Abstract
Lake Shala, endorheic Soda Lake in the Ethiopian Riftvally Lake, supports an enormous biodiversity, with a large human population and economic development. However soda lakes are under several ecological changes, with unexpected shifts in biodiversity composition and physicochemical changes. This study focuses on assessing the community structure of zooplankton in Lake Shala. Samples were taken at monthly intervals from January to December 2018. A total of 32 zooplankton species were identified, of which 23 species were Rotifers and followed by Copepoda (6) and Cladocera (3). The community was dominated by Brachionus dimidiatus, B. dimidiatus inermis, B. plicatilis and B. calyciflorus. Their abundance exhibit significant seasonal and vertical variations (ANOVA, p < 0.05). Zooplankton community structure of Lake Shala has changed over time, benthic harpacticoids (Nitocra lacustris) have become replaced by small-bodied and generalist-feeder rotifers (genus Brachionus). Such ecological shifts may be driven mainly by partial atelomixis in this deep tropical lake. Overall, zooplankton species distribution and abundance are influenced by various environmental factors such as conductivity, salinity, Chl-a and nutrients. This study concludes that the composition and community structure of zooplankton in Lake Shala has changed over time with the change in physicochemical and internal limnological dynamics (atelomix).
1. Introduction
Zooplankton communities play a significant ecological role in aquatic ecosystems (Fernandes and Ramaiah Citation2019), being a critical link between phytoplankton and higher consumers and they contribute to element recycling and energy transfers (Burian Citation2010; Helenius Citation2015). The density and diversity of zooplankton are greatly influenced by different biotic and abiotic factors like physicochemical parameters of water, availability of phytoplankton, lake morphology, and anthropogenic changes in lakes and watersheds (Okoth et al. Citation2011; Waya et al. Citation2014; Diego et al. Citation2015; Merhoon et al. Citation2017), which alter characteristics and ecology of the lake (Fernández-Álamo and Färber-Lorda Citation2006; Helenius Citation2015). Therefore, understanding their dynamics and the factors that affect their community, and their critical linkages with other ecosystem elements, remains an important focus in aquatic ecology to optimizing resource use and enhancing sustainable lake ecosystem management (Joseph and Yamakanamardi Citation2011; Savitha and Yamakanamardi Citation2012).
Soda lakes are variable in their physicochemical features and prone to hydrological influences since they are often in semi-arid regions (Oduor and Schagerl Citation2007; Schagerl et al. Citation2015; Oduor and Kotut Citation2016). These factors control the zooplankton diversity and community structure (Burian Citation2010; Okoth et al. Citation2011). The zooplankton community structure of saline-alkaline lakes is related to the salinity gradient (Gülle et al. Citation2010; Anton-Pardo and Armengol Citation2012; Diego et al. Citation2015). In addition, saline-alkaline lake zooplankton is strongly influenced by hydrodynamics, while seasonality impacts community composition (Isumbisho et al. Citation2006; Okoth et al. Citation2011) or other factors, mainly nutrients, water depth, temperature, dissolved oxygen and food availability can be reasons for vertical and horizontal variations in species composition (Waya et al. Citation2014; Voutilainen et al. Citation2016).
Despite the importance of zooplankton in aquatic ecosystems, understanding of their dynamics and the key factors that affect their community structure have so far been unexplained well in alkaline–saline lakes, especially Ethiopian saline-alkaline lakes have received less attention than other kinds of water systems. The only published sets of data on zooplankton of Lake Shala are those of Green and Mengestou (Citation1991), Defaye (Citation1988) and Mengistou (Citation2016), whose investigations involved the analysis of single occasion sampling. Lake Shala offers a good model to investigate the role of partial atelomxis in driving plankton dynamics in the surface mixing layers of such deep tropical lakes, like Lake Zirahuén, Mexico (Tavera and Martı’nez-Almeida Citation2005), Dom Helvécio and Carioca lakes, Brazil (Souza et al. Citation2008; Barbosa et al. Citation2011, Citation2013). However, few observations have been done on zooplankton dynamics in deep highland lakes in Ethiopia, especially in relation to seasonal mixing patterns (Fetahi et al. Citation2011; Degefu and Schagerl Citation2015).
Lake Shala has been facing ecological degradation due to rapidly-increasing human populations and development activities in the basin during the past ten years (Zinabu et al. Citation2002; Ayenew and Legesse Citation2007). Furthermore, there is a future plan to expand the Abijata Soda Ash production factory to Lake Shala which may impact the structure of biological communities. Therefore, the present study had 3 major objectives a) prepare a checklist of the zooplankton community which could serve as baseline data to monitor future changes in the lake; b) determine the major drivers of zooplankton dynamics in the lake; c) assess long-term trends in zooplankton composition and biomass in relation to internal and external impacts in the lake (atelomixis or catchment impacts). The study may also contribute towards a better understanding of how climate change and human impacts affect zooplankton dynamics in deep tropical lakes.
2. Materials and methods
2.1. Description of the study area
Lake Shala lies between 7°24′-7°33’N and 38°23′-38°39’E at altitudes of approximately 1558 m within the Abijata-Shala Lakes National Park (). The lake is an endorheic and volcano-tectonic lake (Le Turdu et al. Citation1999). Lake Shala is the deepest among the Ethiopian Rift valley lakes (maximum 266 m), with a surface area of around 329 km2 (Von Damm and Edmond Citation1984; Baxter Citation2002). This is an inhospitable place, with caustic water concentrated by the high evaporation rate (Ayenew and Legesse Citation2007). Two rivers (Adabat and Gidu) (Baumann et al. Citation1975; Baxter Citation2002) and a number of hot springs supply water to the lake.
Figure 1. Map of Lake Shala showing the study sites/sampling points. (Abbreviation: SPS: Shala Park Shore; SPO: Shala Park Open; SGS: Shala Gike Shore; SGO: Shala Gike Open).
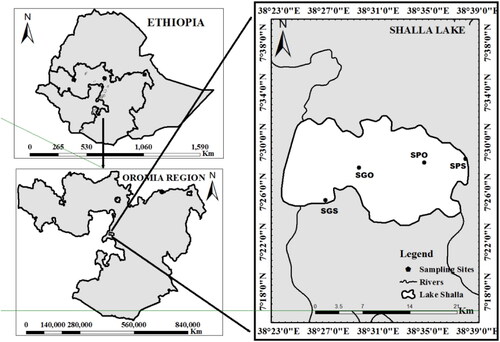
Lake Shala is characterized by a high pH, conductivity, and a high phosphate content, but with very low nitrogen levels (Kebede et al. Citation1994; Ogato Citation2015). Despite this hostile nature of the environment, Lake Shala supports phytoplankton and zooplankton, dominated by diatoms and rotifers, respectively (Kebede et al. Citation1994; Mengistou Citation2016)). The benthic macroinvertebrate community of the lake comprises Tubificidae, Ostracoda and Chironomidae (Tudorancea and Harrison Citation1988). Lake Shala and its islands support a large population of Lesser Flamingos (Phoeniconaias minor Geoffroy), Greater White Pelican, Cormorants, Storks and Phalacrocorax carbo (Stephenson Citation1978; Estifanos Citation2008; Almaw Citation2012). The cichlid Oreochromis niloticus and small-sized fish, Aplocheilichthys sp., being reported for the lake (Golubtsov et al. Citation2002).
