumeration in the laboratory. We attempted to collect at least 20 predators within each age category with food in their stomachs within each impoundment and month. Stomach contents were identified to species for fish and order for invertebrates when possible. Frequency of occurrence (Oi; Bowen Citation1996) of black bullheads was calculated for each month by species and age group based on the number of examined stomachs that contained at least one bullhead.
Age-0 black bullheads were sampled across all four reservoirs between May and September during the same time periods as the predators. Five, 100-m transects were randomly selected around each reservoir, and fish were collected using a beach seine (mesh = 6 mm; length = 15 m). All black bullheads were counted and measured for body depth and width (mm) with and without the dorsal and pectoral spines extended, respectively. Comparisons of width and depth measurements with and without the spines extended were compared using a Student’s t-test; significance was determined at α = 0.05.
The potential vulnerability of black bullheads to predation was assessed using relative vulnerability and probability of ingestion curves (Hambright et al. Citation1991). Relative vulnerability (V) was calculated using data from all four reservoirs combined as:
where d is the body depth or width of the black bullheads and W is the frequency of the gape widths of the predator under consideration. Separate vulnerability curves were calculated based on black bullhead body depth and width measured with and without dorsal and pectoral spines extended for each predator and age group. Relative vulnerability values range from 0 (i.e., no black bullhead were vulnerable to predation at a given gape width) to 1 (i.e., all black bullhead were vulnerable to predation).
Probability of ingestion [P(I)] curves were developed for each predator species using data combined from all impoundments using the following equation (Hambright et al. Citation1991):
where d is the body depth or width of black bullheads [with either the spine(s) extended or retracted], Vd is relative vulnerability (as calculated above), r is a proportional constant to account for increased potential consumption with increasing piscivore body mass, and D is the frequency of body depths or widths of black bullheads. Similar to relative vulnerability, probability of ingestion ranges from 0 (i.e., no possibility of black bullhead ingestion by predators) to 1 (i.e., highest probability of ingestion). Probability of ingestion was compared visually between predators and for each black bullhead body measurement.
Results
Gape widths were measured on a total of 1,412 predators (). Channel catfish had the widest range and the highest mean gape of all three predators (mean ± standard error = 49 ± 2.2 mm; ), and largemouth bass gapes were the smallest on average (mean = 45 ± 0.8 mm). Walleye gapes were intermediate in size compared to the other two predators (mean = 46 ± 0.6 mm).
Figure 2. Frequency of occurrences of gape widths for three different predators collected from four South Dakota small impoundments. Predator species included channel catfish (top panel; n = 170), largemouth bass (middle panel; n = 590), and walleye (bottom panel; n = 652).
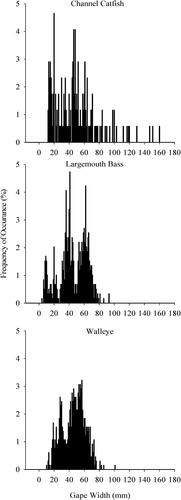
A total of 497 black bullheads were sampled across all impoundments. Average body depth was significantly larger (52 ± 0.3 mm) when the dorsal spine was extended (range = 15–81 mm; mean = 52) than when the spine was not extended (37 ± 0.2 mm; t = −85.0; p ≤ 0.001; ). Body width was significantly larger when pectoral spines were extended (69 ± 0.4 mm) compared to the width measured with pectoral spines retracted (38 ± 0.2 mm; t = −124.5; p ≤ 0.001; ).
Figure 3. Comparison of body depths of black bullheads without (A) and with the dorsal spine extended (B) and the relative vulnerability to potential ingestion (C and D) by three predators common to four South Dakota small impoundments sampled in this study. Measurements of black bullhead depths and predator gape widths were based on fish collected across all four reservoirs combined.
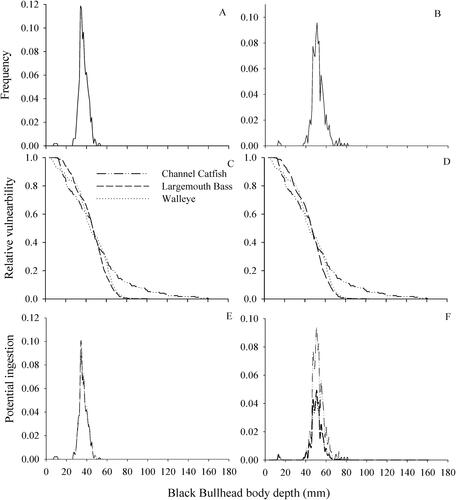
Figure 4. Comparison of body widths of black bullheads without (A) and with pectoral spines extended (B) and the relative vulnerability to potential ingestion (C and D) by three predators common to four South Dakota small impoundments sampled in this study. Measurements of black bullhead depths and predator gape widths were based on fish collected across all four reservoirs combined.