2.2. Physicochemical parameters
Physicochemical parameter data were collected together with the zooplankton samples from January to December 2018. The measurements of water temperature, pH, salinity, dissolved oxygen (DO), and electrical conductivity (EC) were conducted in situ with a portable HQ40D Multimeter. For nutrient analysis, composite water samples (by compositing samples from different depths) were taken from each sampling site in triplicate and transported and analyzed spectrophotometrically in the Limnological Laboratory of Addis Ababa University. NO3-N was analyzed with sodium salicylate method, NH3-N with indo-phenol blue (APHA 1995), and soluble reactive phosphate (PO4-P) with ascorbic acid method (APHA, 2000). NO2-N concentration was determined by the reaction between sulfanilamide and N-naphthyl-(1)-ethylenediamine dihydrochloride (APHA, 1995). Total phosphorus (TP) from unfiltered samples and silica (SiO2-Si) were determined using persulfate digestion and Molybdosilicate Method, respectively (APHA, 1999). Total alkalinity (TA) was determined by titration with 1 N HCl to pH 4.5 using a mixed indicator (bromocresol green-methyl red) within a few hours of sample collection according to Wetzel and Likens (Citation2000).
2.3. Zooplankton and other biological data sampling
Zooplankton collected at monthly intervals from January to December 2018 from four sampling stations (). Zooplankton samples were obtained by vertically hauled from 30 m to the surface from two open water stations and 10 m from two shore sites through a 30-μm plankton net. A vertical bottle sampler (van Dorn, 2 L capacity) was also used for the determination of vertical distribution and abundance of zooplankton at the water surface (0 m), 5, 10, 15, 20, and 30 m depth of open water sampling station and water temperature, electric conductivity and dissolved oxygen were measured at each depth simultaneously with zooplankton sampling. The zooplankton samples were preserved in 4% formalin and brought to Addis Ababa University, Limnology Laboratory. Zooplankton was identified as the lowest taxonomic unit according to these taxonomic keys: Copepods (Defaye Citation1988), Cladocerans (Korinek Citation1999) and Rotifers (Fernando Citation2002). Counting was carried out using a plankton counting chamber under an inverted microscope. Total counts were made and individual densities were expressed as numbers per liter. The abundance of phytoplankton was calculated using the equation in Hötzel and Croome (Citation1999) and Phytoplanktonic Chlorophyll-a (Chl-a) concentrations were estimated from water samples collected from the same place where the in situ variables were measured. Chl a was extracted using 90% acetone by following the procedure of Talling and Driver (Citation1963). Fish pressure.on zooplankton aboundance and diversity was determined by fish stomach content analysis (Hyslop Citation1980). Macro-invertebrates were collected based on the method outlined in Ontario Benthos Biomonitoring Network Protocol Manual (Jones et al. Citation2007).
2.4. Data analyses
Spatial and temporal variability of zooplankton community between sites, and among seasons, were analyzed by non-parametric Kruskall–Wallis ANOVA (Kruskal and Wallis Citation1952). The association between the distribution of zooplankton species, abiotic and biotic factors and sampling stations was evaluated with canonical multivariate analysis using CANOCO windows 4.5 version Software (Ter Braak and Smilauer Citation2002). Detrended correspondence analysis (DCA) was employed to check the response of the data, and the length gradient was 0.195. Therefore, redundancy analysis (RDA) was used to determine the relationship between zooplankton species distribution and environmental parameters.
3. Results
3.1. Spatial, seasonal and trends in physicochemical parameters
The seasonal and spatial environmental variables of Lake Shala are presented in and these variables showed evident spatial and seasonal variations (ANOVA: p < 0.05), except DO, which showed only seasonal variation in Lake Shala ( and ). pH (10.08), Alkalinity (291.1 meq/L), NO3-N (0.17 μg/L), NH3-N (108.78 μg/L), SiO2 (1.09 mg/L), TP (2.03 mg/L) and SRP (1.25 mg/L) were significant at Shala Gike Shore (SGS).
Table 1. Spatial variation of physical environmental variables of the studied stations of Lake Shala (SPS: Shala Park Shore; SPO: Shala Park Open; SGS: Shala Gike Shore; SGO: Shala Gike Open; Temp: Temperature; DO: Dissolved Oxygen; EC: Electrical Conductivity; Alka: Alkalinity).
Table 2. Seasonal variation of physical environmental variables of Lake Shala (Temp: Temperature; DO: Dissolved Oxygen; EC: Electrical Conductivity; Alka: Alkalinity).
Mean concentrations of pH (10.1), Alkalinity (380.5 meq/L), NO3-N (0.19 μg/L), NH3-N (113.69 μg/L), SiO2 (1.16 mg/L), TP(2.14 mg/L) and SRP (1.45 mg/L) were recorded during rainy season, whereas DO (8.12 mg/L), EC (33.99 mS/cm) and salinity (21.34 g/L) were recorded during the dry season and were significantly different.
Comparing the present physicochemical variables value to studies made during 1961-2012, a long-term increasing trend in pH, Alkalinity, Salinity, EC, NO3-N, NH3-N, SRP and TP, and a decreasing trend in SiO2 were observed (). The mean electrical conductivity was generally higher in the present study compared to data from the 1960s () and nearly comparable to the mean value (33000 μS/cm) reported by Talling and Talling (Citation1965) (). The current mean value of salinity in Lake Shala (19.6 g L−1) is comparable to the value (19.582 g L−1) reported by Talling and Talling (Citation1965), but higher than that reported by Baumann et al. (Citation1975) (16.8 mg L−1); 18.1 mg L−1 Kebede et al. (Citation1994) and 15.7 mg L−1 (Talling and Talling Citation1965).
Table 3. Some previous and current physicochemical factors of Lake Shala, and trends over 50 years back from the present study.
The concentration of soluble reactive phosphorus (SRP) appears to have decreased from 1964 to 2000 in Lake Shala except in 1991 (809 μg L−1) a record by Kebede et al. (Citation1994), however highest record was reported during the recent year sampling period by Ogato (Citation2015) (961 μg L−1) and 1020 μg L−1 in the present study. Concentrations of silicate decreased markedly in Lake Shala. Compared to the present data, the high silicate value (130 mg L−1) were recorded in the 1960s by Talling and Talling (Citation1965). Ogato (Citation2015) measured the lowest concentration in 2012 comparable to the current result (). The mean concentration of NH3-N increased considerably when compared with some results reported by Kebede et al. (Citation1994) (4.3 μg L−1), Zinabu (Citation2002) (4.65 μg L−1), Ogato (Citation2015) (42 μg L−1).
3.2. Zooplankton composition and abundance
A total of 32 zooplankton taxa were recorded in Lake Shala across all sites and in the studied periods (). Of these species composition of zooplankton of Lake Shala, the rotifers had the highest species richness of the total zooplankton (23 taxa), of the 23 rotifers identified, 10 species belonged to the genus Brachionus including B. budapestinesis, B. calyciflorus, B. dimidiatus, B. dimidiatus inermis, B. falcatus, B. plicatilis, B. quadridentatus, B. rubens, and B. urceolaris. Other groups were represented by Copepoda (6 taxa) and Cladocera (3 taxa) ().
Table 4. Zooplankton taxa recorded in Lake Shala from January- December 2018.1.
The mean abundance of zooplankton ranged from 830.4 to 1538.6 ind.L−1, with the maximum values in August and the minimum in January (). Among all the zooplankton samples, rotifers accounted for the most considerable fraction of zooplankton density and contributed 86.11% of the total zooplankton density (). Among the rotifers, Brachionus dimidiatus, B. dimidiatus inermis, B. plicatilis and B. calyciflorus dominated during all sampling periods. The highest cell density of B. dimidiatus occurred during the dry (January - March) and post rainy months (September -December). The cell density of B. dimidiatus inermis, however, increased during the pre-rainy months (April - May). Similar patterns of B. plicatilis and B. calyciflorus were observed, with increasing abundance during the rainy months (July - September) ().
Figure 2. Monthly pattern of total zooplankton abundance (ind. L−1) in Lake Shala from January to December 2018.
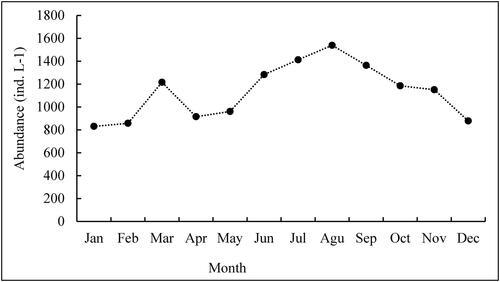
3.3. Spatial and seasonal distribution of zooplankton
The spatial and seasonal variations in the abundance of zooplankton were computed for all the sampling sites and seasons ( and ). On average SPS site had the highest zooplankton mean abundance of 1198.5 ± 296.3 ind.L−1 followed by SPO (1145.0 ± 214.8 ind.L−1), SGO (1109.6 ± 257.4 ind.L−1) and SGS (1076.6 ± 360.2 ind.L−1) (). However, there was no significant difference in spatial zooplankton abundance among the four stations (F = 0.395, p = 0.757).
Table 5. Spatial and total abundance variations in the zooplankton community.
Table 6. Seasonal variations in the abundance (ind.L−1) of the zooplankton group.
The seasonal maximal abundance of zooplankton was observed during the rainy season as 1438.1 ± 159.5 ind.L−1, and minimum abundance during the dry seasons as 967.8 ± 209.0 ind.L−1, thus seasonal fluctuation in the zooplankton community was important (p < 0.05) (). Rotifer, Nauplii, Copepoda and Cladocera abundance (ind.L−1) exhibited significant seasonal pattern, showing a maximum during rainy seasons for Rotifers, Copepoda and Cladocera while Nauplii during post rainy seasons ().
3.4. Vertical distribution of zooplankton
The vertical distribution and percentage contribution of the zooplankton group in Lake Shala are shown in . The vertical distribution of zooplankton demonstrated a significant correlation between zooplankton groups and water depth. The mean zooplankton abundance was greatest in the top 10 m of the water column, having its maximum in the surface (1,357. 7 ind.L−1). However, a size-structured habitat utilization by zooplankton populations in the lake varied in depth. The average density of rotifer and nauplii decreased with increasing depth, it attained the highest averages of 712.7 ind.L−1 and 504.1 ind.L−1 at the surface and it decreased to 1.6 ind.L−1 and 39.6 ind. L−1 at 15–20 m, respectively (). The greatest contrast in the distribution and percentage contribution was observed for the copepod and cladoceran, which were concentrated in the mid and deeper layer of the water, respectively (). Vertical electrical conductivity, temperature, and dissolved oxygen profile of the lake were determined in the same period (). The vertical distribution patterns of zooplankton were remarkably similar to that of electrical conductivity, temperature, and dissolved oxygen curves ( and ).
3.5. Zooplankton species and environmental variables
The Redundancy Analysis (RDA) summarized 94.5% of the total variance in species data on the first two axes (axis 1, 73.5%; axis 2, 20.9%). Some zooplankton species were associated with environmental variables: (i) Brachionus angularis, B. urceolaris, B. plicatilis, Thermocyclops ethiopiensis, and Diaphanosoma excisum, highlighted on the right-hand side were associated with high levels of SRP, NH3-N, NO3-N, TP and macroinvertebrate, and negatively correlated with salinity and electrical conductivity; (ii) Brachionus dimidiatus, B. rubens, B. quadridentatus and T. oblongatus were positively correlated with Alkalinity, Chl a, phytoplankton abundance, pH and fish pressure; (iii) Moina micrura, M. belli, Afrocyclops gibsoni, Eucyclops serrulatus and nauplii shown on the left-hand side were associated with salinity, electrical conductivity and dissolved oxygen; (iv) Brachionus dimidiatus inermis and Brachionus calyciflorus were positively correlated with temperature. In addition, most species shown in the upper half of the tri-plot were negatively correlated with salinity and EC ().
Figure 7. Redundancy analysis (RDA) triplot of zooplankton species in relation to water physicochemical properties and sites (E. serru. - Eucyclops serrulatus, A. gibso. -Afrocyclops gibsoni, M. micru. - Moina micrura, M. belli. - Moina belli, B. calyc. - Brachionus calyciflorus, B.d. in. - Brachionus dimidiatus inermis, D. excis.-Diaphanosoma excisum, B. angul.- Brachionus angularis, T. ethio. - Thermocyclops ethiopiensis, B. plica. - Brachionus plicatilis, B. urceol. - Brachionus urceolaris, T. oblong. - Thermocyclops oblongatus, B. quadr.- Brachionus quadridentatus, B. dimid. - Brachionus dimidiatus, B. ruben. - Brachionus rubens, SPS-Shala Park Shore; SPO-Shala Park Open; SGS-Shala Gike Shore; SGO-Shala Gike Open; Alka-Alkalinity; Chl a - Chlorophyll; Phyto Ab - Phytoplankton Abundance).