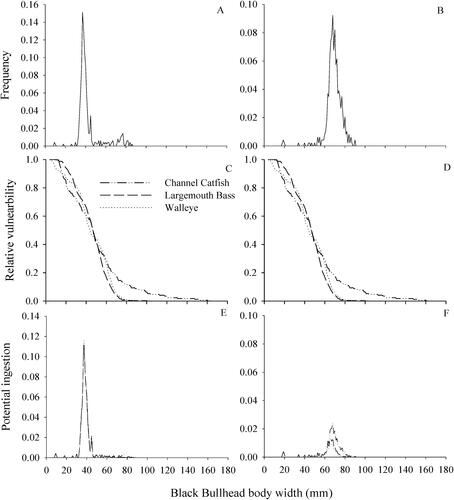
Black bullheads were rarely consumed by the three predators, and stomach contents generally contained a single black bullhead when consumption did occur (). In general, if consumption did occur by any predator, it was only by adults and patterns differed by predator and lake. Black bullheads were only consumed by adult channel catfish in Bear Butte Lake in June and July. No adult channel catfish consumed any black bullheads in any month in the other three lakes. Adult largemouth bass consumed black bullheads in all impoundments sampled, but patterns of consumption differed across each lake. In Bear Butte Lake, largemouth bass only consumed black bullheads in May, but bass consumed bullheads later in the summer (July and/or August) in the other three lakes. Adult walleye only consumed black bullhead in Lake Curlew and consumption was only observed during May.
Table 3. Frequency of occurrence (Oi; %) of Black Bullhead prey items in both adult and juvenile predators across four South Dakota impoundments by month. Numbers in parentheses indicate the number of fish with food in their stomachs that were examined in that lake during that time period. Blanks indicate that no predators of that species and age category were collected from that lake in that month. Zeros indicate that predators were collected during that month but none consumed any black bullhead.
Black bullhead relative vulnerability based on bullhead body depth was similar for all three predators, regardless of whether bullhead depths were measured with the dorsal spine extended or not (). The smallest black bullheads (body depths = 5–12 mm) were highly vulnerable (Vd = 0.8–1.0), and the largest bullheads (body depths = 66–81 mm) collected were low to moderately vulnerable (Vd = 0.01–0.40). Black bullheads were vulnerable to channel catfish at larger sizes compared to largemouth bass and walleye based on bullhead body depths. However, potential ingestion was relatively low for all three predators regardless of whether body depths were measured without dorsal spines extended [P(I) = 0.00–0.12] or with extension [P(I) = 0.00–0.09]. Potential ingestion was similar among all three predators based on black bullhead body depths measured without dorsal spine extension but was higher for channel catfish compared to the other two predators when bullhead depths were measured with the dorsal spines extended.
Black bullhead relative vulnerability based on body width was also similar among the three predators, regardless of whether bullheads widths were measured with pectoral spines extended or not (). Patterns of vulnerability were similar among all three predators; the smallest black bullheads (body widths = 6–23 mm) were highly vulnerable (Vd = 0.8–1.0) and the largest bullheads collected (body widths = 62–83 mm) were minimally vulnerable to predation (Vd = 0.01–0.40). All sizes of black bullheads, especially larger bullheads, were more vulnerable to predation by channel catfish than largemouth bass and walleye. Potential ingestion was similar among predators when pectoral spines were not extended [P(I) = 0.00–0.11] and when pectoral spines were extended [P(I) = 0.00–0.02].
Discussion
This study provides new information about the potential for biological control of black bullheads by an existing predator community. Overall, most of the black bullheads we collected were vulnerable to a degree to all three predators common to each of the four impoundments. However, the occurrence of black bullheads in juvenile and adult predator stomachs was low to negligible and may reflect the low potential ingestion values we calculated based on predator gape widths and various measures of bullhead body widths and depths.