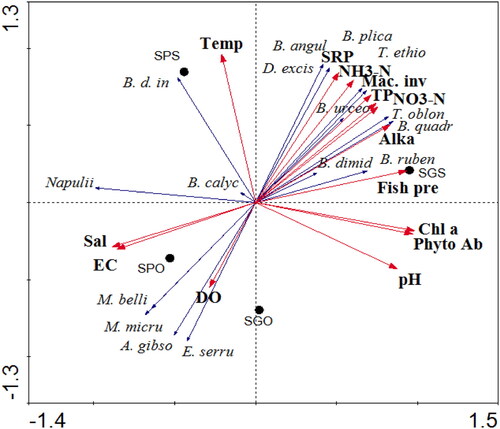
4. Discussion
4.1. Composition and distribution of zooplankton
Zooplankton composition of Lake Shala exhibited typically tropical saline inland water euryhaline biota forms (Derry et al. Citation2003; Burian Citation2010; Okoth et al. Citation2011; Ong’ondo et al. Citation2013), wherein few tolerant and adapted species attained high population densities, as previously reported for this lake (Green and Mengestou Citation1991). In the present study, euryhaline rotifers and crustaceans such as Brachionus dimidiates, B. plicatilis, B. calyciflorus, Afrocyclops gibsoni, Eucyclops serrulatus, Thermocyclops oblongata and T. ethiopiensis inhabited the lake. These rotifers and crustacean species are cosmopolitan and broadly distributed given their broad tolerance to salinity (Derry et al. Citation2003; Echaniz et al. Citation2006; Okoth et al. Citation2011).
In the present study, small-sized rotifers (B. dimidiatus, B. dimidiatus inermis, B. plicatilis and B. calyciflorus) were numerically dominant in the zooplankton population with a significant difference. Similarly, several studies reported the dominance of rotifers in saline-alkaline lakes such as Lake Nakuru, Bogoria, and Abijata (Wodajo and Belay Citation1984; Green and Mengestou Citation1991; Burian Citation2010; Okoth et al. Citation2011). The leading factors to explain this dominance by rotifers are attributed to their fast reproductive rates and developmental stages (Yin et al. Citation2016), unspecialized feeding (Joseph and Yamakanamardi Citation2011) and low abundance and diversity of cladocerans (Anton-Pardo and Armengol Citation2012) in saline lakes promote the development of rotifers. Also rotifers filter small-sized diatoms more efficiently that large-sized Cladocera and Copepoda in the mixing layer in Lake Shala.
The species richness of cladocerans was low and they occurred rarely, according to Afonina and Tashlykova (Citation2018) this group is typical of freshwater, and most species do not tolerate high salinity and electrical conductivity. In East Africa Soda Lakes, species richness and composition are correlated positively with salinity (Mengestou, Mengistou Citation2016). The species richness of Cladocerans decreases with increasing levels of salinity and electrical conductivity of the water body (Diego et al. Citation2015). High salinity and conductivity in Lake Shala also restricted the diversity of Cladocerans species. This agrees with the observations of Ong’ondo et al. (Citation2013) and Wodajo and Belay (Citation1984), who reported low or no record of Cladocera diversities in soda lakes due to high conductivity and salinity, which limits osmoregulation capacity, reduce reproductive and survival rates of Cladocerans (Tavsanoglu et al. Citation2015; Afonina and Tashlykova Citation2018).
Even though significant differences in environmental factors such as physicochemical parameters were recorded in four sampling stations, the rotifer, nauplii, copepoda and cladoceran abundance did not exhibit significant differences among sampling stations. The relative zooplankton abundance homogeneity among sites of Lake Shala follows the findings of Nandini et al. (Citation2008) in the Valle de Bravo reservoir. However, Dagne et al. (Citation2008) and Yongo and Outa (Citation2017) found the strongest horizontal gradients in large-bodied zooplankton. In the present study, the observed lack of horizontal or spatial distribution is not a surprise since the lake is dominated by small-bodied taxa and juvenile ontogenetic stages which have low susceptibility to predation and coupled with the low locomotory ability adequately explains a lack of spatial variations in distribution. Although the physical structure of Lake Shala is distinguished by high wind mixing in the afternoon (partial atelomixis), which may have a significant contribution to the distinct lack of horizontal variations in zooplankton distribution. Souza et al. (Citation2008) and Degefu and Schagerl (Citation2015) also mentioned the key role of atelomixis as a critical factor in the tropical lakes for the plankton community structure.
Several studies on other saline-alkaline lakes documented seasonal variations in zooplankton abundance (Wodajo and Belay Citation1984; Derry et al. Citation2003; Gülle et al. Citation2010; Okoth et al. Citation2011; Afonina and Tashlykova Citation2018). These studies were on deep, oligotrophic lakes such as Lake Burdur (Gülle et al. Citation2010), and shallow alkaline–saline lakes of Ethiopia (Lake Abijata) and Kenya (Lake Nakuru and Bogoria) (Wodajo and Belay Citation1984; Burian Citation2010; Okoth et al. Citation2011). Those authors observed a clear seasonality pattern in the abundance of different zooplankton taxa. The present study also exhibited seasonal variations with the increasing trend during the rainy season, similar to the observed rise of phytoplankton density and biomass (Wagaw et al. Citation2021), which resulted in greater abundance and possibly quality of edible resources (Nandini et al. Citation2008; Arora and Mehra Citation2009; Joseph and Yamakanamardi Citation2011). Okoth et al. (Citation2011) and Gülle et al. (Citation2010) also observed the highest zooplankton abundance during periods of long rains in saline-alkaline lakes of Nakuru and Burdur.
The vertical distribution of zooplankton has been studied in various deep Lakes (e.g. Gülle et al. Citation2010; Degefu and Schagerl Citation2015). Usually, zooplankton shows distinct vertical distribution rather than being homogeneously distributed through the water column. During the present study, the vertical distribution of different zooplankton taxa was concentrated in various zones, nevertheless, the most concentrated region was between 0-15 m (p < 0.05). Most zooplankton density that exists in the upper 15 meters (euphotic layer) of Lake Shala may be due to the abundance of phytoplankton. Gutkowska et al. (Citation2018), discussed and indicated a significant direct correlation between phytoplankton and zooplankton population density in Vistula Lagoon and Lake Łebsko. According to this author, the phytoplankton abundance and biomass showed a pronounced significant occurrence in the euphotic zone than in the non-euphotic layer, which resulted in a dense zooplankton population. Moreover, Lake Shala’s surface water temperatures are tropical, and the minima continue to be high enough to support continued zooplankton production (Burian Citation2010; Okoth et al. Citation2011).
However, during persistent partial atelomictic mixing, zooplankton favor the mixing epilimnion (mixolinion), indicating that food conditions are ideal in this water column. This is likely because of frequent diurnal partial atelomictic mixing, which suspends food particles like phytoplankton, protozoans, and bacteria freely in the upper zone of the water column and permits a rapidly growing zooplankton population in Lake Shala, as occurs in other deep tropical lakes after atelomixis (Ciros‐Pérez et al. Citation2015; Degefu and Schagerl Citation2015). Rotifers and nauplii in particular may experience rapid population peak if food resource increase due to frequent mixing due to atelomixis and contributing for the unpredictable rises in population abundance (Ciros-Pérez et al. Citation2001). Ortega-Mayagoitia et al. (Citation2011) also verified that rotifers as opportunistic species and able to take advantage over increasing in food availability for their growth (especially Brachionus). Another important factors that explain variations in zooplankton abundance could be light-related tolerance factors, oxygen saturation, competition and predation (Williamson et al. Citation2020). According to Ciros‐Pérez et al. (Citation2015), copepods and cladocera migrated to a zone with very low levels of visible light in deep tropical oligotrophic lake.
The most notable occurrence of rotifers and nauplius was detected as the most dominant species in 0 - 10 m depth throughout the year and their density decreased with increasing depth (). These differences were significant for rotifers (F = 17.65, p = 0.00, ANOVA) and nauplius (F = 28.898, p = 0.00, ANOVA) between the various sampling depth. Rotifera attained its highest density year-round due to the flourishing of Brachionus species which can tolerate a high temperature, salinity, and electrical conductivity. Moreover, low phytoplankton biovolume, species composition, and anoxic conditions caused by a steady drop in temperature with depth are related to the decreased density of rotifers and nauplii with an increase in depth (Gülle et al. Citation2010). In this study, the phytoplankton composition and Chl a biomass in relation to depth were not analyzed, but a decline and variance distribution of phytoplankton and Chl a biomass occur in relation to depth (Żurek and Bucka Citation1994; Nandini et al. Citation2008; Voutilainen et al. Citation2016).This finding is concordant with Isumbisho et al. (Citation2006) in Lake Kivu and Gülle et al. (Citation2010) in Lake Burdu, which reported rotifers and nauplii larvae inhabited the surface layer (1-10) and the deeper by Copepods and Cladoceran.
In this study, the vertical distribution patterns of zooplankton were similar to that of electrical conductivity, temperature and dissolved oxygen curves ( and ). Copepods and Cladocerans are known to vertically migrate even in the lower oxygen condition (Dimante-Deimantovica et al. Citation2012; Voutilainen et al. Citation2016). In this study, the abundance of Copepods (F = 19.899, p = 0.00, ANOVA) and Cladocerans (F = 11.908, p = 0.00, ANOVA) were different among the sampling depth and the deepest region had the highest densities. This is supported by findings from various authors (Isumbisho et al. Citation2006; Gülle et al. Citation2010) who reported depth and electrical conductivity as a key factor governing changes in zooplankton abundance. Also, Copepods and Cladocerans are in the depths because of their inability to tolerate the light intensity on the surface compared to the depths (Gülle et al. Citation2010; Merhoon et al. Citation2017) and this can also explain the increase in the total density in the depths compared to the surface.
4.2. Driving factors of zooplankton abundance
By comparing the zooplankton community structure with environmental factors, the zooplankton community varied significantly as biotic and abiotic factors changed between different sampling stations. Chl a, phytoplankton abundance, pH, Alkalinity, and TP, reached the highest values at Shala Gike Station (SGS), where we find abundant B. dimidiatus, B. rubens, B. quadridentatus and T. oblongatus species. Temperature, NH3–N and SRP were also positively correlated with zooplankton abundance, which contributes to the high abundance of D. excisum T. ethiopiensis B. urceolaris, B. budapestinesis and B. plicatilis. Several earlier studies have also confirmed that abiotic and biotic factors such as temperature, nutrients (N and P), SiO2, pH, ionic concentrations and predation influence, directly or indirectly, the distribution and abundance of zooplankton species (Derry et al. Citation2003; Waya et al. Citation2014; Tavsanoglu et al. Citation2015).
The present study showed that the zooplankton community structure is generally influenced by EC and salinity, and most of the species were found against them. Gülle et al. (Citation2010) and Afonina and Tashlykova (Citation2018), also pointed electrical conductivity (salinity) as one of the several factors responsible for the structure of zooplankton communities in saline-alkaline lakes. Its impact can be strongly modified by other factors, and there are also indirect mechanisms by which it may exert its effects e.g. by imposing osmoregulatory stress, influence the availability of feed, or inducing migrations of organisms trying to evade high or low salinity and impact on the abundance of the zooplankton population (Perumal et al. Citation2009.; Afonina and Tashlykova Citation2018; Gutkowska et al. Citation2018).
4.3. Long-term trends of zooplankton changes in deep tropical Lake Shala
The zooplankton taxa encountered in Lake Shala during this study differed remarkably from those previously reported for this lake (Defaye Citation1988; Green and Mengestou Citation1991; Mengistou Citation2016), where between 1 and 9 zooplankton species were recorded. During the previous studies and Mengistou (Citation2016) in his review, of 12 identified species, 9 belonged to Rotifera; 2 Copepoda and 1 Cladocera species were reported. Partly similar results was observed in the present study; of 32 identified species, 23 belonged to Rotifera, 6 Copepoda and 3 Cladocera species was identified. The results of our study revealed that the composition of the species in zooplankton varied considerably, there was a significant increase in the number of species. Because the plankton mesh size net in this study (30 µm) differed from those of earlier studies (64 and 55 µm), the difference in sample collection methods, and inclusion of open water system and deep species, which might bring variation in the zooplankton community composition.