Varied rates of consumption of black bullheads by the same predators and age classes we collected have been found in other studies. A few studies have examined largemouth bass consumption of black bullheads at various ages, both in the wild and captivity. Pelham et al. (Citation2001) found that juvenile largemouth bass did not consume any black bullheads in Spirit Lake, Iowa, USA, but Liao et al. (Citation2002) found that half of adult bass that were sampled in the same lake consumed bullheads. Lewis et al. (Citation1961) found that captive adult largemouth bass consumed few black bullheads relative to other prey items and most bullheads were consumed by the largest bass. Similar to largemouth bass, consumption of black bullheads by walleye may be variable across waters and limited to just the largest individuals. Walter (Citation2000) found that black bullheads were a small portion of the food habits (∼4% by weight) of quality-length and larger walleye (≥ 381 mm TL) in Byre Lake, South Dakota, USA, during the spring. However, black bullheads were not consumed at all by walleye of any size in several other waters in the same U.S. state (Walter Citation2000). Liao et al. (Citation2002) found that black bullheads occurred in <6% of adult walleye (≥305 mm TL) in Spirit Lake, Iowa, USA, but no juvenile walleye consumed any bullhead in that lake (Pelham et al. Citation2001). To date, no study to our knowledge has documented consumption of black bullheads by channel catfish.
Two reasons may explain why variable consumption rates of black bullheads have been observed among different predators. First, black bullheads have an ability to defend themselves unlike other prey (e.g., small-bodied cyprinids; Forbes Citation1989; Holm et al. Citation2009; Fine et al. Citation2011). Second, handling and digestion times of black bullheads may be longer for predators relative to other prey items. Wahl and Stein (Citation1988) found handling times by three Esox species significantly increased when prey had spines versus spineless prey. Several studies have documented that walleye appear to select soft-rayed over spiny-rayed prey species (Parsons Citation1971; Knight et al. Citation1984; Einfalt and Wahl Citation1997). Increased handling and digestion times may negate at least some of the energetic benefits that black bullheads may provide to predators (Werner and Hall Citation1974; Stephens and Krebs Citation1986). Thus, if alternative prey items are available, predators may select alternative energy sources rather than incur injury or lose energy to consume black bullheads.
Results from this study provide new information on biological control of overabundant black bullheads. However, some limitations in this study exist. One such limitation is that we did not evaluate the abundance of alternative prey sources in these reservoirs. Such information could have been used to calculate selectivity of various prey items, including black bullheads, by predators to determine whether the availability of alternative prey plays a role in consumption of bullheads. A second limitation was that sampled black bullheads were all of a similar size, thus, smaller or larger bullheads were not sampled. Incorporation of a wider size range of black bullheads could provide a more comprehensive evaluation of relative vulnerability and potential ingestion. Third, we only assessed consumption of black bullheads by individual predators. A fuller understanding of the impacts predators may have on controlling black bullheads may include additive effects from all predators coupled with an assessment of the existing stock on black bullheads in order to estimate the total effects of consumption on bullhead populations. Fourth, we did not have any measurements of water quality (e.g., productivity) or habitat complexity that may have influenced the ability of black bullhead to avoid predation or limited the ability of predators to find any prey, including bullheads. Such factors have been noted to potentially influence predation on other non-native, nuisance species such as common carp (Weber and Brown Citation2012) and gizzard shad (Michaletz Citation1999). Collectively, this additional research coupled with the results of our study can provide information on the possible use of biological control of black bullhead by predator fish and under what conditions predators consume bullheads.
Acknowledgements
Funding for this research was provided by Federal Aid in Sport Fish Restoration administered by the South Dakota Department of Game, Fish and Parks (Study #1537). We thank the personnel with the Rapid City and Sioux Falls offices of the South Dakota Department of Game, Fish and Parks who assisted with the planning of this project and the collection of data. Additional appreciation is extended to Jake Comer, Austin Galinat, Will Gallman, Jared Hintz, Seth Fopma, Rebecca Kolstrom, Joe Mrnack, Madison Posutsa, Travis Rehm, Katie Schlafke, Allison Shorter, Aaron Sundmark, and Kate Wollman for their assistance with field sampling and laboratory processing. Finally, we thank two anonymous reviewers for their comments and suggestions for improving this manuscript.
Disclosure statement
The authors of this study assert that we have no conflicts of interest, financial or otherwise, related to this specific research.
Data availability statement
The data that support the findings of this study are openly available in Mendeley Data at https://data.mendeley.com/datasets/g53r4hf79x/1 (doi: 10.17632/g53r4hf79x.1).
Additional information
Funding
References
- Blankman J. 2004. Using flathead catfish to renovate ponds overrun with bullheads: Improving water quality within and exiting the ponds. Manning, IA: United States Department of Agriculture, Sustainable Agriculture Research and Education Project FNC 03-453, Final Report.
- Bowen SH. 1996. Quantitative description of the diet. In: B. R. Murphy & D. W. Willis, editors, Fisheries techniques. 2nd ed. Bethesda, MD: American Fisheries Society; p. 513–532.
- Braig EC, Johnson DL. 2003. Impact of black bullhead (Ameiurus melas) on turbidity in a diked wetland. Hydrobiologia. 490(1/3):11–21.