The zooplankton community structure of Lake Shala has also changed over time Tudorancea and Taylor (Citation2002) and Mengistou (Citation2016) reported benthic harpacticoids (Nitocra lacustris) as dominant species from Lakes Shala. However, in the current study, Nitocra lacustris were replaced by small-bodied and generalist-feeders rotifers (genus Brachionus) as the dominant taxa within all sampling sites and seasons. The notable absence of dominance of the benthic harpacticoids in Lake Shala might indicate the presence of ecological or major habitat shifts in the lake over the years. Also, the shift might be due to several reasons including the changes in the water chemistry of the lake (). Various studies in East African soda lakes revealed that plankton community structure changes in relation with water chemistry (Schagerl and Oduor Citation2008; Ogato and Kifle Citation2017). Zooplankton community shift may also associated with ‘atelomixis’ changes brought about by unusual mixing behavior in deep, tropical lakes (Fetahi et al. Citation2011; Degefu and Schagerl Citation2015) ().
Table 7. List and number of zooplankton taxa reported from Lake Shala.
5. Conclusion
In conclusion, euryhaline rotifers dominance in deep tropical lakes is observed, show variations in seasonal and vertical distribution: rotifers and nauplii concentrate in the epilimnion, while copepods and cladoceran are found predominantly in the deeper zone of the lake. Salinity and electrical conductivity remain the most critical factor influencing zooplankton distribution. A noticeable decrease in density and species richness of zooplankton was noticed at sites with high salinity and electrical conductivity. The community composition was highly changed with time series. Thirty-two taxa were recorded during the study, dominance copepod Nitocra lacustris were replaced by rotifers (genus Brachionus). This long-term succession of zooplankton may be associated with physicochemical changes and unusual mixing behavior (partial atelomixis) in deep tropical lakes. Also in future zooplankton studies in the lake, determination of diel vertical migration patterns and vertical distribution effects of the physicochemical variables, thermal stratification, phytoplankton abundance and chlorophyll a would contribute to further understanding of the deep tropical dynamics of the lake zooplankton.
Acknowledgements
We appreciate the financial support provided by Addis Ababa University through its Graduate Studies Program and Thematic Research Project. We thank Dr. Assefa Wosnie and Dr. Yirga Enawgaw for their help and advice in fieldwork and research techniques. Finally, we thank the Ethiopian Wildlife Conservation Authority (EWCA) and Abijatta-Shala Lakes National Park Administration for granting permission to conduct research in Lake Shala.
Disclosure statement
No potential conflict of interest was reported by the author(s).
Additional information
Funding
Notes on contributors
Solomon Wagaw
Dr. Solomon Wagaw graduated from Hawassa University with a first-class biology degree in 2009, and he also earned a master’s degree in limnology, fisheries, and aquatic ecotoxicology there in 2012. His Ph.D. in Aquatic Sciences, Fisheries, and Aquaculture was awarded by Addis Ababa University. He’s written eight publications that were peer-reviewed. Solomon is currently working as lecturer and researcher at Wolkite University.
Seyoum Mengistou
Professor Seyoum Mengistou received his Ph.D. from Waterloo University in Biology (Limnology). He has held a number of positions, including those of director of the zoological natural museum, head of the biology department at Addis Abeba University, editor-in-chief of the Ethiopian Journal of Biological Sciences, founding member of the Ethiopian Fisheries and Aquatic Sciences Association (EFASA), member of the Biological Society of Ethiopia, and member of the Society International of Limnology. Over 20 Ph.D. students have been mentored and supervised by him, and he has published over 95 publications in esteemed national and international scientific journals.
Abebe Getahun
Professor Abebe Getahun earned his BSc in Biology from Addis Abeba University as well as his MSc in Zoology. He then attended a combined program run by the American Museum of Natural History and the City University of New York, USA, to earn his MPhil and PhD in Ecology, Evolution, and Behaviour. He is working on research initiatives while also instructing undergraduate and graduate (MSc and PhD) students. The diversity, conservation, and use of aquatic organisms, particularly fish, are the main areas of his research. Over 90 articles in books and scholarly publications were written by him or with whom he collaborated. He has won numerous funding for study from various organizations. He has held a number of positions in academic administration at Addis Abeba University. He was a founder member of the Biological Society of Ethiopia (BSE) and the Ethiopian Fisheries and Aquatic Sciences Association (EFASA). He is a SINET Editor: Ethiopian Journal of Science and Ethiopian Journal of Biological Sciences.
References
- Afonina EY, Tashlykova NA. 2018. Plankton community and the relationship with the environment in saline lakes of Onon-Torey plain, Northeastern Mongolia. Saudi J Biol Sci. 25(2):399–408.
- Almaw R. 2012. A checklist of the birds of the Abijata-Shala Lakes National Park (central rift-valley, Ethiopia). Addis Abeba, Ethiopia: Ethiopian Wildlife Conservation Authority.
- Anton-Pardo M, Armengol X. 2012. Effects of salinity and water temporality on zooplankton community in coastal Mediterranean ponds. Estuarine Coastal Shelf Sci. 114:93–99.
- Arora J, Mehra NK. 2009. Seasonal dynamics of zooplankton in a shallow eutrophic, man-made hyposaline lake in Delhi (India): role of environmental factors. Hydrobiologia. 626(1):27–40.
- Ayenew T, Legesse D. 2007. The changing face of the Ethiopian rift lakes and their environs: call of the time. Lakes Reservoirs Res Manag. 12(3):149–165.
- Barbosa LG, Barbosa PMM, Barbosa FAR. 2011. Vertical distribution of phytoplankton functional groups in a tropical shallow lake: driving forces on a diel scale. Acta Limnol Brasiliensia. 23(1):63–73.
- Barbosa LG, Barbosa FAR, Bicudo CEM. 2013. Adaptive strategies of desmids in two tropical monomictic lakes in southeast Brazil: do morphometric differences promote life strategies selection? Hydrobiologia. 710(1):157–171.
- Baumann A, Förstner U, Rohde R. 1975. Lake Shala: water chemistry, mineralogy and geochemistry of sediments in an Ethiopian rift lake. Geol Rundsch. 64(1):593–609.
- Baxter RM. 2002. Lake morphology and chemistry. Ethiopian Rift Valley Lakes. Leiden: Backhuys; p. 45–60.
- Burian A. 2010. Zooplankton dynamics of two alkaline-saline lakes in the Keyan Rift Valley [(Doctoral dissertation, uniwien)].
- Ciros-Pérez J, Carmona MJ, Serra M. 2001. Resource competition between sympatric sibling rotifer species. Limnol Oceanogr. 46(6):1511–1523.
- Ciros-Pérez J, Ortega-Mayagoitia E, Alcocer J. 2015. The role of ecophysiological and behavioral traits in structuring the zooplankton assemblage in a deep, oligotrophic, tropical lake. Limnol. Oceanogr. 60(6):2158–2172.