- Brown ML, Willis DW, Blackwell BG. 1999. Physiochemical and biological influences on black bullhead populations in eastern South Dakota glacial lakes. J Freshwater Ecol. 14(1):47–60.
- Cucherousset J, Paillisson J, Carpentier A. 2006. Is mass removal an efficient measure to regulate the North American catfish Ameriurus melas outside of its native range? J Freshwater Ecol. 21(4):699–704.
- Einfalt LM, Wahl DH. 1997. Prey selection by juvenile walleye as influenced by prey morphology and behavior. Can J Fish Aquat Sci. 54(11):2618–2626.
- Fine ML, Sismour EN, Newton SH, Bosher BT, Sullivan ADH, Miano JP, Ghahramani ZN, Mohajer YJ, Nellis S. 2011. A primer of functional morphology and behavioral ecology of the pectoral spine of the channel catfish. In: P. H. Michaletz & V. H. Travnichek, editors, Conservation, ecology, and management of catfish: the second international symposium. Bethesda, MD: American Fisheries Society; p. 745–753.
- Forbes SL. 1989. Prey defenses and predator handling behavior: the dangerous prey hypothesis. Oikos. 55(2):155–158.
- Hambright KD, Drenner RW, McComas SR, Hairston NG. Jr., 1991. Gape-limited piscivores, planktivore size refuges, and the trophic cascade hypothesis. archiv_hydrobiologie. 121(4):389–404.
- Hanchin PA, Willis DW, St. Sauver TW. 2002. Comparison of concurrent trap-net and gill-net samples for black bullheads. J Freshwater Ecol. 17(2):233–237.
- Holland RS, Peters EJ. 1992. Age and growth of channel catfish (Ictalurus punctatus) in the lower Platte River, Nebraska. Transactions of the Nebraska Academy of Sciences. 19:33–42.
- Holm E, Mandrak NE, Burridge ME. 2009. The ROM field guide to freshwater fishes of Ontario. Toronto, ON: Royal Ontario Museum.
- Isaak LC, Neumann RM, Willis DW. 1993. Food habits of walleyes in Lake Thompson. South Dakota. Prairie Naturalist. 25:325–330.
- Jackson JZ, Quist MC, Downing JA, Larscheid JG. 2010. Common carp (Cyprinus carpio), sport fishes, and water quality: ecological thresholds in agriculturally eutrophic lakes. Lake Reservoir Manage. 26(1):14–22.
- Jenkins RM. 1958. The standing crop of fish in Oklahoma ponds. Proceedings of the Oklahoma Academy of Science, 38, 157–172.
- Kahl R. 1991. Restoration of canvasback migrational staging habitat in Wisconsin. Wisconsin Madison, WI: Wisconsin Department of Natural Resources Technical Bulletin 172.
- Kansas Fishes Committee. 2014. Kansas fishes. Lawrence, KS: University Press of Kansas.
- Kimmel BL, Greoger AW. 1986. Limnological and ecological changes associated with reservoir aging. In: G. E. Hall & M. J. van Den Avyle, editors, Reservoir fisheries management: strategies for the 80’s. Bethesda, MD: American Fisheries Society; p. 103–109.
- Knight RL, Margraf FJ, Carline RF. 1984. Piscivory by walleyes and yellow perch in western Lake Erie. Trans Am Fish Soc. 113(6):677–693.
- Kroger R, Tucker CS. 2012. Biological productivity of small impoundments. In: Small impoundments management in North America. In: J. W. Neal & D. W. Willis, editors, Small impoundment management in North America. Bethesda, MD: American Fisheries Society; p. 83–111.
- Leunda PM, Oscoz J, Elvira V, Agorreta A, Perea S, Miranda R. 2008. Feeding habits of the exotic black bullhead Ameiurus melas (Rafinesque) in the Iberian Peninsula: first evidence of direct predation on native fish species. Journal of Fish Biology. 73(1):96–114.
- Lewis WM, Gunning GE, Lyles E, Bridges WL. 1961. Food choice of largemouth bass as a function of availability and vulnerability of food items. Trans Am Fish Soc. 90(3):277–280.
- Liao H, Pierce CL, Larscheid JG. 2002. Diet dynamics of the adult piscivorous fish community in Spirit Lake, Iowa, USA, 1995-1997. Ecology of Freshwater Fish. 11(3):178–189.
- Meronek TG, Bouchard PM, Buckner ER, Burri TM, Demmerly KK, Hatleli DC, Klumb RA, Schmidt SH, Coble DW. 1996. A review of fish control projects. North Am J Fish Manage. 16(1):63–74.