- Dagne A, Herzig A, Jersabek CD, Tadesse Z. 2008. Abundance, species composition and spatial distribution of planktonic rotifers and crustaceans in Lake Ziway (Rift Valley, Ethiopia). Int Rev Hydrobiol. 93(2):210–226.
- Defaye D. 1988. Contribution à la connaissance des Crustacés Copépodes d‘Ethiopie. Hydrobiologia. 164(2):103–147.
- Degefu F, Schagerl M. 2015. Zooplankton abundance, species composition and ecology of tropical high-mountain crater Lake Wonchi, Ethiopia. J Limnol. 74(2):324–334.
- Derry AM, Prepas EE, Hebert PDN. 2003. A comparison of zooplankton communities in saline lake water with variable anion composition. Hydrobiologia. 505(1):199–215.
- Diego F, Yamila B, Gisela M, Patricia M. 2015. Controlling factors in planktonic communities over a salinity gradient in high-altitude lakes. Ann Limnol Int J Limnol. 51(3): 261–272. EDP Sciences.
- Dimante-Deimantovica I, Skute A, Skute R. 2012. Vertical variability of pelagic zooplankton fauna in deep Latvian lakes, with some notes on changes in ecological conditions. Estonian J Ecol. 61(4):247.
- Echaniz SA, Vignatti AM, De Paggi SJ, Paggi JC, Pilati A. 2006. Zooplankton Seasonal Abundance of South American Saline Shallow Lakes. Int Rev Hydrobiol. 91(1):86–100.
- Estifanos TK. 2008. Integrated Assessment of ecosystem services and stakeholder analysis of Abijata-Shala Lakes National Park, Ethiopia [Doctoral dissertation]. M Sc. Thesis in Environmental Sciences, Washington University.
- Fernandes V,Ramaiah N. 2019. Spatial structuring of zooplankton communities through partitioning of habitat and resources in the Bay of Bengal during spring intermonsoon. Turk. J. Zool. 43(1):68–93. doi:10.3906/zoo-1805-6.
- Fernández-Álamo MA, Färber-Lorda J. 2006. Zooplankton and the oceanography of the eastern tropical Pacific: a review. Prog Oceanogr. 69(2-4):318–359.
- Fernando CH. 2002. Zooplankton and tropical freshwater fisheries. A guide to tropical freshwater zooplankton. Identification, ecology, and impact on fisheries. Leiden (The Netherlands): Backhuys Publishers, 255–280.
- Fetahi T, Mengistou S, Schagerl M. 2011. Zooplankton community structure and ecology of the tropical-highland Lake Hayq, Ethiopia. Limnologica. 41(4):389–397.
- Golubtsov AS, Dgebuadze YY, Mina MV. 2002. Fishes of the Ethiopian rift valley. Ethiopian Rift Valley Lakes. 167:258.
- Green J, Mengestou S. 1991. Specific diversity and community structure of Rotifera in a salinity series of Ethiopian inland waters. Hydrobiologia. 209(2):95–106.
- Gülle I, Turna II, Güçlü SS, Gülle P, Güçlü Z. 2010. Zooplankton seasonal abundance and vertical distribution of highly alkaline Lake Burdur, Turkey. Turk J Fish Aquat Sci. 10(2):245–254.
- Gutkowska A, Paturej E, Koszalka J. 2018. Does the location of coastal brackish waters determine diversity and abundance of zooplankton assemblages? Turk J Zool. 42(2):230–244.
- Helenius L. 2015. The role of zooplankton in littoral communities: diversity and food web interactions in the Baltic Sea.
- Hötzel G, Croome R. 1999. A phytoplankton methods manual for Australian freshwaters.
- Hyslop EJ. 1980. Stomach contents analysis—a review of methods and their application. J Fish Biol. 17(4):411–429
- Isumbisho M, Sarmento H, Kaningini B, Micha JC, Descy JP. 2006. Zooplankton of Lake Kivu, East Africa, half a century after the Tanganyika sardine introduction. J Plankton Res. 28(11):971–989.
- Jones C, Somers KM, Craig B, Reynoldson TB. 2007. Ontario Benthos Biomonitoring Network: protocol manual. Dorset. pp: 455–461. Available from https://www.fs.usda.gov/rm/pubs/rmrs_p042/rmrs_p042_455_461.pdf
- Joseph B, Yamakanamardi SM. 2011. Monthly changes in the abundance and biomass of zooplankton and water quality parameters in Kukkarahalli Lake of Mysore, India. J Environ Biol. 32(5):551.
- Kebede E, Mariam ZG, Ahlgren I. 1994. The Ethiopian Rift Valley lakes: chemical characteristics of a salinity-alkalinity series. Hydrobiologia. 288(1):1–12.
- Korinek V. 1999. A guide to limnetic species of Cladocera of African inland Waters (Crustacea, Brachiopoda) (Using the morphology of parthenogenetic females). Volta Basin Research Project, Accra on behalf of International Association of Theoretical and Applied Limnology c/o Department of Biological Sciences, University of Alabama, Tuscaloosa, Alabama.
- Kruskal WH, Wallis WA. 1952. Use of ranks in one-criterion variance analysis. J Am Stat Assoc. 47(260):583–621.
- Le Turdu C, Tiercelin JJ, Gibert E, Travi Y, Lezzar KE, Richert JP, … Taieb M. 1999. The Ziway–Shala lake basin system, Main Ethiopian Rift: influence of volcanism, tectonics, and climatic forcing on basin formation and sedimentation. Palaeogeogr Palaeoclimatol Palaeoecol. 150(3-4):135–177.
- Mengistou S. 2016. Invertebrates of East African soda lakes. In Soda Lakes of East Africa. Cham: Springer; p. 205–226.
- Merhoon KA, Nashaat MR, Alkam FM. 2017. Environmental and vertical distribution study of zooplankton in Al-Diwaniyah River, Iraq. J Biodivers Environ Sci (JBES). 10(6):2017–2228.
- Nandini S, Merino-Ibarra M, Sarma SSS. 2008. Seasonal changes in the zooplankton abundances of the reservoir Valle de Bravo (State of Mexico, Mexico). Lake Reservoir Manag. 24(4):321–330.
- Oduor SO, Kotut K. 2016. Soda lakes of the East African Rift System: the past, the present and the future. In. Soda Lakes of East Africa. Cham: Springer; p. 365–374.
- Oduor SO, Schagerl M. 2007. Temporal trends of ion contents and nutrients in three Kenyan Rift Valley saline-alkaline lakes and their influence on phytoplankton biomass. In Shallow Lakes in a Changing World. Dordrecht: Springer; p. 59–68.
- Ogato T, Kifle D. 2017. Phytoplankton composition and biomass in tropical soda Lake Shala: seasonal changes in response to environmental drivers. Lakes Reservoirs Res Manag. 22(2):168–178.