- Michaletz PH. 1999. Influence of reservoir productivity and juvenile density on first-year growth of gizzard shad. North Am J Fish Manage. 19(3):842–847.
- Miller SA, Crowl TA. 2006. Effects of common carp (Cyprinus carpio) on macrophytes and invertebrate communities in a shallow lake. Freshwater Biol. 51(1):85–94.
- Mundahl ND. 1991. Sediment processing by gizzard shad, Dorosoma cepedianum (Lesueur), in Acton Lake, Ohio, USA. J Fish Biology. 38(4):565–572.
- Parsons JW. 1971. Selective food preferences of walleyes in the 1959 year class in Lake Erie. Trans Am Fish Soc. 100(3):474–485.
- Pierce RB, Tomcko CM, Drake MT. 2003. Population dynamics, trophic interactions, and production of northern pike in a shallow bog lake and their effects on simulated regulation strategies. North American Journal of Fisheries Management,. 23(1):323–330.
- Pelham ME, Pierce CL, Larscheid JG. 2001. Diet dynamics of the juvenile piscivorous fish community in Spirit Lake, Iowa, USA, 1997-1998. Ecology of Freshwater Fish. 10(4):198–211.
- Pope KL, Neuman RM, Bryan SD. 2009. Warmwater fish in small standing waters. In: S. A. Bonar, W. A. Hubert, & D. W. Willis, editors. Standard methods for sampling North American freshwater fishes. Bethesda, MD: American Fisheries Society; p. 13–27.
- Schaus MH, Vanni MJ, Wissing TE. 2002. Biomass-dependent diet shits in omnivorous gizzard shad: implications for growth, food web, and ecosystems effects. Trans Am Fish Soc. 131(1):40–54.
- Scheffer M, Carpenter S, Foley JA, Folke C, Walker B. 2001. Catastrophic shifts in ecosystems. Nature. 413(6856):591–596.
- Scheffer M, Hosper SH, Meijer ML, Moss B, Jeppesen E. 1993. Alternative equilibria in shallow lakes. Trends Ecol Evol. 8(8):275–279.
- Sikora LW, VanDeHey JA, Sass GG, Matzke G, Pruel M. 2021. Fish community changes associated with bullhead removals in four northern Wisconsin lakes. North American Journal of Fisheries Management, Special Issue: catfish 2020—the 3rd International Catfish Symposium.
- Stephens DW, Krebs JR. 1986. Foraging theory. Princeton, NJ: Princeton University Press, Princeton.
- Summerfelt RC. 1999. Lake and reservoir habitat management. In: C. C. Kohler & W. A. Hubert, editors, Inland fisheries management in North America. 2nd ed. Bethesda, MD: American Fisheries Society; p. 285–320.
- Swingle HS. 1950. Relationships and dynamics of balanced and unbalanced fish populations. Auburn, AL: Agricultural Experiment Station of the Alabama Polytechnic Institute. Bulletin 274.
- United States Army Corps of Engineers. 2014. Biological controls. Chicago, IL: United States Corps of Engineers.
- Wahl DH, Stein RA. 1988. Selective predation by three esocids: the role of prey behavior and morphology. Trans Am Fish Soc. 117(2):142–151.
- Walter DD. 2000. Food habits of walleye and saugeye when stocked as secondary predators into small South Dakota impoundments [Master’s thesis]. Brookings, SD: South Dakota State University.
- Weber MJ, Brown ML. 2012. Effects of predator species, vegetation and prey assemblage on prey preferences of predators with emphasis on vulnerability of age-0 common carp. Fisheries Management and Ecology. 19(4):293–300.
- Werner EE, Hall DJ. 1974. Optimal foraging and the size selection of prey by the bluegill sunfish (Lepomis macrochirus). Ecology. 55(5):1042–1052.
- Wheeler A. 1978. Ictalurus melas (Rafinesque, 1820) and I. nebulosus (Lesueur, 1819): the North American catfish in Europe. J Fish Biology. 12(5):435–439.
- Willis DW, Isermann DA, Hubers MJ, Johnson BA, Miller WH, St. Sauver TR, Sorenson JS, Unkenholz EG, Wickstrom GA. 2001. Growth of South Dakota fish: A statewide summary with means by region and water type. Pierre, SD: South Dakota Department of Game, Fish and Parks, Special Report No. 01–05.
- Wydoski RS, Wiley RW. 1999. Management of undesirable fish species. In: C. C. Kohler & W. A. Hubert, editors, Inland fisheries management in North America. 2nd ed. Bethesda, MD: American Fisheries Society; p. 403–430.