- Ogato T. 2015. Dynamics of phytoplankton and physicochemical features of the Ethiopian soda lakes Chitu and Shala, and evaluation of the potential of their waters for the production of Arthrospira (Spirulina) fusiformis (Cyanophyceae) in laboratory cultures [Doctoral dissertation] Addis Ababa, Ethiopia: Addis Ababa University.
- Okoth E, Muchiri M, Ngugi CC, Njenga EW, Ngure V, Orina PS, Wanjohi BK. 2011. Zooplankton partitioning in a tropical alkaline–saline endorheic Lake Nakuru, Kenya: spatial and temporal trends in relation to the environment. Lakes Reserv Res Manag. 16(1):35–47.
- Ong’ondo GO, Yasindi AW, Oduor SO, Jost S, Schagerl M, Sonntag B, Boenigk J. 2013. Ecology and community structure of ciliated protists in two alkaline–saline Rift Valley lakes in Kenya with special emphasis on Frontonia. J Plankton Res. 35(4):759–771.
- Ortega-Mayagoitia E, Ciros-Pérez J, Sánchez-Martínez M. 2011. A story of famine in the pelagic realm: temporal and spatial patterns of food limitation in rotifers from an oligotrophic tropical lake. J Plankton Res. 33(10):1574–1585.
- Perumal NV, Rajkumar M, Perumal P, Rajasekar KT. 2009. Seasonal variations of plankton diversity in the Kaduviyar estuary, Nagapattinam, southeast coast of India. J Environ. Biol. 30(6):1035–1046.
- Savitha N, Yamakanamardi SM. 2012. Studies on abundance of zooplanktons in lakes of Mysore, India. J Environ Biol. 33(6):1079.
- Schagerl M, Burian A, Gruber-Dorninger M, Oduor SO, Kaggwa MN. 2015. Algal communities of Kenyan soda lakes with a special focus on Arthrospira fusiformis. Fottea. 15(2):245–257.
- Schagerl M, Oduor SO. 2008. Phytoplankton community relationship to environmental variables in three Kenyan Rift Valley saline-alkaline lakes. Mar Freshwater Res. 59(2):125–136.
- Souza MBG, Barros CF, Barbosa F, Hajnal E, Padisák J. 2008. Role of atelomixis in replacement of phytoplankton assemblages in Dom Helvécio Lake, South-East Brazil. Hydrobiologia. 607(1):211–224.
- Stephenson JG. 1978. An appraisal of the conservation of nature in the lakes Abijiata and Shala locality with recommendations. Ethiopia: EWCO Addis Ababa
- Talling JF, Driver D. 1963. Some problems in the estimation of chlorophyll-a in phytoplankton, in primary productivity measurement, marine and freshwater. US Atomic Energy Commision Report No. TID-7633; Doty, MS, Ed, 142–146.
- Talling J, Talling IB. 1965. The chemical composition of African lake waters. Int Revue Gesamten Hydrobiol Hydrographie. 50(3):421–463.
- Tavera R, Martı’nez-Almeida V. 2005. Atelomixis as a possible driving force in the phytoplankton composition of Zirahuén, a warm-monomictic tropical lake. Hydrobiologia. 533:199–208.
- Tavsanoglu UN, Maleki R, Akbulut N. 2015. Effects of salinity on the zooplankton community structure in two maar lakes and one freshwater lake in the Konya closed basin, Turkey. Ekoloji. 24(94):25–32.
- Ter Braak CJ, Smilauer P. 2002. CANOCO reference manual and CanoDraw for Windows user’s guide: software for canonical community ordination (version 4.5). www.canoco.com.
- Tudorancea C, Harrison AD. 1988. The benthic communities of the saline lakes Abijata and Shala (Ethiopia). In Saline Lakes. Dordrecht: Springer; p. 117–123.
- Tudorancea C, Taylor WD. (Eds.) 2002. Ethiopian rift valley lakes. Leiden: Backhuys Publishers, Netherlands, 303 pp.
- Von Damm KL, Edmond JM. 1984. Reverse weathering in the closed-basin lakes of the Ethiopian Rift. Am J Sci. 284(7):835–862.
- Voutilainen A, Jurvelius J, Lilja J, Viljanen M, Rahkola-Sorsa M. 2016. Associating spatial patterns of zooplankton abundance with water temperature, depth, planktivorous fish and chlorophyll.
- Wagaw S, Mengistou S, Getahun A. 2021. Phytoplankton community structure in relation to physico-chemical factors in a tropical soda lake, Lake Shala (Ethiopia). Afr J Aquat Sci. 46(4):428–440.
- Waya RK, Limbu SM, Ngupula GW, Mwita CJ, Mgaya YD. 2014. Spatial patterns of zooplankton distribution and abundance in relation to phytoplankton, fish catch and some water quality parameters at Shirati Bay, Lake Victoria, Tanzania. Tanzania J Sci. 40(1):20–32.
- Wetzel RG, Likens GE. 2000. Composition and biomass of phytoplankton. Limnol Anal. 147–174.
- Williamson CE, Overholt EP, Pilla RM, Wilkins KW. 2020. Habitat-mediated responses of zooplankton to decreasing light in two temperate lakes undergoing long-term browning. Front Environ Sci. 8:1–14.
- Wodajo K, Belay A. 1984. Species composition and seasonal abundance of zooplankton in two Ethiopian Rift Valley lakes—Lakes Abiata and Langano. In Tropical Zooplankton. Dordrecht: Springer; p. 129–136.
- Wood RB, Talling JF. 1988. Chemical and algal relationships in a salinity series of Ethiopian inland waters. In Saline Lakes. Dordrecht: Springer; p. 29–67.
- Yin XW, Tan BB, Zhou YC, Li XC, Liu W. 2016. Development time of male and female rotifers with sexual size dimorphism. Hydrobiologia. 767(1):27–35.
- Yongo E, Outa N. 2017. Spatial distribution and abundance of zooplankton communities in Lake Victoria, Kenya. Int J Fish Aquat Res. 2(1):33–35.
- Zinabu GM. 2002. The Ethiopian Rift Valley lakes: major threats and strategies for conservation. In Ethiopian Rift Valley Lakes. Leiden: Backhuys; p. 259–271.
- Zinabu GM, Kebede-Westhead E, Desta Z. 2002. Long-term changes in chemical features of waters of seven Ethiopian rift-valley lakes. Hydrobiologia. 477(1):81–91.
- Żurek R, Bucka H. 1994. Algal size classes and phytoplankton-zooplankton interacting effects. J Plankton Res. 16(6):583–601.